User login
Rapid Response Systems
In 2006,[1] we questioned whether rapid response systems (RRSs) were an effective strategy for detecting and managing deteriorating general ward patients. Since then, the implementation of RRSs has flourished, especially in the United States where accreditors (Joint Commission)[2] and patient‐safety organizations (Institute for Healthcare Improvement 100,000 Live Campaign)[3] have strongly supported RRSs. Decades of evidence show that general ward patients often experience unrecognized deterioration and cardiorespiratory arrest (CA). The low sensitivity and accuracy of periodic assessments by staff are thought to be a major reason for these lapses, as are imbalances between patient needs and clinician (primarily nursing) resources. Additionally, a medical culture that punishes speaking up or bypassing the chain of command are also likely contributors to the problem. A system that effectively recognizes the early signs of deterioration and quickly responds should catch problems before they become life threatening. Over the last decade, RRSs have been the primary intervention implemented to do this. The potential for RRSs to improve outcomes has strong face validity, but researchers have struggled to demonstrate consistent improvements in outcomes across institutions. Given this, are RRSs the best intervention to prevent this failure to rescue? In this editorial we examine the progress of RRSs, how they compare to other options, and we consider whether we should continue to question their implementation.
In our 2007 systematic review,[4] we concluded there was weak to moderate evidence supporting RRSs. Since then, 6 other systematic reviews of the effectiveness or implementation of RRSs have been published. One high‐quality review of effectiveness studies published through 2008 by Chan et al.[5] found that RRSs significantly reduced non‐intensive care unit (ICU) CA (relative risk [RR], 0.66; 95% confidence interval [CI], 0.54‐0.80), but not total hospital mortality (RR, 0.96; 95% CI, 0.84‐1.09) in adult inpatients. In pediatric inpatients, RRSs led to significant improvements in both non‐ICU CA (RR, 0.62; 95% CI, 0.46 to 0.84) and total hospital mortality (RR, 0.79; 95% CI, 0.63 to 0.98). Subsequent to 2008, a structured search[6] finds 26 additional studies.[7, 8, 9, 10, 11, 12, 13, 14, 15, 16, 17, 18, 19, 20, 21, 22, 23, 24, 25, 26, 27, 28, 29, 30] Although the benefit for CA in both adults and children has remained robust, even more so since Chan's review, mortality reductions in adult patients appear to have had the most notable shift. In aggregate, the point estimate (for those studies providing analyzable data), for adult mortality has strengthened to 0.88, with a confidence interval of 0.82‐0.96 in favor of the RRS strategy.
This change has occurred as the analyzable studies since 2008 have all had favorable point estimates, and 4 have had statistically significant confidence intervals. Prior to 2008, 5 had unfavorable point estimates, and only 2 had favorable confidence intervals. As RRSs expand, the benefits, although not universal (some hospitals still experience no improvement in outcomes), seem to be getting stronger and more consistent. This may be secondary to maturation of the intervention and implementation strategies, or it may be the result of secular trends outside of the RRS intervention, although studies controlling for this found it not to be the case.[10] The factors associated with successful implementation of the RRS or improved outcomes include knowledge of activation criteria, communication, teamwork, lack of criticism for activating the RRS, and better attitudes about the team's positive effect on nurses and patients. Many of these factors relate to an improved safety culture in general. Additionally, activation rates may have increased in more recent studies, as greater utilization is associated with improved outcomes.[31] Finally, RRSs, like other patient‐safety and quality interventions, mature with time, often taking several years before they have a full effect on outcomes.[31, 32]
Despite these more favorable results for RRSs, we still see a large discrepancy between the magnitude of benefit for CA and mortality. This may partly be because the exposure groups are different; most studies examined non‐ICU CA, yet studies reporting mortality used total hospital mortality (ICU and non‐ICU). Additionally, although RRSs may effectively prevent CA, this intervention may have a more limited effect in preventing the patient's ultimate demise (particularly in the ICU).
We also still see that effectiveness reports for RRSs continue to be of low to moderate quality. Many reports give no statistics or denominator data or have missing data. Few control for secular trends in providers, outcomes, and confounders. Outcome measures vary widely, and none conducted blinded outcome assessments. Most studies use a pre‐post design without concurrent controls, substantially increasing the risk of bias. The better‐designed studies that use concurrent controls or cluster randomization (Priestley,[33] Bristow,[34] and the MERIT trial[35]) tend to show lower treatment effects, although interestingly in the MERIT trial, while the cluster‐randomized data showed no benefit, the pre‐post data showed significant improvement in the RRS intervention hospitals. These results have been attributed to the control hospitals using their code teams for RRS activities,[36] negating a comparative improvement in the intervention hospitals.
Can we improve RRS research? Likely, yes. We can begin by being more careful about defining the exposure group. Ideally, studies should not include data from the ICU or the emergency department because these patient populations are not part of the exposure group. Although most studies removed ICU and emergency department data for CA, they did not do so for hospital mortality. ICU mortality is likely biased, because only a small proportion of ICU patients have been exposed to an RRS. Definitions also need to be stringent and uniform. For example, CA may be defined in a variety of ways such as calling the code team versus documented cardiopulmonary resuscitation. Unexpected hospital mortality is often defined as excluding patients with do not resuscitate (DNR) orders, but this may or may not accurately exclude expected deaths. We also need to better attempt to control for confounders and secular trends. Outcomes such as CA and mortality are strongly influenced by changes in patient case‐mix over time, the frequency of care limitation/DNR orders, or by poor triage decisions.[37] Outcomes such as unanticipated ICU admission are indirect and may be heavily influenced by local cultural factors. Finally, authors need to provide robust statistical data and clear numerators and denominators to support their conclusions.
Although we need to do our best to improve the quality of the RRS literature, the near ubiquitous presence of this patient‐safety intervention in North American hospitals raises a crucial question, Do we even need more effectiveness studies and if so what kind? Randomized controlled trials are not likely. It is hard to argue that we still sit at a position of equipoise, and randomizing patients who are deteriorating to standard care versus an RRS is neither practical nor ethical. Finding appropriate concurrent control hospitals that have not implemented some type of RRS would also be very difficult.
We should, however, continue to test the effectiveness of RRSs but in a more diverse manner. RRSs should be more directly compared to other interventions that can improve the problem of failure to rescue such as increased nurse staffing[38, 39, 40] and hospitalist staffing.[41] The low sensitivity and accuracy of monitoring vital signs on general wards by staff is also an area strongly deserving of investigation, as it is likely central to the problem. Researchers have sought to use various combinations of vital signs, including aggregated or weighted scoring systems, and recent data suggest some approaches may be superior to others.[42] Many have advocated for continuous monitoring of a limited set of vital signs similar to the ICU, and there are some recent data indicating that this might be effective.[43, 44] This work is in the early stages, and we do not yet know whether this strategy will affect outcomes. It is conceivable that if the false alarm rate can be kept very low and we can minimize the failure to recognize deteriorating patients (good sensitivity, specificity, and positive predictive value), the need for the RRS response team may be reduced or even eliminated. Additionally, as electronic medical records (EMRs) have expanded, there has been growing interest in leveraging these systems to improve the effectiveness of RRSs.[45] There is a tremendous amount of information within the EMRs that can be used to complement vital‐sign monitoring (manual or continuous), because baseline medical problems, laboratory values, and recent history may have a strong impact on the predictive value of changes in vital signs.
Research should also focus on the possible unintended consequences, costs, and the cost‐effectiveness of RRSs compared with other interventions that can or may reduce the rate of failure to rescue. Certainly, establishing RRSs has costs including staff time and the need to pull staff from other clinical duties to respond. Unintended harm, such as diversion of ICU staff from their usual care, are often mentioned but never rigorously evaluated. Increasing nurse staffing has very substantial costs, but how these costs compare to the costs of the RRS are unclear, although likely the comparison would be very favorable to the RRS, because staffing typically relies on existing employees with expertise in caring for the critically ill as opposed to workforce expansion. Given the current healthcare economic climate, any model that relies on additional employees is not likely to gain support. Establishing continuous monitoring systems have up‐front capital costs, although they may reduce other costs in the long run (eg, staff, medical liability). They also have intangible costs for provider workload if the false alarm rates are too high. Again, this strategy is too new to know the answers to these concerns. As we move forward, such evaluations are needed to guide policy decisions.
We also need more evaluation of RRS implementation science. The optimal way to organize, train, and staff RRSs is unknown. Most programs use physician‐led teams, although some use nurse‐led teams. Few studies have compared the various models, although 1 study that compared a resident‐led to an attending‐led team found no difference.[17] Education is ubiquitous, although actual staff training (simulation for example) is not commonly described. In addition, there is wide variation in the frequency of RRS activation. We know nurses and residents often feel pressured not to activate RRSs, and much of the success of the RRS relies on nurses identifying deteriorating patients and calling the response team. The use of continuous monitoring combined with automatic notification of staff may reduce the barriers to activating RRSs, increasing activation rates, but until then we need more understanding of how to break down these barriers. Family/patient access to activation has also gained ground (1 program demonstrated outcome improvement only after this was established[13]), but is not yet widespread.
The role of the RRS in improving processes of care, such as the appropriate institution of DNR orders, end of life/palliative care discussions, and early goal‐directed therapy for sepsis, have been presented in several studies[46, 47] but remain inadequately evaluated. Here too, there is much to learn about how we might realize the full effectiveness of this patient‐safety strategy beyond outcomes such as CA and hospital mortality. Ideally, if all appropriate patients had DNR orders and we stopped failing to recognize and respond to deteriorating ward patients, CAs on general hospital wards could be nearly eliminated.
RRSs have been described as a band‐aid for a failed model of general ward care.[37] What is clear is that many patients suffer preventable harm from unrecognized deterioration. This needs to be challenged, but are RRSs the best intervention? Despite the Joint Commission's Patient Safety Goal 16, should we still question their implementation? Should we (and the Joint Commission) reconsider our approach and prioritize our efforts elsewhere or should we feel comfortable with the investment that we have made in these systems? Even though there are many unknowns, and the quality of RRS studies needs improvement, the literature is accumulating that RRSs do reduce non‐ICU CA and improve hospital mortality. Without direct comparison studies demonstrating superiority of other expensive strategies, there is little reason to reconsider the RRS concept or question their implementation and our investment. We should instead invest further in this foundational patient‐safety strategy to make it as effective as it can be.
Disclosures: Dr. Pronovost reports the following potential conflicts of interest: grant or contract support from the Agency for Healthcare Research and Quality, and the Gordon and Betty Moore Foundation (research related to patient safety and quality of care), and the National Institutes of Health (acute lung injury research); consulting fees from the Association of Professionals in Infection Control and Epidemiology, Inc.; honoraria from various hospitals, health systems, and the Leigh Bureau to speak on quality and patient safety; book royalties from the Penguin Group; and board membership for the Cantel Medical Group. Dr. Winters reports the following potential conflicts of interest: contract or grant support from Masimo Corporation, honoraria from 3M Corporation and various hospitals and health systems, royalties from Lippincott Williams &Wilkins (UptoDate), and consulting fees from several legal firms for medical legal consulting.
- Rapid response teams: walk, don't run. JAMA. 2006;296:1645–1647. , , .
- Joint Commission requirement: The Joint Commission announces the 2008 National Patient Safety Goals and Requirements. Jt Comm Perspect. 2007;27(7):1–22.
- Institute for Healthcare Improvement. 5 million lives campaign: overview. Available at: http://www.ihi.org/offerings/Initiatives/PastStrategicInitiatives/5MillionLivesCampaign/Pages/default.aspx. Accessed November 28, 2012.
- Rapid response systems: a systematic review. Crit Care Med. 2007;35:1238–1243. , , , , , .
- Rapid response teams: a systematic review and meta‐analysis. Arch Intern Med. 2010;170:18–26. , , , , .
- Rapid‐response systems as a patient safety strategy: a systematic review. Ann Intern Med. 2013;158:417–425. , , , , , .
- Hospital‐wide code rates and mortality before and after implementation of a rapid response team. JAMA. 2008;300:2506–2513. , , , , , .
- Experience of pediatric rapid response team in a tertiary care hospital in Pakistan. Indian J Pediatr. 2010;77:273–276. , , , .
- Rescue me: saving the vulnerable non‐ICU patient population. Jt Comm J Qual Patient Saf. 2009;35:199–205. , , , et al.
- Reduction in hospital‐wide mortality after implementation of a rapid response team: a long‐term cohort study. Crit Care. 2011;15:R269. , , , , .
- Using an advanced practice nursing model for a rapid response team. Jt Comm J Qual Patient Saf. 2008;34:743–747. , , , , .
- Immediate and long‐term impact of medical emergency teams on cardiac arrest prevalence and mortality: a plea for periodic basic life‐support training programs. Crit Care Med. 2009;37:3054–3061. , , , , , .
- Successful implementation of a family and patient activated rapid response team in an adult level 1 trauma center. Resuscitation. 2010;81:1676–1681. , , , , , .
- A reduction in cardiac arrests and duration of clinical instability after implementation of a paediatric rapid response system. Qual Saf Health Care. 2009;18:500–504. , , , et al.
- Implementing a rapid response team to decrease emergencies outside the ICU: one hospital's experience. Medsurg Nurs. 2009;18:84–90, 126. , , , et al.
- Sustained effectiveness of a primary‐team‐based rapid response system. Crit Care Med. 2012;40:2562–2568. , , , et al.
- Association between implementation of an intensivist‐led medical emergency team and mortality. BMJ Qual Saf. 2012;21:152–159. , , , .
- Reducing in‐hospital cardiac arrests and hospital mortality by introducing a medical emergency team. Intensive Care Med. 2010;36:100–106. , , , , , .
- Implementation of a multicenter rapid response system in pediatric academic hospitals is effective. Pediatrics. 2011;128:72–78. , , , et al.
- The impact of medical emergency teams on ICU admission rates, cardiopulmonary arrests and mortality in a regional hospital. Resuscitation. 2011;82:707–712. , .
- Introduction of a rapid response system at a United States veterans affairs hospital reduced cardiac arrests. Anesth Analg. 2010;111:679–686. , , , .
- The effect of the medical emergency team on unexpected cardiac arrest and death at the VA Caribbean healthcare system: a retrospective study. Crit Care Shock. 2010;13:98–105. , , , .
- Four years' experience with a hospitalist‐led medical emergency team: an interrupted time series. J Hosp Med. 2012;7:98–103. , , , , .
- Changing cardiac arrest and hospital mortality rates through a medical emergency team takes time and constant review. Crit Care Med. 2010;38:445–450. , , .
- Clinical emergencies and outcomes in patients admitted to a surgical versus medical service. Resuscitation. 2011;82:415–418. , , , et al.
- Evaluating a new rapid response team: NP‐led versus intensivist‐led comparisons. AACN Adv Crit Care. 2012;23:32–42. , , , .
- Implementation of a rapid response team: a success story. Crit Care Nurse. 2009;29:66–75. , .
- Rapid response team in an academic institution: does it make a difference? Chest. 2011;139:1361–1367. , , , .
- Reduction of hospital mortality and of preventable cardiac arrest and death on introduction of a pediatric medical emergency team. Ped Crit Care Med. 2009;10:306–312. , .
- Medical emergency teams are associated with reduced mortality across a major metropolitan health network after two years service: a retrospective study using government administrative data. Crit Care. 2012;16:R210. , .
- Long term effect of a medical emergency team on cardiac arrests in a teaching hospital. Crit Care. 2005;9:R808–R815. , , , , et al.
- Six year audit of cardiac arrests and medical emergency team calls in an Australian outer metropolitan teaching hospital. BMJ. 2007;335:1210–1212. , , , .
- Introducing Critical Care Outreach: a ward‐randomised trial of phased introduction in a general hospital. Intensive Care Med. 2004;30:1398–1404. , , , et al.
- Rates of in‐hospital arrests, deaths, and intensive care admissions: the effect of a medical emergency team. Med J Aust. 2000;173:236–240. , , , et al.
- Introduction of the medical emergency team (MET) system: a cluster randomised controlled trial. Lancet. 2005;365:2091–2097. , , , et al.
- The effectiveness of implementation of the medical emergency team (MET) system and factors associated with use during the MERIT study. Crit Care Resusc. 2007;9:206–212. , , , , , .
- Rethinking rapid response teams. JAMA. 2010;304:1375–1376. , .
- Lower mortality for abdominal aortic aneurysm repair in high‐volume hospitals is contingent upon nurse staffing [published online ahead of print October 22, 2012]. Health Serv Res. doi: 10.1111/1475–6773.12004. , , .
- Nurse‐staffing levels and the quality of care in hospitals. N Engl J Med. 2002;346:1715–1722. , , , , .
- The association of registered nurse staffing levels and patient outcomes: systematic review and meta‐analysis. Med Care. 2007;45:1195–1204. .
- Outcomes of care by hospitalists, general internists, and family physicians. N Engl J Med. 2007;357:2589–2600. , , , , , .
- The ability of the National Early Warning Score (NEWS) to discriminate patients at risk of early cardiac arrest, unanticipated intensive care unit admission, and death. Resuscitation. 2013;84:465–470. , , , , .
- Impact of pulse oximetry surveillance on rescue events and intensive care unit transfers: a before‐and‐after concurrence study. Anesthesiology. 2010;112:282–287. , , , .
- A controlled trial of electronic automated advisory vital signs monitoring in general hospital wards. Crit Care Med. 2012;40:2349–2361. , , , et al.
- Agency for Healthcare Research and Quality. Early warning scoring system proactively identifies patients at risk of deterioration, leading to fewer cardiopulmonary emergencies and deaths. Available at: http://www.innovations.ahrq.gov/content.aspx?id=2607. Accessed March 26, 2013.
- Effect of a rapid response system for patients in shock on time to treatment and mortality during 5 years. Crit Care Med. 2007;35:2568–2575. , , , et al.
- The medical emergency team and end‐of‐life care: a pilot study. Crit Care Resusc. 2007;9:151–156. , , , , , .
In 2006,[1] we questioned whether rapid response systems (RRSs) were an effective strategy for detecting and managing deteriorating general ward patients. Since then, the implementation of RRSs has flourished, especially in the United States where accreditors (Joint Commission)[2] and patient‐safety organizations (Institute for Healthcare Improvement 100,000 Live Campaign)[3] have strongly supported RRSs. Decades of evidence show that general ward patients often experience unrecognized deterioration and cardiorespiratory arrest (CA). The low sensitivity and accuracy of periodic assessments by staff are thought to be a major reason for these lapses, as are imbalances between patient needs and clinician (primarily nursing) resources. Additionally, a medical culture that punishes speaking up or bypassing the chain of command are also likely contributors to the problem. A system that effectively recognizes the early signs of deterioration and quickly responds should catch problems before they become life threatening. Over the last decade, RRSs have been the primary intervention implemented to do this. The potential for RRSs to improve outcomes has strong face validity, but researchers have struggled to demonstrate consistent improvements in outcomes across institutions. Given this, are RRSs the best intervention to prevent this failure to rescue? In this editorial we examine the progress of RRSs, how they compare to other options, and we consider whether we should continue to question their implementation.
In our 2007 systematic review,[4] we concluded there was weak to moderate evidence supporting RRSs. Since then, 6 other systematic reviews of the effectiveness or implementation of RRSs have been published. One high‐quality review of effectiveness studies published through 2008 by Chan et al.[5] found that RRSs significantly reduced non‐intensive care unit (ICU) CA (relative risk [RR], 0.66; 95% confidence interval [CI], 0.54‐0.80), but not total hospital mortality (RR, 0.96; 95% CI, 0.84‐1.09) in adult inpatients. In pediatric inpatients, RRSs led to significant improvements in both non‐ICU CA (RR, 0.62; 95% CI, 0.46 to 0.84) and total hospital mortality (RR, 0.79; 95% CI, 0.63 to 0.98). Subsequent to 2008, a structured search[6] finds 26 additional studies.[7, 8, 9, 10, 11, 12, 13, 14, 15, 16, 17, 18, 19, 20, 21, 22, 23, 24, 25, 26, 27, 28, 29, 30] Although the benefit for CA in both adults and children has remained robust, even more so since Chan's review, mortality reductions in adult patients appear to have had the most notable shift. In aggregate, the point estimate (for those studies providing analyzable data), for adult mortality has strengthened to 0.88, with a confidence interval of 0.82‐0.96 in favor of the RRS strategy.
This change has occurred as the analyzable studies since 2008 have all had favorable point estimates, and 4 have had statistically significant confidence intervals. Prior to 2008, 5 had unfavorable point estimates, and only 2 had favorable confidence intervals. As RRSs expand, the benefits, although not universal (some hospitals still experience no improvement in outcomes), seem to be getting stronger and more consistent. This may be secondary to maturation of the intervention and implementation strategies, or it may be the result of secular trends outside of the RRS intervention, although studies controlling for this found it not to be the case.[10] The factors associated with successful implementation of the RRS or improved outcomes include knowledge of activation criteria, communication, teamwork, lack of criticism for activating the RRS, and better attitudes about the team's positive effect on nurses and patients. Many of these factors relate to an improved safety culture in general. Additionally, activation rates may have increased in more recent studies, as greater utilization is associated with improved outcomes.[31] Finally, RRSs, like other patient‐safety and quality interventions, mature with time, often taking several years before they have a full effect on outcomes.[31, 32]
Despite these more favorable results for RRSs, we still see a large discrepancy between the magnitude of benefit for CA and mortality. This may partly be because the exposure groups are different; most studies examined non‐ICU CA, yet studies reporting mortality used total hospital mortality (ICU and non‐ICU). Additionally, although RRSs may effectively prevent CA, this intervention may have a more limited effect in preventing the patient's ultimate demise (particularly in the ICU).
We also still see that effectiveness reports for RRSs continue to be of low to moderate quality. Many reports give no statistics or denominator data or have missing data. Few control for secular trends in providers, outcomes, and confounders. Outcome measures vary widely, and none conducted blinded outcome assessments. Most studies use a pre‐post design without concurrent controls, substantially increasing the risk of bias. The better‐designed studies that use concurrent controls or cluster randomization (Priestley,[33] Bristow,[34] and the MERIT trial[35]) tend to show lower treatment effects, although interestingly in the MERIT trial, while the cluster‐randomized data showed no benefit, the pre‐post data showed significant improvement in the RRS intervention hospitals. These results have been attributed to the control hospitals using their code teams for RRS activities,[36] negating a comparative improvement in the intervention hospitals.
Can we improve RRS research? Likely, yes. We can begin by being more careful about defining the exposure group. Ideally, studies should not include data from the ICU or the emergency department because these patient populations are not part of the exposure group. Although most studies removed ICU and emergency department data for CA, they did not do so for hospital mortality. ICU mortality is likely biased, because only a small proportion of ICU patients have been exposed to an RRS. Definitions also need to be stringent and uniform. For example, CA may be defined in a variety of ways such as calling the code team versus documented cardiopulmonary resuscitation. Unexpected hospital mortality is often defined as excluding patients with do not resuscitate (DNR) orders, but this may or may not accurately exclude expected deaths. We also need to better attempt to control for confounders and secular trends. Outcomes such as CA and mortality are strongly influenced by changes in patient case‐mix over time, the frequency of care limitation/DNR orders, or by poor triage decisions.[37] Outcomes such as unanticipated ICU admission are indirect and may be heavily influenced by local cultural factors. Finally, authors need to provide robust statistical data and clear numerators and denominators to support their conclusions.
Although we need to do our best to improve the quality of the RRS literature, the near ubiquitous presence of this patient‐safety intervention in North American hospitals raises a crucial question, Do we even need more effectiveness studies and if so what kind? Randomized controlled trials are not likely. It is hard to argue that we still sit at a position of equipoise, and randomizing patients who are deteriorating to standard care versus an RRS is neither practical nor ethical. Finding appropriate concurrent control hospitals that have not implemented some type of RRS would also be very difficult.
We should, however, continue to test the effectiveness of RRSs but in a more diverse manner. RRSs should be more directly compared to other interventions that can improve the problem of failure to rescue such as increased nurse staffing[38, 39, 40] and hospitalist staffing.[41] The low sensitivity and accuracy of monitoring vital signs on general wards by staff is also an area strongly deserving of investigation, as it is likely central to the problem. Researchers have sought to use various combinations of vital signs, including aggregated or weighted scoring systems, and recent data suggest some approaches may be superior to others.[42] Many have advocated for continuous monitoring of a limited set of vital signs similar to the ICU, and there are some recent data indicating that this might be effective.[43, 44] This work is in the early stages, and we do not yet know whether this strategy will affect outcomes. It is conceivable that if the false alarm rate can be kept very low and we can minimize the failure to recognize deteriorating patients (good sensitivity, specificity, and positive predictive value), the need for the RRS response team may be reduced or even eliminated. Additionally, as electronic medical records (EMRs) have expanded, there has been growing interest in leveraging these systems to improve the effectiveness of RRSs.[45] There is a tremendous amount of information within the EMRs that can be used to complement vital‐sign monitoring (manual or continuous), because baseline medical problems, laboratory values, and recent history may have a strong impact on the predictive value of changes in vital signs.
Research should also focus on the possible unintended consequences, costs, and the cost‐effectiveness of RRSs compared with other interventions that can or may reduce the rate of failure to rescue. Certainly, establishing RRSs has costs including staff time and the need to pull staff from other clinical duties to respond. Unintended harm, such as diversion of ICU staff from their usual care, are often mentioned but never rigorously evaluated. Increasing nurse staffing has very substantial costs, but how these costs compare to the costs of the RRS are unclear, although likely the comparison would be very favorable to the RRS, because staffing typically relies on existing employees with expertise in caring for the critically ill as opposed to workforce expansion. Given the current healthcare economic climate, any model that relies on additional employees is not likely to gain support. Establishing continuous monitoring systems have up‐front capital costs, although they may reduce other costs in the long run (eg, staff, medical liability). They also have intangible costs for provider workload if the false alarm rates are too high. Again, this strategy is too new to know the answers to these concerns. As we move forward, such evaluations are needed to guide policy decisions.
We also need more evaluation of RRS implementation science. The optimal way to organize, train, and staff RRSs is unknown. Most programs use physician‐led teams, although some use nurse‐led teams. Few studies have compared the various models, although 1 study that compared a resident‐led to an attending‐led team found no difference.[17] Education is ubiquitous, although actual staff training (simulation for example) is not commonly described. In addition, there is wide variation in the frequency of RRS activation. We know nurses and residents often feel pressured not to activate RRSs, and much of the success of the RRS relies on nurses identifying deteriorating patients and calling the response team. The use of continuous monitoring combined with automatic notification of staff may reduce the barriers to activating RRSs, increasing activation rates, but until then we need more understanding of how to break down these barriers. Family/patient access to activation has also gained ground (1 program demonstrated outcome improvement only after this was established[13]), but is not yet widespread.
The role of the RRS in improving processes of care, such as the appropriate institution of DNR orders, end of life/palliative care discussions, and early goal‐directed therapy for sepsis, have been presented in several studies[46, 47] but remain inadequately evaluated. Here too, there is much to learn about how we might realize the full effectiveness of this patient‐safety strategy beyond outcomes such as CA and hospital mortality. Ideally, if all appropriate patients had DNR orders and we stopped failing to recognize and respond to deteriorating ward patients, CAs on general hospital wards could be nearly eliminated.
RRSs have been described as a band‐aid for a failed model of general ward care.[37] What is clear is that many patients suffer preventable harm from unrecognized deterioration. This needs to be challenged, but are RRSs the best intervention? Despite the Joint Commission's Patient Safety Goal 16, should we still question their implementation? Should we (and the Joint Commission) reconsider our approach and prioritize our efforts elsewhere or should we feel comfortable with the investment that we have made in these systems? Even though there are many unknowns, and the quality of RRS studies needs improvement, the literature is accumulating that RRSs do reduce non‐ICU CA and improve hospital mortality. Without direct comparison studies demonstrating superiority of other expensive strategies, there is little reason to reconsider the RRS concept or question their implementation and our investment. We should instead invest further in this foundational patient‐safety strategy to make it as effective as it can be.
Disclosures: Dr. Pronovost reports the following potential conflicts of interest: grant or contract support from the Agency for Healthcare Research and Quality, and the Gordon and Betty Moore Foundation (research related to patient safety and quality of care), and the National Institutes of Health (acute lung injury research); consulting fees from the Association of Professionals in Infection Control and Epidemiology, Inc.; honoraria from various hospitals, health systems, and the Leigh Bureau to speak on quality and patient safety; book royalties from the Penguin Group; and board membership for the Cantel Medical Group. Dr. Winters reports the following potential conflicts of interest: contract or grant support from Masimo Corporation, honoraria from 3M Corporation and various hospitals and health systems, royalties from Lippincott Williams &Wilkins (UptoDate), and consulting fees from several legal firms for medical legal consulting.
In 2006,[1] we questioned whether rapid response systems (RRSs) were an effective strategy for detecting and managing deteriorating general ward patients. Since then, the implementation of RRSs has flourished, especially in the United States where accreditors (Joint Commission)[2] and patient‐safety organizations (Institute for Healthcare Improvement 100,000 Live Campaign)[3] have strongly supported RRSs. Decades of evidence show that general ward patients often experience unrecognized deterioration and cardiorespiratory arrest (CA). The low sensitivity and accuracy of periodic assessments by staff are thought to be a major reason for these lapses, as are imbalances between patient needs and clinician (primarily nursing) resources. Additionally, a medical culture that punishes speaking up or bypassing the chain of command are also likely contributors to the problem. A system that effectively recognizes the early signs of deterioration and quickly responds should catch problems before they become life threatening. Over the last decade, RRSs have been the primary intervention implemented to do this. The potential for RRSs to improve outcomes has strong face validity, but researchers have struggled to demonstrate consistent improvements in outcomes across institutions. Given this, are RRSs the best intervention to prevent this failure to rescue? In this editorial we examine the progress of RRSs, how they compare to other options, and we consider whether we should continue to question their implementation.
In our 2007 systematic review,[4] we concluded there was weak to moderate evidence supporting RRSs. Since then, 6 other systematic reviews of the effectiveness or implementation of RRSs have been published. One high‐quality review of effectiveness studies published through 2008 by Chan et al.[5] found that RRSs significantly reduced non‐intensive care unit (ICU) CA (relative risk [RR], 0.66; 95% confidence interval [CI], 0.54‐0.80), but not total hospital mortality (RR, 0.96; 95% CI, 0.84‐1.09) in adult inpatients. In pediatric inpatients, RRSs led to significant improvements in both non‐ICU CA (RR, 0.62; 95% CI, 0.46 to 0.84) and total hospital mortality (RR, 0.79; 95% CI, 0.63 to 0.98). Subsequent to 2008, a structured search[6] finds 26 additional studies.[7, 8, 9, 10, 11, 12, 13, 14, 15, 16, 17, 18, 19, 20, 21, 22, 23, 24, 25, 26, 27, 28, 29, 30] Although the benefit for CA in both adults and children has remained robust, even more so since Chan's review, mortality reductions in adult patients appear to have had the most notable shift. In aggregate, the point estimate (for those studies providing analyzable data), for adult mortality has strengthened to 0.88, with a confidence interval of 0.82‐0.96 in favor of the RRS strategy.
This change has occurred as the analyzable studies since 2008 have all had favorable point estimates, and 4 have had statistically significant confidence intervals. Prior to 2008, 5 had unfavorable point estimates, and only 2 had favorable confidence intervals. As RRSs expand, the benefits, although not universal (some hospitals still experience no improvement in outcomes), seem to be getting stronger and more consistent. This may be secondary to maturation of the intervention and implementation strategies, or it may be the result of secular trends outside of the RRS intervention, although studies controlling for this found it not to be the case.[10] The factors associated with successful implementation of the RRS or improved outcomes include knowledge of activation criteria, communication, teamwork, lack of criticism for activating the RRS, and better attitudes about the team's positive effect on nurses and patients. Many of these factors relate to an improved safety culture in general. Additionally, activation rates may have increased in more recent studies, as greater utilization is associated with improved outcomes.[31] Finally, RRSs, like other patient‐safety and quality interventions, mature with time, often taking several years before they have a full effect on outcomes.[31, 32]
Despite these more favorable results for RRSs, we still see a large discrepancy between the magnitude of benefit for CA and mortality. This may partly be because the exposure groups are different; most studies examined non‐ICU CA, yet studies reporting mortality used total hospital mortality (ICU and non‐ICU). Additionally, although RRSs may effectively prevent CA, this intervention may have a more limited effect in preventing the patient's ultimate demise (particularly in the ICU).
We also still see that effectiveness reports for RRSs continue to be of low to moderate quality. Many reports give no statistics or denominator data or have missing data. Few control for secular trends in providers, outcomes, and confounders. Outcome measures vary widely, and none conducted blinded outcome assessments. Most studies use a pre‐post design without concurrent controls, substantially increasing the risk of bias. The better‐designed studies that use concurrent controls or cluster randomization (Priestley,[33] Bristow,[34] and the MERIT trial[35]) tend to show lower treatment effects, although interestingly in the MERIT trial, while the cluster‐randomized data showed no benefit, the pre‐post data showed significant improvement in the RRS intervention hospitals. These results have been attributed to the control hospitals using their code teams for RRS activities,[36] negating a comparative improvement in the intervention hospitals.
Can we improve RRS research? Likely, yes. We can begin by being more careful about defining the exposure group. Ideally, studies should not include data from the ICU or the emergency department because these patient populations are not part of the exposure group. Although most studies removed ICU and emergency department data for CA, they did not do so for hospital mortality. ICU mortality is likely biased, because only a small proportion of ICU patients have been exposed to an RRS. Definitions also need to be stringent and uniform. For example, CA may be defined in a variety of ways such as calling the code team versus documented cardiopulmonary resuscitation. Unexpected hospital mortality is often defined as excluding patients with do not resuscitate (DNR) orders, but this may or may not accurately exclude expected deaths. We also need to better attempt to control for confounders and secular trends. Outcomes such as CA and mortality are strongly influenced by changes in patient case‐mix over time, the frequency of care limitation/DNR orders, or by poor triage decisions.[37] Outcomes such as unanticipated ICU admission are indirect and may be heavily influenced by local cultural factors. Finally, authors need to provide robust statistical data and clear numerators and denominators to support their conclusions.
Although we need to do our best to improve the quality of the RRS literature, the near ubiquitous presence of this patient‐safety intervention in North American hospitals raises a crucial question, Do we even need more effectiveness studies and if so what kind? Randomized controlled trials are not likely. It is hard to argue that we still sit at a position of equipoise, and randomizing patients who are deteriorating to standard care versus an RRS is neither practical nor ethical. Finding appropriate concurrent control hospitals that have not implemented some type of RRS would also be very difficult.
We should, however, continue to test the effectiveness of RRSs but in a more diverse manner. RRSs should be more directly compared to other interventions that can improve the problem of failure to rescue such as increased nurse staffing[38, 39, 40] and hospitalist staffing.[41] The low sensitivity and accuracy of monitoring vital signs on general wards by staff is also an area strongly deserving of investigation, as it is likely central to the problem. Researchers have sought to use various combinations of vital signs, including aggregated or weighted scoring systems, and recent data suggest some approaches may be superior to others.[42] Many have advocated for continuous monitoring of a limited set of vital signs similar to the ICU, and there are some recent data indicating that this might be effective.[43, 44] This work is in the early stages, and we do not yet know whether this strategy will affect outcomes. It is conceivable that if the false alarm rate can be kept very low and we can minimize the failure to recognize deteriorating patients (good sensitivity, specificity, and positive predictive value), the need for the RRS response team may be reduced or even eliminated. Additionally, as electronic medical records (EMRs) have expanded, there has been growing interest in leveraging these systems to improve the effectiveness of RRSs.[45] There is a tremendous amount of information within the EMRs that can be used to complement vital‐sign monitoring (manual or continuous), because baseline medical problems, laboratory values, and recent history may have a strong impact on the predictive value of changes in vital signs.
Research should also focus on the possible unintended consequences, costs, and the cost‐effectiveness of RRSs compared with other interventions that can or may reduce the rate of failure to rescue. Certainly, establishing RRSs has costs including staff time and the need to pull staff from other clinical duties to respond. Unintended harm, such as diversion of ICU staff from their usual care, are often mentioned but never rigorously evaluated. Increasing nurse staffing has very substantial costs, but how these costs compare to the costs of the RRS are unclear, although likely the comparison would be very favorable to the RRS, because staffing typically relies on existing employees with expertise in caring for the critically ill as opposed to workforce expansion. Given the current healthcare economic climate, any model that relies on additional employees is not likely to gain support. Establishing continuous monitoring systems have up‐front capital costs, although they may reduce other costs in the long run (eg, staff, medical liability). They also have intangible costs for provider workload if the false alarm rates are too high. Again, this strategy is too new to know the answers to these concerns. As we move forward, such evaluations are needed to guide policy decisions.
We also need more evaluation of RRS implementation science. The optimal way to organize, train, and staff RRSs is unknown. Most programs use physician‐led teams, although some use nurse‐led teams. Few studies have compared the various models, although 1 study that compared a resident‐led to an attending‐led team found no difference.[17] Education is ubiquitous, although actual staff training (simulation for example) is not commonly described. In addition, there is wide variation in the frequency of RRS activation. We know nurses and residents often feel pressured not to activate RRSs, and much of the success of the RRS relies on nurses identifying deteriorating patients and calling the response team. The use of continuous monitoring combined with automatic notification of staff may reduce the barriers to activating RRSs, increasing activation rates, but until then we need more understanding of how to break down these barriers. Family/patient access to activation has also gained ground (1 program demonstrated outcome improvement only after this was established[13]), but is not yet widespread.
The role of the RRS in improving processes of care, such as the appropriate institution of DNR orders, end of life/palliative care discussions, and early goal‐directed therapy for sepsis, have been presented in several studies[46, 47] but remain inadequately evaluated. Here too, there is much to learn about how we might realize the full effectiveness of this patient‐safety strategy beyond outcomes such as CA and hospital mortality. Ideally, if all appropriate patients had DNR orders and we stopped failing to recognize and respond to deteriorating ward patients, CAs on general hospital wards could be nearly eliminated.
RRSs have been described as a band‐aid for a failed model of general ward care.[37] What is clear is that many patients suffer preventable harm from unrecognized deterioration. This needs to be challenged, but are RRSs the best intervention? Despite the Joint Commission's Patient Safety Goal 16, should we still question their implementation? Should we (and the Joint Commission) reconsider our approach and prioritize our efforts elsewhere or should we feel comfortable with the investment that we have made in these systems? Even though there are many unknowns, and the quality of RRS studies needs improvement, the literature is accumulating that RRSs do reduce non‐ICU CA and improve hospital mortality. Without direct comparison studies demonstrating superiority of other expensive strategies, there is little reason to reconsider the RRS concept or question their implementation and our investment. We should instead invest further in this foundational patient‐safety strategy to make it as effective as it can be.
Disclosures: Dr. Pronovost reports the following potential conflicts of interest: grant or contract support from the Agency for Healthcare Research and Quality, and the Gordon and Betty Moore Foundation (research related to patient safety and quality of care), and the National Institutes of Health (acute lung injury research); consulting fees from the Association of Professionals in Infection Control and Epidemiology, Inc.; honoraria from various hospitals, health systems, and the Leigh Bureau to speak on quality and patient safety; book royalties from the Penguin Group; and board membership for the Cantel Medical Group. Dr. Winters reports the following potential conflicts of interest: contract or grant support from Masimo Corporation, honoraria from 3M Corporation and various hospitals and health systems, royalties from Lippincott Williams &Wilkins (UptoDate), and consulting fees from several legal firms for medical legal consulting.
- Rapid response teams: walk, don't run. JAMA. 2006;296:1645–1647. , , .
- Joint Commission requirement: The Joint Commission announces the 2008 National Patient Safety Goals and Requirements. Jt Comm Perspect. 2007;27(7):1–22.
- Institute for Healthcare Improvement. 5 million lives campaign: overview. Available at: http://www.ihi.org/offerings/Initiatives/PastStrategicInitiatives/5MillionLivesCampaign/Pages/default.aspx. Accessed November 28, 2012.
- Rapid response systems: a systematic review. Crit Care Med. 2007;35:1238–1243. , , , , , .
- Rapid response teams: a systematic review and meta‐analysis. Arch Intern Med. 2010;170:18–26. , , , , .
- Rapid‐response systems as a patient safety strategy: a systematic review. Ann Intern Med. 2013;158:417–425. , , , , , .
- Hospital‐wide code rates and mortality before and after implementation of a rapid response team. JAMA. 2008;300:2506–2513. , , , , , .
- Experience of pediatric rapid response team in a tertiary care hospital in Pakistan. Indian J Pediatr. 2010;77:273–276. , , , .
- Rescue me: saving the vulnerable non‐ICU patient population. Jt Comm J Qual Patient Saf. 2009;35:199–205. , , , et al.
- Reduction in hospital‐wide mortality after implementation of a rapid response team: a long‐term cohort study. Crit Care. 2011;15:R269. , , , , .
- Using an advanced practice nursing model for a rapid response team. Jt Comm J Qual Patient Saf. 2008;34:743–747. , , , , .
- Immediate and long‐term impact of medical emergency teams on cardiac arrest prevalence and mortality: a plea for periodic basic life‐support training programs. Crit Care Med. 2009;37:3054–3061. , , , , , .
- Successful implementation of a family and patient activated rapid response team in an adult level 1 trauma center. Resuscitation. 2010;81:1676–1681. , , , , , .
- A reduction in cardiac arrests and duration of clinical instability after implementation of a paediatric rapid response system. Qual Saf Health Care. 2009;18:500–504. , , , et al.
- Implementing a rapid response team to decrease emergencies outside the ICU: one hospital's experience. Medsurg Nurs. 2009;18:84–90, 126. , , , et al.
- Sustained effectiveness of a primary‐team‐based rapid response system. Crit Care Med. 2012;40:2562–2568. , , , et al.
- Association between implementation of an intensivist‐led medical emergency team and mortality. BMJ Qual Saf. 2012;21:152–159. , , , .
- Reducing in‐hospital cardiac arrests and hospital mortality by introducing a medical emergency team. Intensive Care Med. 2010;36:100–106. , , , , , .
- Implementation of a multicenter rapid response system in pediatric academic hospitals is effective. Pediatrics. 2011;128:72–78. , , , et al.
- The impact of medical emergency teams on ICU admission rates, cardiopulmonary arrests and mortality in a regional hospital. Resuscitation. 2011;82:707–712. , .
- Introduction of a rapid response system at a United States veterans affairs hospital reduced cardiac arrests. Anesth Analg. 2010;111:679–686. , , , .
- The effect of the medical emergency team on unexpected cardiac arrest and death at the VA Caribbean healthcare system: a retrospective study. Crit Care Shock. 2010;13:98–105. , , , .
- Four years' experience with a hospitalist‐led medical emergency team: an interrupted time series. J Hosp Med. 2012;7:98–103. , , , , .
- Changing cardiac arrest and hospital mortality rates through a medical emergency team takes time and constant review. Crit Care Med. 2010;38:445–450. , , .
- Clinical emergencies and outcomes in patients admitted to a surgical versus medical service. Resuscitation. 2011;82:415–418. , , , et al.
- Evaluating a new rapid response team: NP‐led versus intensivist‐led comparisons. AACN Adv Crit Care. 2012;23:32–42. , , , .
- Implementation of a rapid response team: a success story. Crit Care Nurse. 2009;29:66–75. , .
- Rapid response team in an academic institution: does it make a difference? Chest. 2011;139:1361–1367. , , , .
- Reduction of hospital mortality and of preventable cardiac arrest and death on introduction of a pediatric medical emergency team. Ped Crit Care Med. 2009;10:306–312. , .
- Medical emergency teams are associated with reduced mortality across a major metropolitan health network after two years service: a retrospective study using government administrative data. Crit Care. 2012;16:R210. , .
- Long term effect of a medical emergency team on cardiac arrests in a teaching hospital. Crit Care. 2005;9:R808–R815. , , , , et al.
- Six year audit of cardiac arrests and medical emergency team calls in an Australian outer metropolitan teaching hospital. BMJ. 2007;335:1210–1212. , , , .
- Introducing Critical Care Outreach: a ward‐randomised trial of phased introduction in a general hospital. Intensive Care Med. 2004;30:1398–1404. , , , et al.
- Rates of in‐hospital arrests, deaths, and intensive care admissions: the effect of a medical emergency team. Med J Aust. 2000;173:236–240. , , , et al.
- Introduction of the medical emergency team (MET) system: a cluster randomised controlled trial. Lancet. 2005;365:2091–2097. , , , et al.
- The effectiveness of implementation of the medical emergency team (MET) system and factors associated with use during the MERIT study. Crit Care Resusc. 2007;9:206–212. , , , , , .
- Rethinking rapid response teams. JAMA. 2010;304:1375–1376. , .
- Lower mortality for abdominal aortic aneurysm repair in high‐volume hospitals is contingent upon nurse staffing [published online ahead of print October 22, 2012]. Health Serv Res. doi: 10.1111/1475–6773.12004. , , .
- Nurse‐staffing levels and the quality of care in hospitals. N Engl J Med. 2002;346:1715–1722. , , , , .
- The association of registered nurse staffing levels and patient outcomes: systematic review and meta‐analysis. Med Care. 2007;45:1195–1204. .
- Outcomes of care by hospitalists, general internists, and family physicians. N Engl J Med. 2007;357:2589–2600. , , , , , .
- The ability of the National Early Warning Score (NEWS) to discriminate patients at risk of early cardiac arrest, unanticipated intensive care unit admission, and death. Resuscitation. 2013;84:465–470. , , , , .
- Impact of pulse oximetry surveillance on rescue events and intensive care unit transfers: a before‐and‐after concurrence study. Anesthesiology. 2010;112:282–287. , , , .
- A controlled trial of electronic automated advisory vital signs monitoring in general hospital wards. Crit Care Med. 2012;40:2349–2361. , , , et al.
- Agency for Healthcare Research and Quality. Early warning scoring system proactively identifies patients at risk of deterioration, leading to fewer cardiopulmonary emergencies and deaths. Available at: http://www.innovations.ahrq.gov/content.aspx?id=2607. Accessed March 26, 2013.
- Effect of a rapid response system for patients in shock on time to treatment and mortality during 5 years. Crit Care Med. 2007;35:2568–2575. , , , et al.
- The medical emergency team and end‐of‐life care: a pilot study. Crit Care Resusc. 2007;9:151–156. , , , , , .
- Rapid response teams: walk, don't run. JAMA. 2006;296:1645–1647. , , .
- Joint Commission requirement: The Joint Commission announces the 2008 National Patient Safety Goals and Requirements. Jt Comm Perspect. 2007;27(7):1–22.
- Institute for Healthcare Improvement. 5 million lives campaign: overview. Available at: http://www.ihi.org/offerings/Initiatives/PastStrategicInitiatives/5MillionLivesCampaign/Pages/default.aspx. Accessed November 28, 2012.
- Rapid response systems: a systematic review. Crit Care Med. 2007;35:1238–1243. , , , , , .
- Rapid response teams: a systematic review and meta‐analysis. Arch Intern Med. 2010;170:18–26. , , , , .
- Rapid‐response systems as a patient safety strategy: a systematic review. Ann Intern Med. 2013;158:417–425. , , , , , .
- Hospital‐wide code rates and mortality before and after implementation of a rapid response team. JAMA. 2008;300:2506–2513. , , , , , .
- Experience of pediatric rapid response team in a tertiary care hospital in Pakistan. Indian J Pediatr. 2010;77:273–276. , , , .
- Rescue me: saving the vulnerable non‐ICU patient population. Jt Comm J Qual Patient Saf. 2009;35:199–205. , , , et al.
- Reduction in hospital‐wide mortality after implementation of a rapid response team: a long‐term cohort study. Crit Care. 2011;15:R269. , , , , .
- Using an advanced practice nursing model for a rapid response team. Jt Comm J Qual Patient Saf. 2008;34:743–747. , , , , .
- Immediate and long‐term impact of medical emergency teams on cardiac arrest prevalence and mortality: a plea for periodic basic life‐support training programs. Crit Care Med. 2009;37:3054–3061. , , , , , .
- Successful implementation of a family and patient activated rapid response team in an adult level 1 trauma center. Resuscitation. 2010;81:1676–1681. , , , , , .
- A reduction in cardiac arrests and duration of clinical instability after implementation of a paediatric rapid response system. Qual Saf Health Care. 2009;18:500–504. , , , et al.
- Implementing a rapid response team to decrease emergencies outside the ICU: one hospital's experience. Medsurg Nurs. 2009;18:84–90, 126. , , , et al.
- Sustained effectiveness of a primary‐team‐based rapid response system. Crit Care Med. 2012;40:2562–2568. , , , et al.
- Association between implementation of an intensivist‐led medical emergency team and mortality. BMJ Qual Saf. 2012;21:152–159. , , , .
- Reducing in‐hospital cardiac arrests and hospital mortality by introducing a medical emergency team. Intensive Care Med. 2010;36:100–106. , , , , , .
- Implementation of a multicenter rapid response system in pediatric academic hospitals is effective. Pediatrics. 2011;128:72–78. , , , et al.
- The impact of medical emergency teams on ICU admission rates, cardiopulmonary arrests and mortality in a regional hospital. Resuscitation. 2011;82:707–712. , .
- Introduction of a rapid response system at a United States veterans affairs hospital reduced cardiac arrests. Anesth Analg. 2010;111:679–686. , , , .
- The effect of the medical emergency team on unexpected cardiac arrest and death at the VA Caribbean healthcare system: a retrospective study. Crit Care Shock. 2010;13:98–105. , , , .
- Four years' experience with a hospitalist‐led medical emergency team: an interrupted time series. J Hosp Med. 2012;7:98–103. , , , , .
- Changing cardiac arrest and hospital mortality rates through a medical emergency team takes time and constant review. Crit Care Med. 2010;38:445–450. , , .
- Clinical emergencies and outcomes in patients admitted to a surgical versus medical service. Resuscitation. 2011;82:415–418. , , , et al.
- Evaluating a new rapid response team: NP‐led versus intensivist‐led comparisons. AACN Adv Crit Care. 2012;23:32–42. , , , .
- Implementation of a rapid response team: a success story. Crit Care Nurse. 2009;29:66–75. , .
- Rapid response team in an academic institution: does it make a difference? Chest. 2011;139:1361–1367. , , , .
- Reduction of hospital mortality and of preventable cardiac arrest and death on introduction of a pediatric medical emergency team. Ped Crit Care Med. 2009;10:306–312. , .
- Medical emergency teams are associated with reduced mortality across a major metropolitan health network after two years service: a retrospective study using government administrative data. Crit Care. 2012;16:R210. , .
- Long term effect of a medical emergency team on cardiac arrests in a teaching hospital. Crit Care. 2005;9:R808–R815. , , , , et al.
- Six year audit of cardiac arrests and medical emergency team calls in an Australian outer metropolitan teaching hospital. BMJ. 2007;335:1210–1212. , , , .
- Introducing Critical Care Outreach: a ward‐randomised trial of phased introduction in a general hospital. Intensive Care Med. 2004;30:1398–1404. , , , et al.
- Rates of in‐hospital arrests, deaths, and intensive care admissions: the effect of a medical emergency team. Med J Aust. 2000;173:236–240. , , , et al.
- Introduction of the medical emergency team (MET) system: a cluster randomised controlled trial. Lancet. 2005;365:2091–2097. , , , et al.
- The effectiveness of implementation of the medical emergency team (MET) system and factors associated with use during the MERIT study. Crit Care Resusc. 2007;9:206–212. , , , , , .
- Rethinking rapid response teams. JAMA. 2010;304:1375–1376. , .
- Lower mortality for abdominal aortic aneurysm repair in high‐volume hospitals is contingent upon nurse staffing [published online ahead of print October 22, 2012]. Health Serv Res. doi: 10.1111/1475–6773.12004. , , .
- Nurse‐staffing levels and the quality of care in hospitals. N Engl J Med. 2002;346:1715–1722. , , , , .
- The association of registered nurse staffing levels and patient outcomes: systematic review and meta‐analysis. Med Care. 2007;45:1195–1204. .
- Outcomes of care by hospitalists, general internists, and family physicians. N Engl J Med. 2007;357:2589–2600. , , , , , .
- The ability of the National Early Warning Score (NEWS) to discriminate patients at risk of early cardiac arrest, unanticipated intensive care unit admission, and death. Resuscitation. 2013;84:465–470. , , , , .
- Impact of pulse oximetry surveillance on rescue events and intensive care unit transfers: a before‐and‐after concurrence study. Anesthesiology. 2010;112:282–287. , , , .
- A controlled trial of electronic automated advisory vital signs monitoring in general hospital wards. Crit Care Med. 2012;40:2349–2361. , , , et al.
- Agency for Healthcare Research and Quality. Early warning scoring system proactively identifies patients at risk of deterioration, leading to fewer cardiopulmonary emergencies and deaths. Available at: http://www.innovations.ahrq.gov/content.aspx?id=2607. Accessed March 26, 2013.
- Effect of a rapid response system for patients in shock on time to treatment and mortality during 5 years. Crit Care Med. 2007;35:2568–2575. , , , et al.
- The medical emergency team and end‐of‐life care: a pilot study. Crit Care Resusc. 2007;9:151–156. , , , , , .
Delayed Antimicrobial Administration
In‐hospital administration of antimicrobials is often subject to controls and policies designed to limit the indiscriminant use of antimicrobials in situations where they are not warranted, to control costs, and to reduce the potential for the development of resistant microorganismsa major public health threat and patient safety concern. These controls and policies may include strategies such as limiting the choices of antimicrobials on formulary, rotational schedules of available antimicrobials, and antimicrobial approval processes.16 Antimicrobial approval processes commonly require that the bedside clinician obtain permission from a secondary source to administer a particular antimicrobial. This approval process may take the form of submitting written justification forms and/or direct telephone/fax requests to an Infectious Disease specialist, Clinical Pharmacist, or other surrogate prior to release of the antibiotic from the pharmacy. While these approval processes and other strategies have been shown to reduce the development of resistance,7, 8 improve outcomes,8 and provide education and revision of antimicrobial choice that may be more appropriate for the patient and suspected infection,9 they have the potential to delay the administration of the necessary antimicrobial by adding additional steps to the sequence of ordering, obtaining, and administering the medication. While it is certainly desirable to control the indiscriminant use of these medications, delays in antimicrobial administration may, in turn, worsen outcomes, thus counteracting the beneficial effects of control policies. The early and timely administration of appropriate broad spectrum antimicrobials chosen to cover the most expected organisms has been consistently shown to improve outcomes1027 and has been cited as an essential element of the Surviving Sepsis Campaign (
At our institution, the antimicrobial approval process requires written justification forms and/or a call or fax to an Infectious Disease specialist or Clinical Pharmacist prior to the antimicrobial being released from the pharmacy. We hypothesized that this process is associated with significant delays in patients receiving their prescribed antimicrobials when a restricted drug was chosen. The antibiotic approval process at our institution allows 1‐time stat doses of restricted antimicrobials to be ordered without preapproval at night (10 PM to 8 AM), but not during the day. This allowed us to compare the time‐to‐administration of restricted antimicrobials to their unrestricted counterparts and to themselves during this exempt time period.
Methods
Study Design
This was a single‐institution retrospective cohort study. We included all patients admitted to Johns Hopkins Hospital units utilizing the hospital's computerized provider order entry system (CPOE) who had stat orders placed for any of 24 preselected commonly ordered intravenous antimicrobials over a 1‐year period between November 1, 2005 and October 31, 2006. We did not include oral medications that were similarly subject to the approval process since we anticipated that the amount of time required to prepare the drug in the pharmacy and to deliver it to the patient would be systematically different than for intravenous medications. The CPOE system captures time of administration of all drugs (as charted by the nurse) in addition to the time of order entry by the provider. Institutional guidelines dictate that when drugs are ordered stat, they should be administered within 30 minutes of the order.
Classification of Antibiotics
Particular antimicrobials (24 different drugs) were chosen prospectively to include a broad range of commonly ordered drugs available at our hospital in intravenous form (Table 1). For this analysis, we only considered the first dose of the prescribed antimicrobial, since subsequent doses are not generally ordered stat and the timing of administration is clinically less important than the timing of the initial dose. Additionally, for patients who had multiple orders for stat antimicrobials during the course of hospitalization, we only included the first stat order in analysis.
Restricted Antimicrobials | Unrestricted Antimicrobials |
---|---|
Ampicillin/sulbactam | Acyclovir |
Azithromycin | Amphotericin |
Cefepime | Ampicillin |
Ciprofloxacin | Cefazolin |
Fluconazole | Ceftriaxone |
Gatifloxacin | Cephalexin |
Linezolid | Clindamycin |
Meropenem | Doxycycline |
Moxifloxacin | Ertapenem |
Vancomycin | Gentamicin |
Metronidazole | |
Oxacillin | |
Tobramycin | |
Trimethoprim/sulfamethoxazole |
For the purposes of the analysis, we defined antimicrobials as restricted or unrestricted. At our institution, restricted antimicrobials require either written or telephone approval from an Infectious Disease physician or Clinical Pharmacist. Obtaining written approval involves filling out a form that indicates the choice of agent (including route and dose) for an approved indication (eg, vancomycin for proven methicillin‐resistant Staphylococcus aureus bacteremia) and faxing this form to the hospital pharmacy. In other cases, approval requires a phone conversation with an Infectious Disease clinical fellow or a Clinical Pharmacist. None of these processes can be initiated or executed from within the CPOE system itself.
At our institution, the first dose of any restricted antimicrobial may be administered without going through the approval process if it is prescribed in the overnight hours defined as between 10 PM and 8 AM (Table 2). Retroactive approval is required the following day if the antimicrobial is to be continued.
Restricted Antimicrobial (eg, Vancomycin) | Unrestricted Antimicrobial (eg, Cefazolin) | |
---|---|---|
Daytime order (8 AM to 10 PM) | Approval required | No approval required |
Nighttime order (10 PM to 8 AM) | Exempted from approval for first dose (approval required during the next daytime period) | No approval required |
Data Acquisition
All antibiotic administration data were extracted electronically from the CPOE system. During the time of the study, not all hospital units utilized this system. Although CPOE was in place for all general medical wards, the intensive care units and many surgical services were not using CPOE at the time of the study. Based on limitations of the data entered into our CPOE system, many relevant clinical variables were not available for this study, such as clinical diagnoses or the level of care (eg, intensive care unit vs step‐down unit, vs regular nursing floor) at the time of the order.
Outcome Measures
We defined time‐to‐administration as the period of time between order entry and administration of the drug to the patient, as charted by the nurse in the CPOE system. However, nursing policy allows nurses to document the time of administration as the exact time of the order provided that the drug is delivered within 1 hour of a stat order, whereas all administration times beyond 1 hour of the order are expected to be documented precisely. This nursing policy eliminated our ability to determine whether antimicrobials were administered within 30 minutes of the order, and limited the value of examining time‐to‐administration as a continuous variable, but allowed us to reliably identify delays of >1 hour or >2 hours presuming that the nurse‐charted administration times were accurate. As our primary outcome, we defined delay in administration as when the antimicrobial was administered >1 hour from time of order. We examined delays of >2 hours as a secondary outcome. Antimicrobials ordered stat but not delivered within 4 hours of the order were excluded from analysis based on the recognition that extended delays might have resulted from changes in clinical circumstances or errors in documentation. Similarly, antimicrobials charted as being delivered prior to the order were excluded.
Statistical Analyses
We used the likelihood‐ratio chi‐square test to determine 2‐sided P values for differences between proportions. Logistic regression models were used to derive odds ratios (ORs) and to adjust for covariables. We adjusted for weekday versus weekend orders, patient characteristics including sex, age, white versus non‐white ethnicity and orders placed on medicine versus non‐medicine (eg, surgical or obstetrical) wards. Analyses were conducted in JMP 5.1 (SAS Institute, Cary, NC).
Results
In total, 3337 orders for stat antimicrobials were written during the study period, of which 86 (2.6%) were excluded based on being outside the specified 4‐hour window. This left a total of 3251 orders in 3251 discrete patients for analysis.
We found that a statistically significantly higher percentage of delays in antimicrobial administration when the antimicrobial was restricted as compared to unrestricted. This was the case for both our primary outcome of a >1 hour delay (Figure 1) and our secondary outcome of a delay of >2 hours (Figure 2). For restricted antimicrobial, delays of >1 hr occurred with 46.1% of orders during the day and with 38.8% of orders at night (when exempt from approval), P < 0.001. For unrestricted antimicrobials, delays of >1 hr occurred in 36.4% and 36.6% of instances, respectively (P = 0.57). The odds ratio for a delay in administration of 1 hour for restricted antimicrobials was 1.49 (95% CI = 1.23‐1.82).
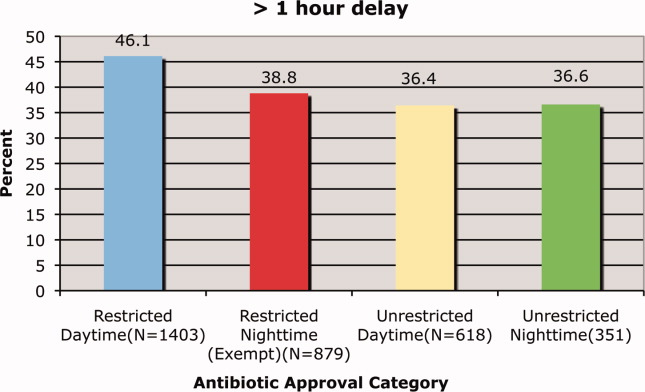
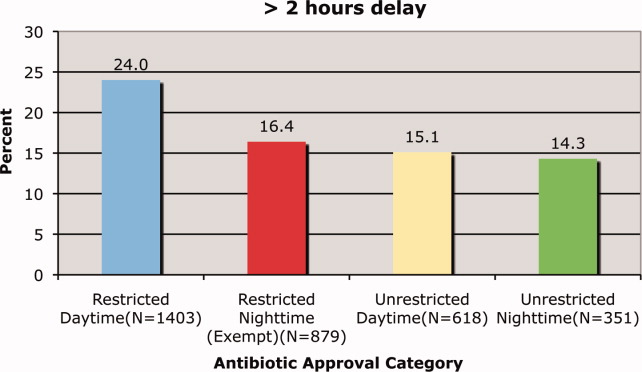
Delays beyond 2 hours occurred 24.0% of the time for restricted antimicrobials during the day versus 16.4% at night. Unrestricted antimicrobials were delayed >2 hrs only 15.1% and 14.3% of the time for day and night periods, respectively (P = 0.35). The odds ratio for a two‐hour or greater delay was 1.78 (95% CI = 1.39‐2.21), P < 0.0001 when the antimicrobial was restricted.
These odds ratios and statistical significance were unchanged by adjustment for primary service (medicine vs. non‐medicine), age, sex, ethnicity or whether the order was placed on a weekend or weekday (data not shown).
Discussion
We found that our institution's antimicrobial approval process was associated with statistically significant delays in the administration of antimicrobials that were ordered stat by the prescribing clinician. These delays were evident both when comparing restricted antimicrobials to unrestricted ones and when these restricted antimicrobials were compared to themselves during the overnight time period when they were temporarily exempt from the approval process. This suggests that the delay is associated with the approval process itself and not the specific drug or the time of day. We also found that over one‐third of all stat antimicrobial orders were not carried out in the within one hour. This rate approached nearly 50% for restricted drugs ordered stat. This high baseline rate for all stat‐ordered antimicrobials underscores system challenges that seem to be exacerbated when restricted antimicrobials are chosen.
We do not know if the delays we observed resulted in patient harm. Indeed it is possible, if not likely, that patient care at our institution is improved by the judicious use of certain antimicrobials, even if the process required to enforce their use may result in delayed antimicrobial administration in some instances. Since we did not collect clinical information on baseline diagnoses or severity of illness, and we did not have information on clinical outcomes, we cannot determine whether the clinical delays we observed might have caused harm. Determining the impact these approval policies have on patient outcomes would require a separate study designed to collect the necessary clinical data to answer that question.
An additional limitation was that we did not ascertain the indications for the antimicrobials to determine whether they truly needed to be given stat. We suspect that antimicrobials are sometimes ordered stat even when the infection being treated is not likely to be serious or life‐threatening. Additionally, since we relied on the nurse‐charted time of administration, it is possible that in some instances there was a charted delay in administration when in reality the patient received the antimicrobial in a timely fashion. In urgent situations, the nurses may be too busy to document that the medication was given until long after the dose is given, and this may result in inaccuracies in the charted administration time. However, this type of documentation error would be expected to affect restricted and unrestricted antimicrobials similarly and would be unlikely to result in a systematic bias.
Because we conducted this study at a single institution, the results may not be applicable to other medical institutions, especially since restriction policies and antimicrobial approval processes vary from hospital to hospital. The burden of delays may be related to the number of restricted antimicrobials on formulary, the types of antimicrobials restricted, the number of steps required to have them released from the pharmacy, whether the approval process is initiated from within the order entry system, and other factors that may streamline or hamper the approval process.
In our institution, there are several steps in the process, any of which might contribute to the delay. Faxed approval sheets may take time to arrive to and be acted upon by the pharmacy, errant pages may delay communication between the provider and the person providing approval, and there may be delays in the final approval being relayed to the pharmacy by the individual providing approval. In fact, an alternative explanation for the observed administration delays is that once ordered in CPOE, the prescribing physicians themselves are slow in initiating the approval process. While this is certainly possible, especially given the stresses surrounding the management of a seriously ill patient on the general ward, this still suggests that having to go through the approval process may impact the process of care.
Other possible explanations for the delays observed when the restrictive antimicrobial policy was in effect may include pharmacy staffing. Since the workload in the pharmacy would be expected to be greater during the day, when more patient care activity is occurring such as clinics and operating rooms, this increased workload may have slowed down the pharmacy filling the orders. However, such human resource‐workload imbalances would also be expected to slow most pharmacy processes and should lead to delays in filling the orders for other medications including the unrestricted antimicrobials. We did not track other non‐antimicrobial medications to examine their patterns of delay. Nursing workload also varies between day and night but the time period where the antimicrobial administration delays occurred is the time when nursing is favorably staffed unlike the night when nurse to patient ratios are low. It is possible that despite better nurse to patient ratios during the day, the workload‐to‐nursing ratio remains high and contributes to delays in administration of otherwise stat‐ordered antimicrobials. Again, it is unclear why this would disproportionately affect the restricted class of antimicrobials.
We do not advocate the abandonment of antimicrobial control policies. The process described here is very institution‐specific and while its benefits are proven, energy should be channeled where appropriate to facilitate this process. These policies are clearly necessary to help reduce costs, limit the unwarranted use of these drugs, and slow the proliferation of ever more resistant strains of microorganisms. However, we do advocate careful consideration of the components of the approval process itself, ensuring that delays in antimicrobial administration are kept to a minimum and are avoided altogether in critically ill patients. One way to accomplish this might be to not require approval for the first administration of a stat antibiotic, but to require approval for subsequent doses. Our institution's overnight exempt period data suggest that this would eliminate the incremental delays incurred by the approval process itself. As important, our results show that even for unrestricted antibiotics, we fall short of achieving recommended best practices, highlighting the challenges inherent to carrying out multi‐step clinical tasks in an efficient fashion.
- Antimicrobial stewardship.Am J Med.2006;119:S53–S61. .
- Restrictive antibiotic policies are appropriate in intensive care units.Crit Care Med.2003;31:S25–S28. .
- Effects of requiring prior authorization for selected anti‐microbials: expenditures, susceptibilities and clinical outcomes.Clin Infect Dis.1997;25:230–239. , , , et al.
- Class restriction of cephalosporin use to control total cephaslosporin resistance in nosocomial Klebsiella.JAMA.1998;280:1233–1237. , , , et al.
- Rotation and restricted use of antibiotics in a medical intensive care unit: impact on the incidence of ventilator associated pneumonia caused by antibiotic resistant Gram‐negative bacteremia.Am J Respir Crit Care Med.2001;63(3 Pt 1):837–843. , , , et al.
- Impact of rotation of empiric antibiotic schedules on infectious mortality in an intensive care unit.CCM.2001;29:1101–1108. , , , et al.
- Antimicrobial use control measures to prevent and control antimicrobial resistance in US hospitals.Infect Control Hosp Epidemiol.2006;27:1088–1095. , , , et al.
- Cost‐effectiveness of prospective and continuous parenteral antibiotic control: experience at the Palo Alto Veterans Affairs Medical Center from 1987 to 1989.Am J Med.1991;90:439–444. , , , , .
- Programmatic role of the infectious diseases physician in controlling antimicrobial costs in the hospital.Clin Infect Dis.1997;24:471–485. , .
- Antimicrobial stewardship and the role of pharmacokinetics–pharmacodynamics in the modern antibiotic era.Diagn Microbiol Infect Dis.2007;57(3 Suppl.):77S–83S. , .
- Improving inpatient antibiotic prescribing: insights from participation in a national collaborative.Jt Comm J Qual Improv.2001;27:387–402. , , , , , .
- Managing antibiotic resistance.N Engl J Med.2000;343:1961–1963. , .
- Antimicrobial stewardship programs in health care systems.Clin Microbiol Rev.2005;18(4):638–656. , .
- Interventions to improve antibiotic prescribing practices for hospital inpatients.Cochrane Database Syst Rev.2005;4:CD003543. , , , , , , , , , , .
- Evaluation of an antimicrobial stewardship program at a pediatric teaching hospital.Pediatr Infect Dis J.2008;27(2):106–111. , , , , .
- The utility of hospital antibiograms as tools for guiding empiric therapy and tracking resistance. Insights from the Society of Infectious Diseases Pharmacists.Pharmacotheray.2007;27(9):1306–1312. .
- Strategies to contain the emergence of antimicrobial resistance: a systematic review of effectiveness and cost‐effectiveness.J Health Serv Res Policy.2002;7(2):111–117. , , , .
- Infectious Diseases Society of America;Society for Healthcare Epidemiology of America.Infectious Diseases Society of America and the Society for Healthcare Epidemiology of America guidelines for developing an institutional program to enhance antimicrobial stewardship.Clin Infect Dis.2007;44(2):159–177. , , , , , , , , , , , , ;
- the Healthcare Infection Control Practices Advisory Committee. Management of Multi‐Drug Reistsant Organisms in Healthcare Settings: 2006. Available at: http://www.cdc.gov/ncidod/dhqp/pdf/ar/MDROGuideline2006.pdf. Accessed May 19,2008. , , , , and
- Gram‐negative bacteremia. IV Re‐evaluation of clinical features and treatment in 612 patients.Am J Med.1980;68:344–355. , , .
- Gram‐negative bacteremia. III. Reassessment of etiology, epidemiology and ecology in 612 patients.Am J Med.1980;68:332–343. , , , .
- Inadequate antimicrobial treatment of infections: a risk factor for hospital mortality among critically ill patients.Chest.1999;115:462–474. , , , .
- Modification of empiric antibiotic treatment in patients with pneumonia acquired in the intensive care unit. ICU‐Acquired Pneumonia Study Group.Intensive Care Med.1996;22:387–394. .
- The value of routine microbial investigation in ventilator‐associated pneumonia.Am J Respir Crit Care Med.1997;156:196–200. , , , , .
- Impact of BAL data on the therapy and outcome of ventilator‐associated pneumonia.Chest.1997;111:676–685. , , , et al.
- The influence of mini‐BAL cultures on patient outcomes: implications for the antibiotic management of ventilator associated pneumonia.Chest.1998;113:412–420. , .
- The influence of inadequate antimicrobial treatment of bloodstream infections on patient outcomes in the ICU setting.Chest.2000;118:146–155. , , , , .
In‐hospital administration of antimicrobials is often subject to controls and policies designed to limit the indiscriminant use of antimicrobials in situations where they are not warranted, to control costs, and to reduce the potential for the development of resistant microorganismsa major public health threat and patient safety concern. These controls and policies may include strategies such as limiting the choices of antimicrobials on formulary, rotational schedules of available antimicrobials, and antimicrobial approval processes.16 Antimicrobial approval processes commonly require that the bedside clinician obtain permission from a secondary source to administer a particular antimicrobial. This approval process may take the form of submitting written justification forms and/or direct telephone/fax requests to an Infectious Disease specialist, Clinical Pharmacist, or other surrogate prior to release of the antibiotic from the pharmacy. While these approval processes and other strategies have been shown to reduce the development of resistance,7, 8 improve outcomes,8 and provide education and revision of antimicrobial choice that may be more appropriate for the patient and suspected infection,9 they have the potential to delay the administration of the necessary antimicrobial by adding additional steps to the sequence of ordering, obtaining, and administering the medication. While it is certainly desirable to control the indiscriminant use of these medications, delays in antimicrobial administration may, in turn, worsen outcomes, thus counteracting the beneficial effects of control policies. The early and timely administration of appropriate broad spectrum antimicrobials chosen to cover the most expected organisms has been consistently shown to improve outcomes1027 and has been cited as an essential element of the Surviving Sepsis Campaign (
At our institution, the antimicrobial approval process requires written justification forms and/or a call or fax to an Infectious Disease specialist or Clinical Pharmacist prior to the antimicrobial being released from the pharmacy. We hypothesized that this process is associated with significant delays in patients receiving their prescribed antimicrobials when a restricted drug was chosen. The antibiotic approval process at our institution allows 1‐time stat doses of restricted antimicrobials to be ordered without preapproval at night (10 PM to 8 AM), but not during the day. This allowed us to compare the time‐to‐administration of restricted antimicrobials to their unrestricted counterparts and to themselves during this exempt time period.
Methods
Study Design
This was a single‐institution retrospective cohort study. We included all patients admitted to Johns Hopkins Hospital units utilizing the hospital's computerized provider order entry system (CPOE) who had stat orders placed for any of 24 preselected commonly ordered intravenous antimicrobials over a 1‐year period between November 1, 2005 and October 31, 2006. We did not include oral medications that were similarly subject to the approval process since we anticipated that the amount of time required to prepare the drug in the pharmacy and to deliver it to the patient would be systematically different than for intravenous medications. The CPOE system captures time of administration of all drugs (as charted by the nurse) in addition to the time of order entry by the provider. Institutional guidelines dictate that when drugs are ordered stat, they should be administered within 30 minutes of the order.
Classification of Antibiotics
Particular antimicrobials (24 different drugs) were chosen prospectively to include a broad range of commonly ordered drugs available at our hospital in intravenous form (Table 1). For this analysis, we only considered the first dose of the prescribed antimicrobial, since subsequent doses are not generally ordered stat and the timing of administration is clinically less important than the timing of the initial dose. Additionally, for patients who had multiple orders for stat antimicrobials during the course of hospitalization, we only included the first stat order in analysis.
Restricted Antimicrobials | Unrestricted Antimicrobials |
---|---|
Ampicillin/sulbactam | Acyclovir |
Azithromycin | Amphotericin |
Cefepime | Ampicillin |
Ciprofloxacin | Cefazolin |
Fluconazole | Ceftriaxone |
Gatifloxacin | Cephalexin |
Linezolid | Clindamycin |
Meropenem | Doxycycline |
Moxifloxacin | Ertapenem |
Vancomycin | Gentamicin |
Metronidazole | |
Oxacillin | |
Tobramycin | |
Trimethoprim/sulfamethoxazole |
For the purposes of the analysis, we defined antimicrobials as restricted or unrestricted. At our institution, restricted antimicrobials require either written or telephone approval from an Infectious Disease physician or Clinical Pharmacist. Obtaining written approval involves filling out a form that indicates the choice of agent (including route and dose) for an approved indication (eg, vancomycin for proven methicillin‐resistant Staphylococcus aureus bacteremia) and faxing this form to the hospital pharmacy. In other cases, approval requires a phone conversation with an Infectious Disease clinical fellow or a Clinical Pharmacist. None of these processes can be initiated or executed from within the CPOE system itself.
At our institution, the first dose of any restricted antimicrobial may be administered without going through the approval process if it is prescribed in the overnight hours defined as between 10 PM and 8 AM (Table 2). Retroactive approval is required the following day if the antimicrobial is to be continued.
Restricted Antimicrobial (eg, Vancomycin) | Unrestricted Antimicrobial (eg, Cefazolin) | |
---|---|---|
Daytime order (8 AM to 10 PM) | Approval required | No approval required |
Nighttime order (10 PM to 8 AM) | Exempted from approval for first dose (approval required during the next daytime period) | No approval required |
Data Acquisition
All antibiotic administration data were extracted electronically from the CPOE system. During the time of the study, not all hospital units utilized this system. Although CPOE was in place for all general medical wards, the intensive care units and many surgical services were not using CPOE at the time of the study. Based on limitations of the data entered into our CPOE system, many relevant clinical variables were not available for this study, such as clinical diagnoses or the level of care (eg, intensive care unit vs step‐down unit, vs regular nursing floor) at the time of the order.
Outcome Measures
We defined time‐to‐administration as the period of time between order entry and administration of the drug to the patient, as charted by the nurse in the CPOE system. However, nursing policy allows nurses to document the time of administration as the exact time of the order provided that the drug is delivered within 1 hour of a stat order, whereas all administration times beyond 1 hour of the order are expected to be documented precisely. This nursing policy eliminated our ability to determine whether antimicrobials were administered within 30 minutes of the order, and limited the value of examining time‐to‐administration as a continuous variable, but allowed us to reliably identify delays of >1 hour or >2 hours presuming that the nurse‐charted administration times were accurate. As our primary outcome, we defined delay in administration as when the antimicrobial was administered >1 hour from time of order. We examined delays of >2 hours as a secondary outcome. Antimicrobials ordered stat but not delivered within 4 hours of the order were excluded from analysis based on the recognition that extended delays might have resulted from changes in clinical circumstances or errors in documentation. Similarly, antimicrobials charted as being delivered prior to the order were excluded.
Statistical Analyses
We used the likelihood‐ratio chi‐square test to determine 2‐sided P values for differences between proportions. Logistic regression models were used to derive odds ratios (ORs) and to adjust for covariables. We adjusted for weekday versus weekend orders, patient characteristics including sex, age, white versus non‐white ethnicity and orders placed on medicine versus non‐medicine (eg, surgical or obstetrical) wards. Analyses were conducted in JMP 5.1 (SAS Institute, Cary, NC).
Results
In total, 3337 orders for stat antimicrobials were written during the study period, of which 86 (2.6%) were excluded based on being outside the specified 4‐hour window. This left a total of 3251 orders in 3251 discrete patients for analysis.
We found that a statistically significantly higher percentage of delays in antimicrobial administration when the antimicrobial was restricted as compared to unrestricted. This was the case for both our primary outcome of a >1 hour delay (Figure 1) and our secondary outcome of a delay of >2 hours (Figure 2). For restricted antimicrobial, delays of >1 hr occurred with 46.1% of orders during the day and with 38.8% of orders at night (when exempt from approval), P < 0.001. For unrestricted antimicrobials, delays of >1 hr occurred in 36.4% and 36.6% of instances, respectively (P = 0.57). The odds ratio for a delay in administration of 1 hour for restricted antimicrobials was 1.49 (95% CI = 1.23‐1.82).
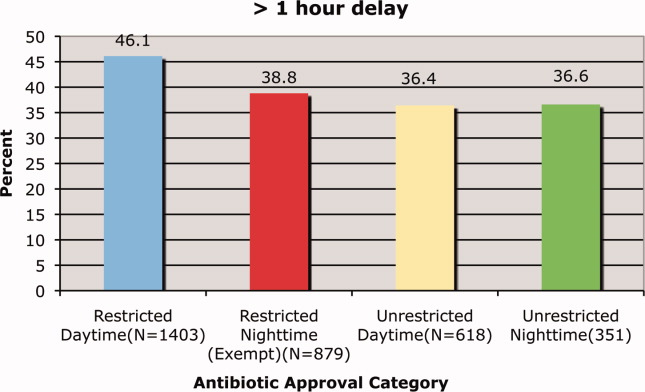
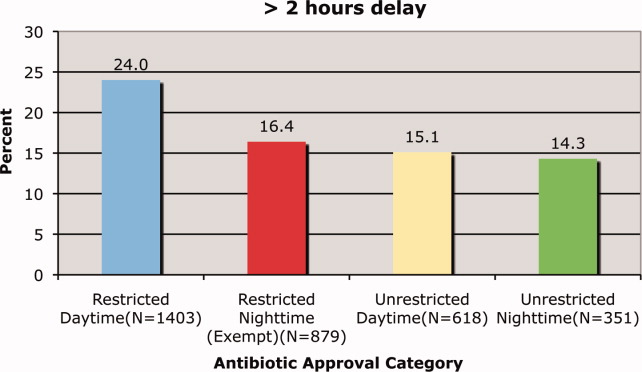
Delays beyond 2 hours occurred 24.0% of the time for restricted antimicrobials during the day versus 16.4% at night. Unrestricted antimicrobials were delayed >2 hrs only 15.1% and 14.3% of the time for day and night periods, respectively (P = 0.35). The odds ratio for a two‐hour or greater delay was 1.78 (95% CI = 1.39‐2.21), P < 0.0001 when the antimicrobial was restricted.
These odds ratios and statistical significance were unchanged by adjustment for primary service (medicine vs. non‐medicine), age, sex, ethnicity or whether the order was placed on a weekend or weekday (data not shown).
Discussion
We found that our institution's antimicrobial approval process was associated with statistically significant delays in the administration of antimicrobials that were ordered stat by the prescribing clinician. These delays were evident both when comparing restricted antimicrobials to unrestricted ones and when these restricted antimicrobials were compared to themselves during the overnight time period when they were temporarily exempt from the approval process. This suggests that the delay is associated with the approval process itself and not the specific drug or the time of day. We also found that over one‐third of all stat antimicrobial orders were not carried out in the within one hour. This rate approached nearly 50% for restricted drugs ordered stat. This high baseline rate for all stat‐ordered antimicrobials underscores system challenges that seem to be exacerbated when restricted antimicrobials are chosen.
We do not know if the delays we observed resulted in patient harm. Indeed it is possible, if not likely, that patient care at our institution is improved by the judicious use of certain antimicrobials, even if the process required to enforce their use may result in delayed antimicrobial administration in some instances. Since we did not collect clinical information on baseline diagnoses or severity of illness, and we did not have information on clinical outcomes, we cannot determine whether the clinical delays we observed might have caused harm. Determining the impact these approval policies have on patient outcomes would require a separate study designed to collect the necessary clinical data to answer that question.
An additional limitation was that we did not ascertain the indications for the antimicrobials to determine whether they truly needed to be given stat. We suspect that antimicrobials are sometimes ordered stat even when the infection being treated is not likely to be serious or life‐threatening. Additionally, since we relied on the nurse‐charted time of administration, it is possible that in some instances there was a charted delay in administration when in reality the patient received the antimicrobial in a timely fashion. In urgent situations, the nurses may be too busy to document that the medication was given until long after the dose is given, and this may result in inaccuracies in the charted administration time. However, this type of documentation error would be expected to affect restricted and unrestricted antimicrobials similarly and would be unlikely to result in a systematic bias.
Because we conducted this study at a single institution, the results may not be applicable to other medical institutions, especially since restriction policies and antimicrobial approval processes vary from hospital to hospital. The burden of delays may be related to the number of restricted antimicrobials on formulary, the types of antimicrobials restricted, the number of steps required to have them released from the pharmacy, whether the approval process is initiated from within the order entry system, and other factors that may streamline or hamper the approval process.
In our institution, there are several steps in the process, any of which might contribute to the delay. Faxed approval sheets may take time to arrive to and be acted upon by the pharmacy, errant pages may delay communication between the provider and the person providing approval, and there may be delays in the final approval being relayed to the pharmacy by the individual providing approval. In fact, an alternative explanation for the observed administration delays is that once ordered in CPOE, the prescribing physicians themselves are slow in initiating the approval process. While this is certainly possible, especially given the stresses surrounding the management of a seriously ill patient on the general ward, this still suggests that having to go through the approval process may impact the process of care.
Other possible explanations for the delays observed when the restrictive antimicrobial policy was in effect may include pharmacy staffing. Since the workload in the pharmacy would be expected to be greater during the day, when more patient care activity is occurring such as clinics and operating rooms, this increased workload may have slowed down the pharmacy filling the orders. However, such human resource‐workload imbalances would also be expected to slow most pharmacy processes and should lead to delays in filling the orders for other medications including the unrestricted antimicrobials. We did not track other non‐antimicrobial medications to examine their patterns of delay. Nursing workload also varies between day and night but the time period where the antimicrobial administration delays occurred is the time when nursing is favorably staffed unlike the night when nurse to patient ratios are low. It is possible that despite better nurse to patient ratios during the day, the workload‐to‐nursing ratio remains high and contributes to delays in administration of otherwise stat‐ordered antimicrobials. Again, it is unclear why this would disproportionately affect the restricted class of antimicrobials.
We do not advocate the abandonment of antimicrobial control policies. The process described here is very institution‐specific and while its benefits are proven, energy should be channeled where appropriate to facilitate this process. These policies are clearly necessary to help reduce costs, limit the unwarranted use of these drugs, and slow the proliferation of ever more resistant strains of microorganisms. However, we do advocate careful consideration of the components of the approval process itself, ensuring that delays in antimicrobial administration are kept to a minimum and are avoided altogether in critically ill patients. One way to accomplish this might be to not require approval for the first administration of a stat antibiotic, but to require approval for subsequent doses. Our institution's overnight exempt period data suggest that this would eliminate the incremental delays incurred by the approval process itself. As important, our results show that even for unrestricted antibiotics, we fall short of achieving recommended best practices, highlighting the challenges inherent to carrying out multi‐step clinical tasks in an efficient fashion.
In‐hospital administration of antimicrobials is often subject to controls and policies designed to limit the indiscriminant use of antimicrobials in situations where they are not warranted, to control costs, and to reduce the potential for the development of resistant microorganismsa major public health threat and patient safety concern. These controls and policies may include strategies such as limiting the choices of antimicrobials on formulary, rotational schedules of available antimicrobials, and antimicrobial approval processes.16 Antimicrobial approval processes commonly require that the bedside clinician obtain permission from a secondary source to administer a particular antimicrobial. This approval process may take the form of submitting written justification forms and/or direct telephone/fax requests to an Infectious Disease specialist, Clinical Pharmacist, or other surrogate prior to release of the antibiotic from the pharmacy. While these approval processes and other strategies have been shown to reduce the development of resistance,7, 8 improve outcomes,8 and provide education and revision of antimicrobial choice that may be more appropriate for the patient and suspected infection,9 they have the potential to delay the administration of the necessary antimicrobial by adding additional steps to the sequence of ordering, obtaining, and administering the medication. While it is certainly desirable to control the indiscriminant use of these medications, delays in antimicrobial administration may, in turn, worsen outcomes, thus counteracting the beneficial effects of control policies. The early and timely administration of appropriate broad spectrum antimicrobials chosen to cover the most expected organisms has been consistently shown to improve outcomes1027 and has been cited as an essential element of the Surviving Sepsis Campaign (
At our institution, the antimicrobial approval process requires written justification forms and/or a call or fax to an Infectious Disease specialist or Clinical Pharmacist prior to the antimicrobial being released from the pharmacy. We hypothesized that this process is associated with significant delays in patients receiving their prescribed antimicrobials when a restricted drug was chosen. The antibiotic approval process at our institution allows 1‐time stat doses of restricted antimicrobials to be ordered without preapproval at night (10 PM to 8 AM), but not during the day. This allowed us to compare the time‐to‐administration of restricted antimicrobials to their unrestricted counterparts and to themselves during this exempt time period.
Methods
Study Design
This was a single‐institution retrospective cohort study. We included all patients admitted to Johns Hopkins Hospital units utilizing the hospital's computerized provider order entry system (CPOE) who had stat orders placed for any of 24 preselected commonly ordered intravenous antimicrobials over a 1‐year period between November 1, 2005 and October 31, 2006. We did not include oral medications that were similarly subject to the approval process since we anticipated that the amount of time required to prepare the drug in the pharmacy and to deliver it to the patient would be systematically different than for intravenous medications. The CPOE system captures time of administration of all drugs (as charted by the nurse) in addition to the time of order entry by the provider. Institutional guidelines dictate that when drugs are ordered stat, they should be administered within 30 minutes of the order.
Classification of Antibiotics
Particular antimicrobials (24 different drugs) were chosen prospectively to include a broad range of commonly ordered drugs available at our hospital in intravenous form (Table 1). For this analysis, we only considered the first dose of the prescribed antimicrobial, since subsequent doses are not generally ordered stat and the timing of administration is clinically less important than the timing of the initial dose. Additionally, for patients who had multiple orders for stat antimicrobials during the course of hospitalization, we only included the first stat order in analysis.
Restricted Antimicrobials | Unrestricted Antimicrobials |
---|---|
Ampicillin/sulbactam | Acyclovir |
Azithromycin | Amphotericin |
Cefepime | Ampicillin |
Ciprofloxacin | Cefazolin |
Fluconazole | Ceftriaxone |
Gatifloxacin | Cephalexin |
Linezolid | Clindamycin |
Meropenem | Doxycycline |
Moxifloxacin | Ertapenem |
Vancomycin | Gentamicin |
Metronidazole | |
Oxacillin | |
Tobramycin | |
Trimethoprim/sulfamethoxazole |
For the purposes of the analysis, we defined antimicrobials as restricted or unrestricted. At our institution, restricted antimicrobials require either written or telephone approval from an Infectious Disease physician or Clinical Pharmacist. Obtaining written approval involves filling out a form that indicates the choice of agent (including route and dose) for an approved indication (eg, vancomycin for proven methicillin‐resistant Staphylococcus aureus bacteremia) and faxing this form to the hospital pharmacy. In other cases, approval requires a phone conversation with an Infectious Disease clinical fellow or a Clinical Pharmacist. None of these processes can be initiated or executed from within the CPOE system itself.
At our institution, the first dose of any restricted antimicrobial may be administered without going through the approval process if it is prescribed in the overnight hours defined as between 10 PM and 8 AM (Table 2). Retroactive approval is required the following day if the antimicrobial is to be continued.
Restricted Antimicrobial (eg, Vancomycin) | Unrestricted Antimicrobial (eg, Cefazolin) | |
---|---|---|
Daytime order (8 AM to 10 PM) | Approval required | No approval required |
Nighttime order (10 PM to 8 AM) | Exempted from approval for first dose (approval required during the next daytime period) | No approval required |
Data Acquisition
All antibiotic administration data were extracted electronically from the CPOE system. During the time of the study, not all hospital units utilized this system. Although CPOE was in place for all general medical wards, the intensive care units and many surgical services were not using CPOE at the time of the study. Based on limitations of the data entered into our CPOE system, many relevant clinical variables were not available for this study, such as clinical diagnoses or the level of care (eg, intensive care unit vs step‐down unit, vs regular nursing floor) at the time of the order.
Outcome Measures
We defined time‐to‐administration as the period of time between order entry and administration of the drug to the patient, as charted by the nurse in the CPOE system. However, nursing policy allows nurses to document the time of administration as the exact time of the order provided that the drug is delivered within 1 hour of a stat order, whereas all administration times beyond 1 hour of the order are expected to be documented precisely. This nursing policy eliminated our ability to determine whether antimicrobials were administered within 30 minutes of the order, and limited the value of examining time‐to‐administration as a continuous variable, but allowed us to reliably identify delays of >1 hour or >2 hours presuming that the nurse‐charted administration times were accurate. As our primary outcome, we defined delay in administration as when the antimicrobial was administered >1 hour from time of order. We examined delays of >2 hours as a secondary outcome. Antimicrobials ordered stat but not delivered within 4 hours of the order were excluded from analysis based on the recognition that extended delays might have resulted from changes in clinical circumstances or errors in documentation. Similarly, antimicrobials charted as being delivered prior to the order were excluded.
Statistical Analyses
We used the likelihood‐ratio chi‐square test to determine 2‐sided P values for differences between proportions. Logistic regression models were used to derive odds ratios (ORs) and to adjust for covariables. We adjusted for weekday versus weekend orders, patient characteristics including sex, age, white versus non‐white ethnicity and orders placed on medicine versus non‐medicine (eg, surgical or obstetrical) wards. Analyses were conducted in JMP 5.1 (SAS Institute, Cary, NC).
Results
In total, 3337 orders for stat antimicrobials were written during the study period, of which 86 (2.6%) were excluded based on being outside the specified 4‐hour window. This left a total of 3251 orders in 3251 discrete patients for analysis.
We found that a statistically significantly higher percentage of delays in antimicrobial administration when the antimicrobial was restricted as compared to unrestricted. This was the case for both our primary outcome of a >1 hour delay (Figure 1) and our secondary outcome of a delay of >2 hours (Figure 2). For restricted antimicrobial, delays of >1 hr occurred with 46.1% of orders during the day and with 38.8% of orders at night (when exempt from approval), P < 0.001. For unrestricted antimicrobials, delays of >1 hr occurred in 36.4% and 36.6% of instances, respectively (P = 0.57). The odds ratio for a delay in administration of 1 hour for restricted antimicrobials was 1.49 (95% CI = 1.23‐1.82).
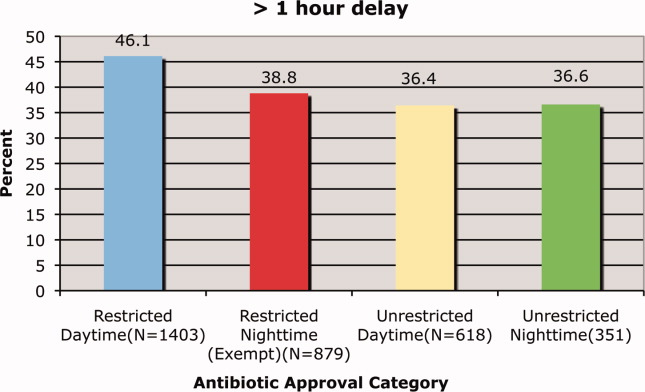
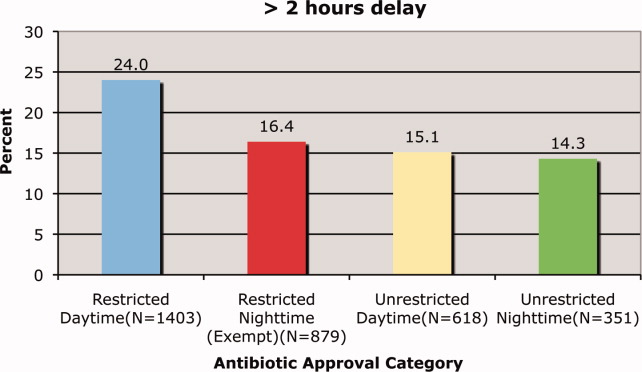
Delays beyond 2 hours occurred 24.0% of the time for restricted antimicrobials during the day versus 16.4% at night. Unrestricted antimicrobials were delayed >2 hrs only 15.1% and 14.3% of the time for day and night periods, respectively (P = 0.35). The odds ratio for a two‐hour or greater delay was 1.78 (95% CI = 1.39‐2.21), P < 0.0001 when the antimicrobial was restricted.
These odds ratios and statistical significance were unchanged by adjustment for primary service (medicine vs. non‐medicine), age, sex, ethnicity or whether the order was placed on a weekend or weekday (data not shown).
Discussion
We found that our institution's antimicrobial approval process was associated with statistically significant delays in the administration of antimicrobials that were ordered stat by the prescribing clinician. These delays were evident both when comparing restricted antimicrobials to unrestricted ones and when these restricted antimicrobials were compared to themselves during the overnight time period when they were temporarily exempt from the approval process. This suggests that the delay is associated with the approval process itself and not the specific drug or the time of day. We also found that over one‐third of all stat antimicrobial orders were not carried out in the within one hour. This rate approached nearly 50% for restricted drugs ordered stat. This high baseline rate for all stat‐ordered antimicrobials underscores system challenges that seem to be exacerbated when restricted antimicrobials are chosen.
We do not know if the delays we observed resulted in patient harm. Indeed it is possible, if not likely, that patient care at our institution is improved by the judicious use of certain antimicrobials, even if the process required to enforce their use may result in delayed antimicrobial administration in some instances. Since we did not collect clinical information on baseline diagnoses or severity of illness, and we did not have information on clinical outcomes, we cannot determine whether the clinical delays we observed might have caused harm. Determining the impact these approval policies have on patient outcomes would require a separate study designed to collect the necessary clinical data to answer that question.
An additional limitation was that we did not ascertain the indications for the antimicrobials to determine whether they truly needed to be given stat. We suspect that antimicrobials are sometimes ordered stat even when the infection being treated is not likely to be serious or life‐threatening. Additionally, since we relied on the nurse‐charted time of administration, it is possible that in some instances there was a charted delay in administration when in reality the patient received the antimicrobial in a timely fashion. In urgent situations, the nurses may be too busy to document that the medication was given until long after the dose is given, and this may result in inaccuracies in the charted administration time. However, this type of documentation error would be expected to affect restricted and unrestricted antimicrobials similarly and would be unlikely to result in a systematic bias.
Because we conducted this study at a single institution, the results may not be applicable to other medical institutions, especially since restriction policies and antimicrobial approval processes vary from hospital to hospital. The burden of delays may be related to the number of restricted antimicrobials on formulary, the types of antimicrobials restricted, the number of steps required to have them released from the pharmacy, whether the approval process is initiated from within the order entry system, and other factors that may streamline or hamper the approval process.
In our institution, there are several steps in the process, any of which might contribute to the delay. Faxed approval sheets may take time to arrive to and be acted upon by the pharmacy, errant pages may delay communication between the provider and the person providing approval, and there may be delays in the final approval being relayed to the pharmacy by the individual providing approval. In fact, an alternative explanation for the observed administration delays is that once ordered in CPOE, the prescribing physicians themselves are slow in initiating the approval process. While this is certainly possible, especially given the stresses surrounding the management of a seriously ill patient on the general ward, this still suggests that having to go through the approval process may impact the process of care.
Other possible explanations for the delays observed when the restrictive antimicrobial policy was in effect may include pharmacy staffing. Since the workload in the pharmacy would be expected to be greater during the day, when more patient care activity is occurring such as clinics and operating rooms, this increased workload may have slowed down the pharmacy filling the orders. However, such human resource‐workload imbalances would also be expected to slow most pharmacy processes and should lead to delays in filling the orders for other medications including the unrestricted antimicrobials. We did not track other non‐antimicrobial medications to examine their patterns of delay. Nursing workload also varies between day and night but the time period where the antimicrobial administration delays occurred is the time when nursing is favorably staffed unlike the night when nurse to patient ratios are low. It is possible that despite better nurse to patient ratios during the day, the workload‐to‐nursing ratio remains high and contributes to delays in administration of otherwise stat‐ordered antimicrobials. Again, it is unclear why this would disproportionately affect the restricted class of antimicrobials.
We do not advocate the abandonment of antimicrobial control policies. The process described here is very institution‐specific and while its benefits are proven, energy should be channeled where appropriate to facilitate this process. These policies are clearly necessary to help reduce costs, limit the unwarranted use of these drugs, and slow the proliferation of ever more resistant strains of microorganisms. However, we do advocate careful consideration of the components of the approval process itself, ensuring that delays in antimicrobial administration are kept to a minimum and are avoided altogether in critically ill patients. One way to accomplish this might be to not require approval for the first administration of a stat antibiotic, but to require approval for subsequent doses. Our institution's overnight exempt period data suggest that this would eliminate the incremental delays incurred by the approval process itself. As important, our results show that even for unrestricted antibiotics, we fall short of achieving recommended best practices, highlighting the challenges inherent to carrying out multi‐step clinical tasks in an efficient fashion.
- Antimicrobial stewardship.Am J Med.2006;119:S53–S61. .
- Restrictive antibiotic policies are appropriate in intensive care units.Crit Care Med.2003;31:S25–S28. .
- Effects of requiring prior authorization for selected anti‐microbials: expenditures, susceptibilities and clinical outcomes.Clin Infect Dis.1997;25:230–239. , , , et al.
- Class restriction of cephalosporin use to control total cephaslosporin resistance in nosocomial Klebsiella.JAMA.1998;280:1233–1237. , , , et al.
- Rotation and restricted use of antibiotics in a medical intensive care unit: impact on the incidence of ventilator associated pneumonia caused by antibiotic resistant Gram‐negative bacteremia.Am J Respir Crit Care Med.2001;63(3 Pt 1):837–843. , , , et al.
- Impact of rotation of empiric antibiotic schedules on infectious mortality in an intensive care unit.CCM.2001;29:1101–1108. , , , et al.
- Antimicrobial use control measures to prevent and control antimicrobial resistance in US hospitals.Infect Control Hosp Epidemiol.2006;27:1088–1095. , , , et al.
- Cost‐effectiveness of prospective and continuous parenteral antibiotic control: experience at the Palo Alto Veterans Affairs Medical Center from 1987 to 1989.Am J Med.1991;90:439–444. , , , , .
- Programmatic role of the infectious diseases physician in controlling antimicrobial costs in the hospital.Clin Infect Dis.1997;24:471–485. , .
- Antimicrobial stewardship and the role of pharmacokinetics–pharmacodynamics in the modern antibiotic era.Diagn Microbiol Infect Dis.2007;57(3 Suppl.):77S–83S. , .
- Improving inpatient antibiotic prescribing: insights from participation in a national collaborative.Jt Comm J Qual Improv.2001;27:387–402. , , , , , .
- Managing antibiotic resistance.N Engl J Med.2000;343:1961–1963. , .
- Antimicrobial stewardship programs in health care systems.Clin Microbiol Rev.2005;18(4):638–656. , .
- Interventions to improve antibiotic prescribing practices for hospital inpatients.Cochrane Database Syst Rev.2005;4:CD003543. , , , , , , , , , , .
- Evaluation of an antimicrobial stewardship program at a pediatric teaching hospital.Pediatr Infect Dis J.2008;27(2):106–111. , , , , .
- The utility of hospital antibiograms as tools for guiding empiric therapy and tracking resistance. Insights from the Society of Infectious Diseases Pharmacists.Pharmacotheray.2007;27(9):1306–1312. .
- Strategies to contain the emergence of antimicrobial resistance: a systematic review of effectiveness and cost‐effectiveness.J Health Serv Res Policy.2002;7(2):111–117. , , , .
- Infectious Diseases Society of America;Society for Healthcare Epidemiology of America.Infectious Diseases Society of America and the Society for Healthcare Epidemiology of America guidelines for developing an institutional program to enhance antimicrobial stewardship.Clin Infect Dis.2007;44(2):159–177. , , , , , , , , , , , , ;
- the Healthcare Infection Control Practices Advisory Committee. Management of Multi‐Drug Reistsant Organisms in Healthcare Settings: 2006. Available at: http://www.cdc.gov/ncidod/dhqp/pdf/ar/MDROGuideline2006.pdf. Accessed May 19,2008. , , , , and
- Gram‐negative bacteremia. IV Re‐evaluation of clinical features and treatment in 612 patients.Am J Med.1980;68:344–355. , , .
- Gram‐negative bacteremia. III. Reassessment of etiology, epidemiology and ecology in 612 patients.Am J Med.1980;68:332–343. , , , .
- Inadequate antimicrobial treatment of infections: a risk factor for hospital mortality among critically ill patients.Chest.1999;115:462–474. , , , .
- Modification of empiric antibiotic treatment in patients with pneumonia acquired in the intensive care unit. ICU‐Acquired Pneumonia Study Group.Intensive Care Med.1996;22:387–394. .
- The value of routine microbial investigation in ventilator‐associated pneumonia.Am J Respir Crit Care Med.1997;156:196–200. , , , , .
- Impact of BAL data on the therapy and outcome of ventilator‐associated pneumonia.Chest.1997;111:676–685. , , , et al.
- The influence of mini‐BAL cultures on patient outcomes: implications for the antibiotic management of ventilator associated pneumonia.Chest.1998;113:412–420. , .
- The influence of inadequate antimicrobial treatment of bloodstream infections on patient outcomes in the ICU setting.Chest.2000;118:146–155. , , , , .
- Antimicrobial stewardship.Am J Med.2006;119:S53–S61. .
- Restrictive antibiotic policies are appropriate in intensive care units.Crit Care Med.2003;31:S25–S28. .
- Effects of requiring prior authorization for selected anti‐microbials: expenditures, susceptibilities and clinical outcomes.Clin Infect Dis.1997;25:230–239. , , , et al.
- Class restriction of cephalosporin use to control total cephaslosporin resistance in nosocomial Klebsiella.JAMA.1998;280:1233–1237. , , , et al.
- Rotation and restricted use of antibiotics in a medical intensive care unit: impact on the incidence of ventilator associated pneumonia caused by antibiotic resistant Gram‐negative bacteremia.Am J Respir Crit Care Med.2001;63(3 Pt 1):837–843. , , , et al.
- Impact of rotation of empiric antibiotic schedules on infectious mortality in an intensive care unit.CCM.2001;29:1101–1108. , , , et al.
- Antimicrobial use control measures to prevent and control antimicrobial resistance in US hospitals.Infect Control Hosp Epidemiol.2006;27:1088–1095. , , , et al.
- Cost‐effectiveness of prospective and continuous parenteral antibiotic control: experience at the Palo Alto Veterans Affairs Medical Center from 1987 to 1989.Am J Med.1991;90:439–444. , , , , .
- Programmatic role of the infectious diseases physician in controlling antimicrobial costs in the hospital.Clin Infect Dis.1997;24:471–485. , .
- Antimicrobial stewardship and the role of pharmacokinetics–pharmacodynamics in the modern antibiotic era.Diagn Microbiol Infect Dis.2007;57(3 Suppl.):77S–83S. , .
- Improving inpatient antibiotic prescribing: insights from participation in a national collaborative.Jt Comm J Qual Improv.2001;27:387–402. , , , , , .
- Managing antibiotic resistance.N Engl J Med.2000;343:1961–1963. , .
- Antimicrobial stewardship programs in health care systems.Clin Microbiol Rev.2005;18(4):638–656. , .
- Interventions to improve antibiotic prescribing practices for hospital inpatients.Cochrane Database Syst Rev.2005;4:CD003543. , , , , , , , , , , .
- Evaluation of an antimicrobial stewardship program at a pediatric teaching hospital.Pediatr Infect Dis J.2008;27(2):106–111. , , , , .
- The utility of hospital antibiograms as tools for guiding empiric therapy and tracking resistance. Insights from the Society of Infectious Diseases Pharmacists.Pharmacotheray.2007;27(9):1306–1312. .
- Strategies to contain the emergence of antimicrobial resistance: a systematic review of effectiveness and cost‐effectiveness.J Health Serv Res Policy.2002;7(2):111–117. , , , .
- Infectious Diseases Society of America;Society for Healthcare Epidemiology of America.Infectious Diseases Society of America and the Society for Healthcare Epidemiology of America guidelines for developing an institutional program to enhance antimicrobial stewardship.Clin Infect Dis.2007;44(2):159–177. , , , , , , , , , , , , ;
- the Healthcare Infection Control Practices Advisory Committee. Management of Multi‐Drug Reistsant Organisms in Healthcare Settings: 2006. Available at: http://www.cdc.gov/ncidod/dhqp/pdf/ar/MDROGuideline2006.pdf. Accessed May 19,2008. , , , , and
- Gram‐negative bacteremia. IV Re‐evaluation of clinical features and treatment in 612 patients.Am J Med.1980;68:344–355. , , .
- Gram‐negative bacteremia. III. Reassessment of etiology, epidemiology and ecology in 612 patients.Am J Med.1980;68:332–343. , , , .
- Inadequate antimicrobial treatment of infections: a risk factor for hospital mortality among critically ill patients.Chest.1999;115:462–474. , , , .
- Modification of empiric antibiotic treatment in patients with pneumonia acquired in the intensive care unit. ICU‐Acquired Pneumonia Study Group.Intensive Care Med.1996;22:387–394. .
- The value of routine microbial investigation in ventilator‐associated pneumonia.Am J Respir Crit Care Med.1997;156:196–200. , , , , .
- Impact of BAL data on the therapy and outcome of ventilator‐associated pneumonia.Chest.1997;111:676–685. , , , et al.
- The influence of mini‐BAL cultures on patient outcomes: implications for the antibiotic management of ventilator associated pneumonia.Chest.1998;113:412–420. , .
- The influence of inadequate antimicrobial treatment of bloodstream infections on patient outcomes in the ICU setting.Chest.2000;118:146–155. , , , , .
Copyright © 2010 Society of Hospital Medicine