User login
Ultrasound and Pleural Effusions
Hospitalists commonly encounter pleural effusions, and their detection and characterization by point‐of‐care ultrasound can guide management. Approximately 44% to 57% of hospitalized patients with bacterial pneumonia,[1, 2] and up to 62% of intensive care unit (ICU) patients[3] have a pleural effusion. For patients with a parapneumonic effusion, hospitalists can use ultrasound to quantify and characterize pleural fluid to determine whether diagnostic or therapeutic drainage is indicated, as well as guide performance of thoracentesis. For patients with lung cancer, detection of a malignant pleural effusion changes staging to stage IV, regardless of tumor size or lymph node involvement, and hospitalists may discuss more appropriate treatment options with patients and consultants.
Routine use of pleural ultrasonography may help hospitalists provide high‐value care by reducing ancillary testing, including computerized tomography (CT) scans that expose patients to ionizing radiation, and reducing complications of thoracentesis. However, many hospitalists may not be familiar with the use of point‐of‐care ultrasound. A national survey in 2012 revealed only 25% of internal medicine residencies have formal curricula to teach point‐of‐care ultrasonography.[4] The purpose of this review is to provide an overview of how point‐of‐care ultrasound can be utilized by hospitalists in the care of patients with pleural effusions. We review the literature on the diagnosis and evaluation of pleural effusions with ultrasound, as well as techniques to examine and drain the pleural space.
DIAGNOSIS OF PLEURAL EFFUSION
History and Physical Exam
Pleural effusions are most commonly associated with heart failure, pneumonia, cancer, pulmonary embolism, viral disease, coronary artery bypass surgery, and cirrhosis with ascites. The most common symptoms related to pleural effusion are nonspecific and often indistinguishable from those of the underlying disease process, including cough, dyspnea, and pleuritic chest pain.[5]
Diagnostic accuracy of a physical examination to detect pleural fluid is highly dependent on the size of the effusion and is unlikely to detect effusions <300 mL. A systematic review found the most accurate physical exam findings to rule in a pleural effusion were dullness to percussion (positive likelihood ratio [LR]: 8.7; 95% CI: 2.2‐33.8) and asymmetric chest expansion (positive LR: 8.1; 95% CI: 5.2‐12.7). Normal tactile vocal fremitus was the most accurate physical exam finding to rule out a pleural effusion (negative LR: 0.21; 95% CI: 0.12‐0.37).[6] A major limitation of all these studies is that physical exam was compared to chest radiography as the reference standard, and posterior‐anterior chest radiographs are not sensitive for detection of pleural effusions until 200 mL of fluid has accumulated.[7] Further, chest percussion penetrates to a maximum depth of 6 cm, and its utility is limited in obese patients.[8] Characteristics of pleural fluid that can change management, such as loculations, cannot be detected by physical exam.
Chest Radiography
Chest radiography has traditionally been used to diagnose pleural effusions. Free‐flowing pleural fluid collects in the most dependent portions of the thorax, initially in the subpulmonic space followed by the costophrenic recesses. Pleural fluid is detectable in the costophrenic recesses on lateral upright chest radiograph after 50 mL has accumulated. On standard posterior‐anterior chest radiograph, blunting of the costophrenic recesses and obliteration of the hemidiaphragm are seen when >200 mL and >500 mL of pleural fluid have accumulated, respectively.[7] However, upright chest radiographs can miss a considerable number of effusions, including as many as 10% of parapneumonic effusions large enough to indicate need for drainage.[9] Supine anterior‐posterior chest radiographs can miss a significant proportion of large effusions seen on chest CT,[10] ultrasound,[11] and lateral decubitus radiographs.[12] Pleural effusions are frequently mistaken for parenchymal opacities on portable anterior‐posterior chest radiographs.[10]
Computerized Tomography
Chest CT serves as the reference standard in most modern diagnostic accuracy studies. Limitations of chest CT include difficulty distinguishing small effusions from pleural thickening, dependent atelectasis, or tumor; lower sensitivity for detecting pleural fluid septations compared to ultrasound[13]; exposure of patients to approximately 7 mSv of ionizing radiation (the equivalent radiation dose of 350 chest radiographs)[14]; high cost; and need to transport patients to radiology departments where CT scanners are located.
Pleural Ultrasonography
Ultrasound can rapidly differentiate conditions that demonstrate a nonspecific, radiopaque appearance of lower lung fields on chest radiographs, including pleural effusions, pneumonia, atelectasis, elevated hemidiaphragm, and lung or pleural masses. Advantages of point‐of‐care ultrasound include the ability of hospitalists to acquire and interpret images at the bedside and integrate findings into clinical decision making immediately. Multiple studies have demonstrated superior diagnostic accuracy of ultrasound compared to chest radiography for detection of pleural effusions. Pleural ultrasound can detect physiologic amounts of pleural fluid (5 mL),[15] but a minimal volume of 20 mL is more reliably detected,[16] and ultrasound is 100% sensitive for effusions >100 mL.[17] In a prospective study of critically ill patients with acute respiratory distress syndrome, the diagnostic accuracy of ultrasound for pleural effusions was superior (93%) compared to auscultation (61%) and anterior‐posterior chest radiograph (47%), using chest CT as the reference standard.[18] A meta‐analysis of 4 studies calculated a pooled sensitivity and specificity of ultrasound for detection of pleural effusions as 93% (95% CI: 89%‐96%) and 96% (95% CI: 95%‐98%), respectively.[18, 19, 20, 21, 22] Ultrasound has the additional benefit of characterizing underlying lung parenchyma, which is well described in the literature but beyond the scope of this review.[23]
Sensitivity and specificity of chest radiography and ultrasonography to detect a pleural effusion are displayed in Table 1.[9, 10, 11, 12, 18, 20, 21, 22, 24, 25, 26]
Exam | Reference Standard | Sensitivity | Specificity | Study | |
---|---|---|---|---|---|
| |||||
Chest radiograph | Supine AP | Upright PA/lateral | 92% | Woodring[24] | |
Lateral decubitus XR | 67% | 70% | Ruskin[12] | ||
Ultrasound | 82% | 82% | Emamian[11] | ||
Ultrasound or thoracentesis | 33% | Kocijancic[25] | |||
CT | 39% | 85% | Lichtenstein[18] | ||
CT | 66% | 89% | Kitazano[10] | ||
CT | 65% | 81% | Xirouchaki[26] | ||
CT | 78% | 76% | Brixey[9] | ||
Lateral decubitus | Ultrasound or thoracentesis | 94% | 100% | Kocijancic[25] | |
Upright PA | CT | 82% | 81% | Brixey[9] | |
Lateral upright | CT | 86% | 88% | Brixey[9] | |
Ultrasound | Cardiology | CT | 93% | 88% | Kataoka[20] |
Point of care | CT or tube thoracostomy | 96% | 100% | Ma[21] | |
CT | 92% | 93% | Lichtenstein[18] | ||
CT | 94% | 99% | Rocco[22] | ||
CT | 100% | 100% | Xirouchaki[26] |
PLEURAL ULTRASOUND EXAMINATION
A pleural ultrasound exam may be performed as part of a complete lung ultrasound exam, such as the BLUE (Bedside Lung Ultrasound in Emergency) protocol,[27] or a focused exam to evaluate a suspected or known pleural effusion seen on chest radiograph or CT scan.[27] Free‐flowing pleural effusions accumulate in the most dependent portions of the thorax, most commonly, the posterolateral costophrenic recesses in semirecumbent or seated patients, but anteriorly in mechanically ventilated patients in a prone position.
A low‐frequency (25 MHz) phased‐array transducer is generally preferred for imaging in between the ribs. High‐frequency linear transducers do not provide adequate penetration to visualize deep structures, but do provide superior visualization of the pleural line to assess pleural thickness, measure pleural depth, and evaluate for pneumothorax.
Pleural effusions are best evaluated starting at the level of the diaphragm. Place the transducer in a longitudinal plane on the posterior axillary line at the level of the diaphragm with the transducer orientation marker (notch) pointed cephalad (Figure 1). Five structures must be definitively identified to diagnose a pleural effusion: liver/spleen, diaphragm, pleural fluid, lung, and chest wall (Figure 2A). Large pleural effusions compress the adjacent lung causing atelectasis, which gives the lung a tissue‐like echogenicity similar to the liver (Figure 2B). Static air bronchograms are commonly seen in atelectatic lung bases with pleural effusions.[28]
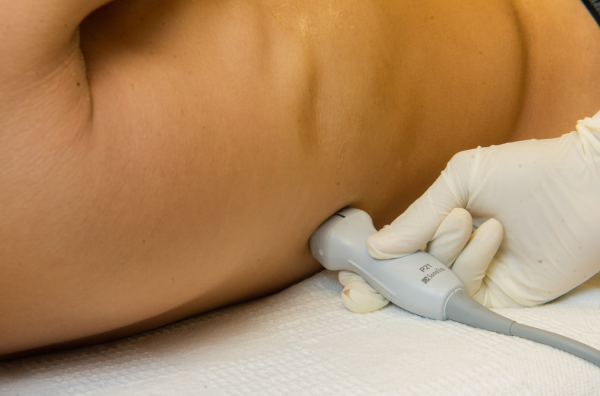
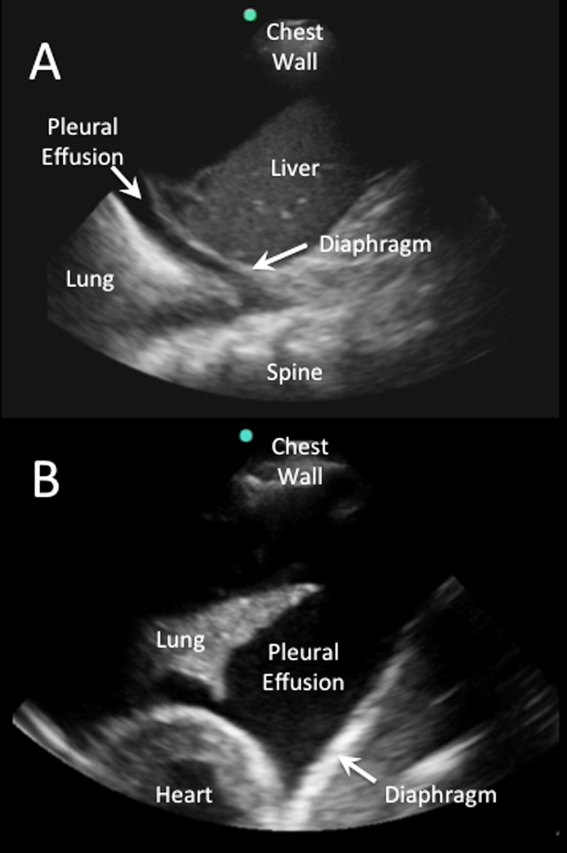
Color flow Doppler and M‐mode ultrasound may be utilized as adjuncts to routine 2‐dimensional ultrasonography. Free‐flowing pleural effusions will demonstrate flow by color Doppler (Figure 3A). Using M‐mode ultrasound, the lung can been seen moving within a pleural effusion to and from the chest wall (sinusoid sign).[29] Absence of flow or movement is seen with dense pleural loculations, pleural thickening, and peripheral lung or pleural masses (Figure 3B).
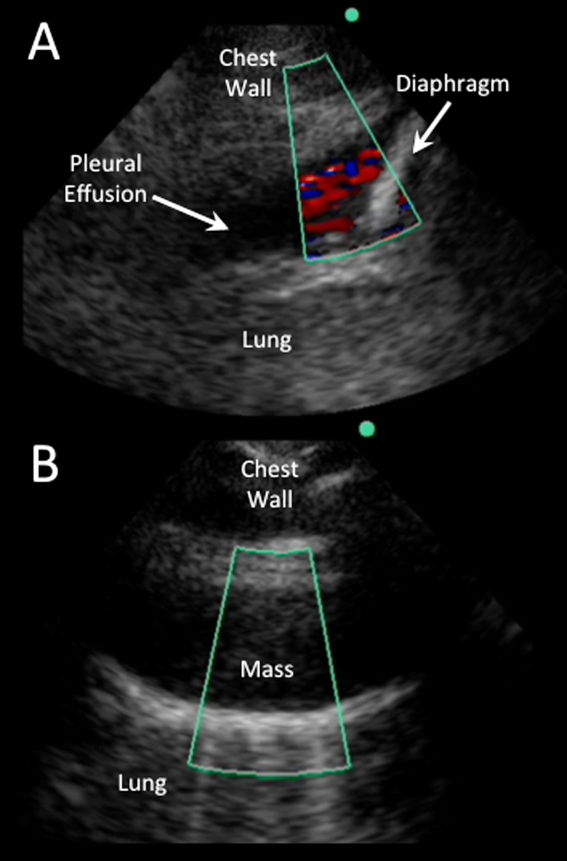
CHARACTERIZATION OF PLEURAL EFFUSION
Pleural Fluid Volume
Quantification of pleural fluid volume has been proposed using formulas with sonographic measurements.[30, 31, 32] These formulas are most accurate for moderate‐sized effusions but have not been validated beyond individual study cohorts. The largest study (n = 150) found a strong correlation between calculated and actual volumes drained by thoracentesis (r2 = 0.79; P < 0.001) using the formula (Volume [mL] = 16 parietal to visceral pleura distance (mm) at the mid‐diaphragm).[31] Although an accurate quantitative pleural fluid volume assessment may be possible, these formulas are not commonly used in clinical practice. A qualitative assessment is adequate for most clinical decision making using categories of minimal, small, moderate, or large volume.
Simple Versus Complex Effusions
Based on its sonographic appearance, pleural effusions are categorized as simple or complex. Simple pleural effusions are anechoic and usually transudative. Complex pleural effusions are subcategorized as homogeneously or heterogeneously echogenic, with or without septations, and are more often exudative.[33]
Effusions with heterogeneous echogenicity with swirling echoes suggest high cellular content that is often associated with malignancy.[34] Fibrinous stranding, septations, and loculations also suggest an exudative effusion (Figure 4A), and are more readily identified and characterized on lung ultrasound than CT scan.[35]
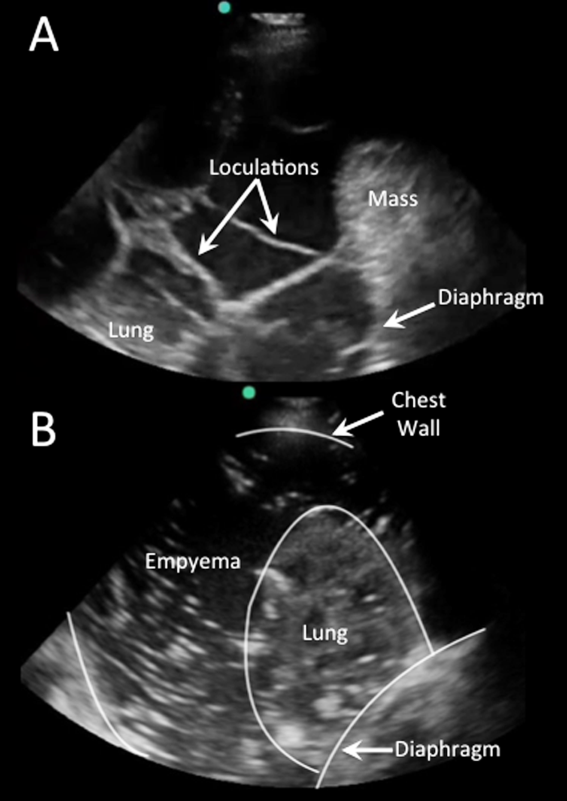
Homogenously echogenic effusions are most often due to hemothorax or empyema.[36] The high cell count of a hemothorax creates a layering effect in costophrenic recesses (hematocrit sign). Empyemas develop from complex effusions that organize into collections of pus and usually have a homogeneously echogenic, speckled appearance (Figure 4B). Sonographic evidence of septations in the presence of empyema predicts the need for intrapleural fibrinolytic therapy, longer duration of drainage, and possible surgical intervention.[37]
Isolated dense loculations may be challenging to differentiate from peripheral lung or pleural lesions, such as abscess or tumor.
Pleural Thickness
Normal visceral and parietal pleura are apposed and 0.2 to 0.3 mm thick.[38] Pleural effusions with parietal pleural thickness >10 mm, pleural nodularity, and diaphragmatic thickness >7 mm predicted underlying malignancy with high specificity and positive predictive value in 1 study.[39] As many as 20% of anechoic lesions of the pleura are solid rather than fluid. Color flow Doppler ultrasound can differentiate small pleural effusions from solid pleural abnormalities with sensitivity and specificity of 89% and 100%, respectively.[40]
PLEURAL FLUID DRAINAGE
Since its first description in 1967, use of ultrasound guidance for thoracentesis has evolved to become the standard of care in many hospitals in the United States.[41] The British Thoracic Society guidelines recommend that all thoracenteses be performed with ultrasound guidance.[42] The American College of Graduate Medical Education now requires proficiency in the use of ultrasound for thoracentesis and pleural catheter insertion by pulmonary and critical care fellows.[43]
The impetus for these recommendations stems from increased procedural success and decreased complications associated with ultrasound‐guided drainage of pleural effusions. A study evaluating thoracentesis site selection based on physical exam and chest radiographs demonstrated inaccurate site selection in 15% of patients, and use of ultrasound for site selection prevented possible accidental organ puncture in 10% of all cases.[44] The success rate of thoracentesis for small pleural effusions has been shown to increase from 66% to 90% with ultrasound guidance.[42] Using ultrasound, the distance from the skin to parietal and visceral pleura can be measured to determine whether thoracentesis can be safely performed, and to guide selection of an adequate length needle (Figure 5). A minimum pleural effusion depth of 1.5 cm between the visceral and parietal pleura has been recommended to perform diagnostic thoracentesis.[28] Diagnostic thoracentesis of complex septated pleural effusions or empyemas may be performed with a straight needle, but therapeutic drainage often requires temporary insertion of a catheter. Traditionally, large‐bore chest tubes (>24 F) had been advocated to drain viscid pus, but recent evidence suggests that small‐bore catheters (1014 F) with instillation of thrombolytics may be as effective and performed with less discomfort.[45] Video‐assisted thoracoscopy to lyse septations and evacuate infected materials is indicated when chest tube drainage has failed.
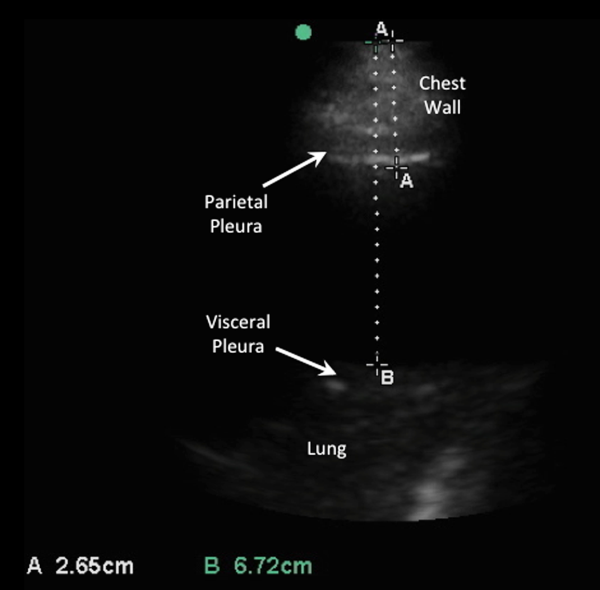
The most common complication of pleural drainage is pneumothorax. A meta‐analysis demonstrated a reduction in post‐thoracentesis pneumothorax rates from 9% to 4% with use of ultrasound.[46] Transporting patients to radiology for ultrasound marking has not been shown to decrease pneumothorax rates compared to thoracentesis without ultrasound guidance, likely due to changes in patient position and prolonged delays between marking and drainage.[47] Postprocedure pneumothorax can be ruled out if lung sliding is visualized. A meta‐analysis demonstrated superior sensitivity and similar specificity of pleural ultrasonography versus chest radiography to detect pneumothorax (sensitivity 91% vs 50% and specificity 98% vs 99%, respectively).[48] Real‐time ultrasound guidance for thoracentesis, or use of ultrasound to track the needle tip, has not been well studied but may be performed by experienced proceduralists to drain small effusions.
FUTURE RESEARCH
Future research should focus on the clinical effectiveness of point‐of‐care pleural ultrasonography when integrated with other diagnostic tools, and application of new ultrasound technologies to evaluate pleural diseases. Routine use of point‐of‐care ultrasound as the primary imaging modality in a medical ICU demonstrated a highly statistically significant reduction in chest x‐rays (3.75 vs 0.82, P < 0.0001) and chest CT scans (0.10 vs 0.04, P = 0.0007).[49] Similar studies have yet to be performed with the use of ultrasound specifically in the management of pleural diseases. Thus, clinical effectiveness studies are needed to assess the impact of routine use of pleural ultrasound on the initiation of appropriate therapies, length of stay, and costs in the management of pleural disease.
Point‐of‐care pleural ultrasound findings need to be evaluated in the context of other clinical findings and diagnostic tests. Certain ultrasound findings have been associated with exudative pleural effusions, but whether exudative and transudative effusions can be differentiated noninvasively using ultrasound findings alone, or in combination with other clinical data, warrants investigation. Similar to severity of illness scores, models that incorporate clinical, laboratory, and ultrasound findings need to be developed to guide treatment decisions, such as type of drainage procedure, as well as prognostication.
Finally, new technologies may advance the utility of point‐of‐care pleural ultrasonography. Even though 3‐dimensional ultrasonography has been available for over 2 decades, comparative studies of conventional 2‐dimensional versus 3‐dimensional ultrasonography to characterize pleural effusions have yet to be performed. Furthermore, computer‐aided detection has been shown to facilitate interpretation of ultrasound images, but this technology has yet to be applied to pleural ultrasonography.
CONCLUSIONS
Point‐of‐care pleural ultrasound is a powerful bedside tool in the hospitalist's armamentarium that is superior to physical examination and chest radiographs in the detection and characterization of pleural effusions. Furthermore, ultrasound performs similarly when compared to CT scans but offers the advantages of decreased cost, avoidance of ionizing radiation, and availability at the bedside. Ultrasound guidance reduces complications and increases the success rate of pleural drainage procedures, leading to improved patient safety. As clinical effectiveness studies emerge revealing its true value, point‐of‐care pleural ultrasonography is likely to become the standard diagnostic tool to evaluate and manage patients with pleural effusions.
Disclosures: Dr. Restrepo is partially supported by award number K23HL096054 from the National Heart, Lung, and Blood Institute. The content is solely the responsibility of the authors and does not necessarily represent the official views of the National Heart, Lung, and Blood Institute or the National Institutes of Health. The authors report no conflicts of interest.
- Parapneumonic effusions. Am J Med. 1980;69(4):507–512. , , , .
- The incidence and clinical correlates of parapneumonic effusions in pneumococcal pneumonia. Chest. 1978;74(2):170–173. , , .
- Pleural effusions in the medical ICU: prevalence, causes, and clinical implications. Chest. 1997;111(4):1018–1023. , , , , .
- Point‐of‐care ultrasound in internal medicine: a national survey of educational leadership. J Grad Med Educ. 2013;5(3):498–502. , , , .
- Pleural Diseases. Philadelphia, PA: Lippincott Williams 2007. .
- Does this patient have a pleural effusion? JAMA. 2009;301(3):309–317. , , .
- Pleural fluid volume estimation: a chest radiograph prediction rule. Acad Radiol. 1996;3(2):103–109. , , , .
- Accuracy of the physical examination in evaluating pleural effusion. Cleve Clin J Med. 2008;75(4):297–303. , .
- The efficacy of chest radiographs in detecting parapneumonic effusions. Respirology. 2011;16(6):1000–1004. , , , , .
- Differentiation of pleural effusions from parenchymal opacities: accuracy of bedside chest radiography. AJR Am J Roentgenol. 2010;194(2):407–412. , , , , .
- Accuracy of the diagnosis of pleural effusion on supine chest X‐ray. Eur Radiol. 1997;7(1):57–60. , , , .
- Detection of pleural effusions on supine chest radiographs. AJR Am J Roentgenol. 1987;148(4):681–683. , , , .
- Multiloculated pleural effusion detected by ultrasound only in a critically‐ill patient. Am J Case Rep. 2013;14:63–66. , , , , .
- Exposure to low‐dose ionizing radiation from medical imaging procedures. N Engl J Med. 2009;361(9):849–857. , , , et al.
- The diagnosis of pleural effusion by ultrasonic and radiologic techniques. Chest. 1976;70(1):33–37. , , .
- Ultrasound in blunt abdominal and thoracic trauma. J Trauma. 1993;34(4):488–495. , , , , , .
- Application of color Doppler ultrasound in the study of small pleural effusion. Med Ultrason. 2010;12(1):12–16. , , , et al.
- Comparative diagnostic performances of auscultation, chest radiography, and lung ultrasonography in acute respiratory distress syndrome. Anesthesiology. 2004;100(1):9–15. , , , , , .
- Diagnostic accuracy of sonography for pleural effusion: systematic review. Sao Paulo Med J. 2010;128(2):90–95. , , , , .
- The role of thoracic ultrasonography for evaluation of patients with decompensated chronic heart failure. J Am Coll Cardiol. 2000;35(6):1638–1646. , .
- Trauma ultrasound examination versus chest radiography in the detection of hemothorax. Ann Emerg Med. 1997;29(3):312–315; discussion 315–316. , .
- Diagnostic accuracy of bedside ultrasonography in the ICU: feasibility of detecting pulmonary effusion and lung contusion in patients on respiratory support after severe blunt thoracic trauma. Acta Anaesthesiol Scand. 2008;52(6):776–784. , , , et al.
- Lung ultrasound in the critically ill. Ann Intensive Care. 2014;4(1):1. .
- Recognition of pleural effusion on supine radiographs: how much fluid is required? AJR. Am J Roentgenol. 1984;142(1):59–64. .
- Chest sonography versus lateral decubitus radiography in the diagnosis of small pleural effusions. J Clin Ultrasound. 2003;31(2):69–74. , , .
- Impact of lung ultrasound on clinical decision making in critically ill patients. Intensive Care Med. 2014;40(1):57–65. , , , , .
- Lung ultrasound in acute respiratory failure an introduction to the BLUE‐protocol. Minerva Anestesiol. 2009;75(5):313–317. .
- Point‐of‐Care Ultrasound. 1st ed. Philadelphia, PA: Saunders; 2014. , , .
- International evidence‐based recommendations for point‐of‐care lung ultrasound. Intensive Care Med. 2012;38(4):577–591. , , , et al.
- Ultrasound estimation of volume of pleural fluid in mechanically ventilated patients. Intensive Care Med. 2006;32(2):318–321. , , , et al.
- Ultrasound estimation of volume of postoperative pleural effusion in cardiac surgery patients. Interact Cardiovasc Thorac Surg. 2010;10(2):204–207. , , .
- Multiplane ultrasound approach to quantify pleural effusion at the bedside. Intensive Care Med. 2010;36(4):656–664. , , , et al.
- Value of sonography in determining the nature of pleural effusion: analysis of 320 cases. AJR Am J Roentgenol. 1992;159(1):29–33. , , , , , .
- Echogenic swirling pattern as a predictor of malignant pleural effusions in patients with malignancies. Chest. 2004;126(1):129–134. , , , , , .
- Imaging the pleura: sonography, CT, and MR imaging. AJR Am J Roentgenol. 1991;156(6):1145–1153. , .
- Pleural effusions in febrile medical ICU patients: chest ultrasound study. Chest. 2004;126(4):1274–1280. , , , et al.
- Sonographic septation: a useful prognostic indicator of acute thoracic empyema. J Ultrasound Med. 2000;19(12):837–843. , , , , .
- Sonography of the pleura [in German]. Ultraschall Med. 2010;31(1):8–22, quiz 23–25. .
- Thoracic ultrasound in the diagnosis of malignant pleural effusion. Thorax. 2009;64(2):139–143. , , .
- “Fluid color” sign: a useful indicator for discrimination between pleural thickening and pleural effusion. J Ultrasound Med. 1995;14(10):767–769. , , , .
- Reflected ultrasound in the detection and localization of pleural effusion. JAMA. 1967;200(5):399–402. , , .
- Pleural procedures and thoracic ultrasound: British Thoracic Society Pleural Disease Guideline 2010. Thorax. 2010;65(suppl 2):ii61–ii76. , , , .
- Accreditation Council for Graduate Medical Education. http://www.acgme.org/acgmeweb. Accessed January 15, 2015.
- Accuracy of pleural puncture sites: a prospective comparison of clinical examination with ultrasound. Chest. 2003;123(2):436–441. , , .
- A double blind randomized cross over trial comparing rate of decortication and efficacy of intrapleural instillation of alteplase vs placebo in patients with empyemas and complicated parapneumonic effusions. Respir Med. 2012;106(5):716–723. , , , , , .
- Pneumothorax following thoracentesis: a systematic review and meta‐analysis. Arch Intern Med. 2010;170(4):332–339. , , , .
- Factors affecting the development of pneumothorax associated with thoracentesis. AJR Am J Roentgenol. 1991;156(5):917–920. , , , , , .
- Test characteristics of ultrasonography for the detection of pneumothorax: a systematic review and meta‐analysis. Chest. 2012;141(3):703–708. , , .
- The effect of point‐of‐care ultrasonography on imaging studies in the medical ICU: a comparative study. Chest. 2014;146(6):1574–1577. , , , et al.
Hospitalists commonly encounter pleural effusions, and their detection and characterization by point‐of‐care ultrasound can guide management. Approximately 44% to 57% of hospitalized patients with bacterial pneumonia,[1, 2] and up to 62% of intensive care unit (ICU) patients[3] have a pleural effusion. For patients with a parapneumonic effusion, hospitalists can use ultrasound to quantify and characterize pleural fluid to determine whether diagnostic or therapeutic drainage is indicated, as well as guide performance of thoracentesis. For patients with lung cancer, detection of a malignant pleural effusion changes staging to stage IV, regardless of tumor size or lymph node involvement, and hospitalists may discuss more appropriate treatment options with patients and consultants.
Routine use of pleural ultrasonography may help hospitalists provide high‐value care by reducing ancillary testing, including computerized tomography (CT) scans that expose patients to ionizing radiation, and reducing complications of thoracentesis. However, many hospitalists may not be familiar with the use of point‐of‐care ultrasound. A national survey in 2012 revealed only 25% of internal medicine residencies have formal curricula to teach point‐of‐care ultrasonography.[4] The purpose of this review is to provide an overview of how point‐of‐care ultrasound can be utilized by hospitalists in the care of patients with pleural effusions. We review the literature on the diagnosis and evaluation of pleural effusions with ultrasound, as well as techniques to examine and drain the pleural space.
DIAGNOSIS OF PLEURAL EFFUSION
History and Physical Exam
Pleural effusions are most commonly associated with heart failure, pneumonia, cancer, pulmonary embolism, viral disease, coronary artery bypass surgery, and cirrhosis with ascites. The most common symptoms related to pleural effusion are nonspecific and often indistinguishable from those of the underlying disease process, including cough, dyspnea, and pleuritic chest pain.[5]
Diagnostic accuracy of a physical examination to detect pleural fluid is highly dependent on the size of the effusion and is unlikely to detect effusions <300 mL. A systematic review found the most accurate physical exam findings to rule in a pleural effusion were dullness to percussion (positive likelihood ratio [LR]: 8.7; 95% CI: 2.2‐33.8) and asymmetric chest expansion (positive LR: 8.1; 95% CI: 5.2‐12.7). Normal tactile vocal fremitus was the most accurate physical exam finding to rule out a pleural effusion (negative LR: 0.21; 95% CI: 0.12‐0.37).[6] A major limitation of all these studies is that physical exam was compared to chest radiography as the reference standard, and posterior‐anterior chest radiographs are not sensitive for detection of pleural effusions until 200 mL of fluid has accumulated.[7] Further, chest percussion penetrates to a maximum depth of 6 cm, and its utility is limited in obese patients.[8] Characteristics of pleural fluid that can change management, such as loculations, cannot be detected by physical exam.
Chest Radiography
Chest radiography has traditionally been used to diagnose pleural effusions. Free‐flowing pleural fluid collects in the most dependent portions of the thorax, initially in the subpulmonic space followed by the costophrenic recesses. Pleural fluid is detectable in the costophrenic recesses on lateral upright chest radiograph after 50 mL has accumulated. On standard posterior‐anterior chest radiograph, blunting of the costophrenic recesses and obliteration of the hemidiaphragm are seen when >200 mL and >500 mL of pleural fluid have accumulated, respectively.[7] However, upright chest radiographs can miss a considerable number of effusions, including as many as 10% of parapneumonic effusions large enough to indicate need for drainage.[9] Supine anterior‐posterior chest radiographs can miss a significant proportion of large effusions seen on chest CT,[10] ultrasound,[11] and lateral decubitus radiographs.[12] Pleural effusions are frequently mistaken for parenchymal opacities on portable anterior‐posterior chest radiographs.[10]
Computerized Tomography
Chest CT serves as the reference standard in most modern diagnostic accuracy studies. Limitations of chest CT include difficulty distinguishing small effusions from pleural thickening, dependent atelectasis, or tumor; lower sensitivity for detecting pleural fluid septations compared to ultrasound[13]; exposure of patients to approximately 7 mSv of ionizing radiation (the equivalent radiation dose of 350 chest radiographs)[14]; high cost; and need to transport patients to radiology departments where CT scanners are located.
Pleural Ultrasonography
Ultrasound can rapidly differentiate conditions that demonstrate a nonspecific, radiopaque appearance of lower lung fields on chest radiographs, including pleural effusions, pneumonia, atelectasis, elevated hemidiaphragm, and lung or pleural masses. Advantages of point‐of‐care ultrasound include the ability of hospitalists to acquire and interpret images at the bedside and integrate findings into clinical decision making immediately. Multiple studies have demonstrated superior diagnostic accuracy of ultrasound compared to chest radiography for detection of pleural effusions. Pleural ultrasound can detect physiologic amounts of pleural fluid (5 mL),[15] but a minimal volume of 20 mL is more reliably detected,[16] and ultrasound is 100% sensitive for effusions >100 mL.[17] In a prospective study of critically ill patients with acute respiratory distress syndrome, the diagnostic accuracy of ultrasound for pleural effusions was superior (93%) compared to auscultation (61%) and anterior‐posterior chest radiograph (47%), using chest CT as the reference standard.[18] A meta‐analysis of 4 studies calculated a pooled sensitivity and specificity of ultrasound for detection of pleural effusions as 93% (95% CI: 89%‐96%) and 96% (95% CI: 95%‐98%), respectively.[18, 19, 20, 21, 22] Ultrasound has the additional benefit of characterizing underlying lung parenchyma, which is well described in the literature but beyond the scope of this review.[23]
Sensitivity and specificity of chest radiography and ultrasonography to detect a pleural effusion are displayed in Table 1.[9, 10, 11, 12, 18, 20, 21, 22, 24, 25, 26]
Exam | Reference Standard | Sensitivity | Specificity | Study | |
---|---|---|---|---|---|
| |||||
Chest radiograph | Supine AP | Upright PA/lateral | 92% | Woodring[24] | |
Lateral decubitus XR | 67% | 70% | Ruskin[12] | ||
Ultrasound | 82% | 82% | Emamian[11] | ||
Ultrasound or thoracentesis | 33% | Kocijancic[25] | |||
CT | 39% | 85% | Lichtenstein[18] | ||
CT | 66% | 89% | Kitazano[10] | ||
CT | 65% | 81% | Xirouchaki[26] | ||
CT | 78% | 76% | Brixey[9] | ||
Lateral decubitus | Ultrasound or thoracentesis | 94% | 100% | Kocijancic[25] | |
Upright PA | CT | 82% | 81% | Brixey[9] | |
Lateral upright | CT | 86% | 88% | Brixey[9] | |
Ultrasound | Cardiology | CT | 93% | 88% | Kataoka[20] |
Point of care | CT or tube thoracostomy | 96% | 100% | Ma[21] | |
CT | 92% | 93% | Lichtenstein[18] | ||
CT | 94% | 99% | Rocco[22] | ||
CT | 100% | 100% | Xirouchaki[26] |
PLEURAL ULTRASOUND EXAMINATION
A pleural ultrasound exam may be performed as part of a complete lung ultrasound exam, such as the BLUE (Bedside Lung Ultrasound in Emergency) protocol,[27] or a focused exam to evaluate a suspected or known pleural effusion seen on chest radiograph or CT scan.[27] Free‐flowing pleural effusions accumulate in the most dependent portions of the thorax, most commonly, the posterolateral costophrenic recesses in semirecumbent or seated patients, but anteriorly in mechanically ventilated patients in a prone position.
A low‐frequency (25 MHz) phased‐array transducer is generally preferred for imaging in between the ribs. High‐frequency linear transducers do not provide adequate penetration to visualize deep structures, but do provide superior visualization of the pleural line to assess pleural thickness, measure pleural depth, and evaluate for pneumothorax.
Pleural effusions are best evaluated starting at the level of the diaphragm. Place the transducer in a longitudinal plane on the posterior axillary line at the level of the diaphragm with the transducer orientation marker (notch) pointed cephalad (Figure 1). Five structures must be definitively identified to diagnose a pleural effusion: liver/spleen, diaphragm, pleural fluid, lung, and chest wall (Figure 2A). Large pleural effusions compress the adjacent lung causing atelectasis, which gives the lung a tissue‐like echogenicity similar to the liver (Figure 2B). Static air bronchograms are commonly seen in atelectatic lung bases with pleural effusions.[28]
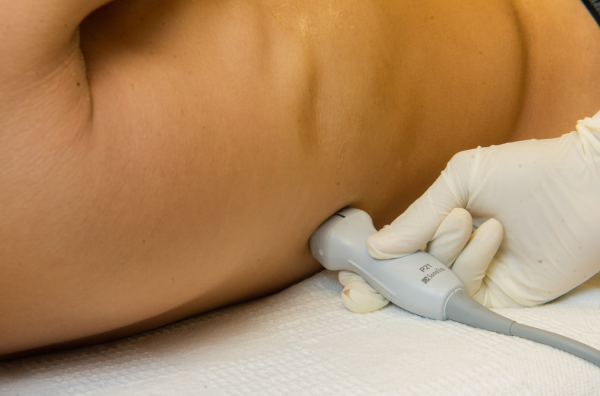
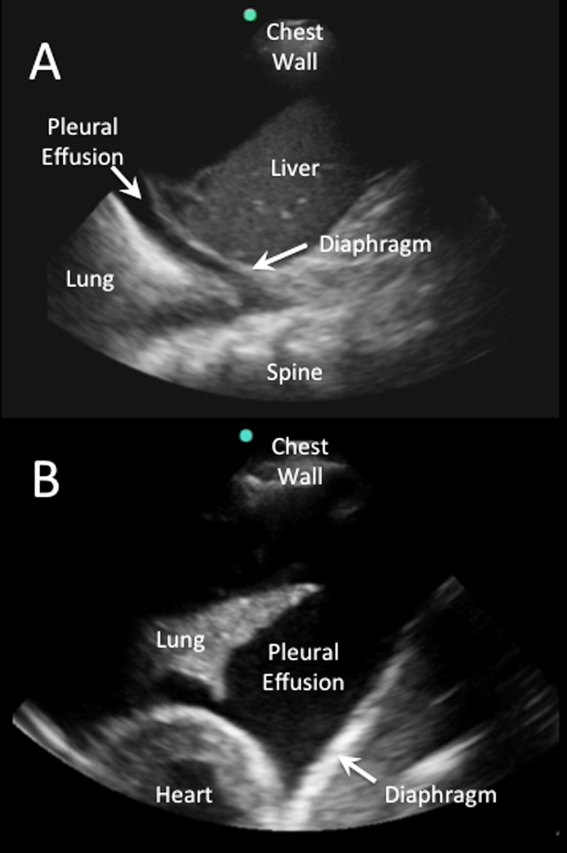
Color flow Doppler and M‐mode ultrasound may be utilized as adjuncts to routine 2‐dimensional ultrasonography. Free‐flowing pleural effusions will demonstrate flow by color Doppler (Figure 3A). Using M‐mode ultrasound, the lung can been seen moving within a pleural effusion to and from the chest wall (sinusoid sign).[29] Absence of flow or movement is seen with dense pleural loculations, pleural thickening, and peripheral lung or pleural masses (Figure 3B).
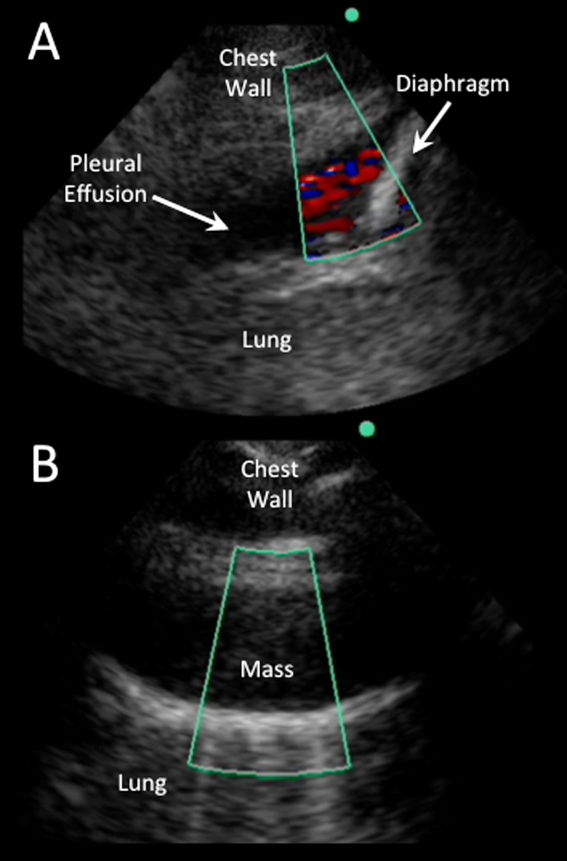
CHARACTERIZATION OF PLEURAL EFFUSION
Pleural Fluid Volume
Quantification of pleural fluid volume has been proposed using formulas with sonographic measurements.[30, 31, 32] These formulas are most accurate for moderate‐sized effusions but have not been validated beyond individual study cohorts. The largest study (n = 150) found a strong correlation between calculated and actual volumes drained by thoracentesis (r2 = 0.79; P < 0.001) using the formula (Volume [mL] = 16 parietal to visceral pleura distance (mm) at the mid‐diaphragm).[31] Although an accurate quantitative pleural fluid volume assessment may be possible, these formulas are not commonly used in clinical practice. A qualitative assessment is adequate for most clinical decision making using categories of minimal, small, moderate, or large volume.
Simple Versus Complex Effusions
Based on its sonographic appearance, pleural effusions are categorized as simple or complex. Simple pleural effusions are anechoic and usually transudative. Complex pleural effusions are subcategorized as homogeneously or heterogeneously echogenic, with or without septations, and are more often exudative.[33]
Effusions with heterogeneous echogenicity with swirling echoes suggest high cellular content that is often associated with malignancy.[34] Fibrinous stranding, septations, and loculations also suggest an exudative effusion (Figure 4A), and are more readily identified and characterized on lung ultrasound than CT scan.[35]
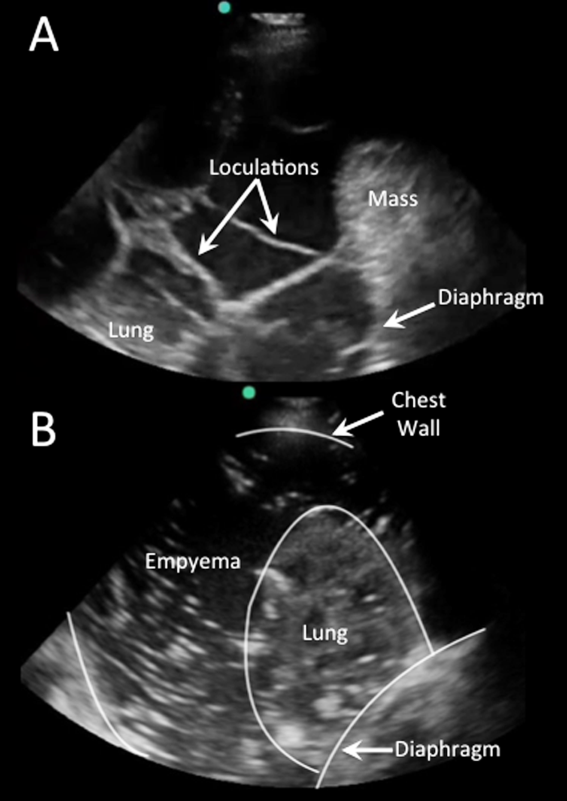
Homogenously echogenic effusions are most often due to hemothorax or empyema.[36] The high cell count of a hemothorax creates a layering effect in costophrenic recesses (hematocrit sign). Empyemas develop from complex effusions that organize into collections of pus and usually have a homogeneously echogenic, speckled appearance (Figure 4B). Sonographic evidence of septations in the presence of empyema predicts the need for intrapleural fibrinolytic therapy, longer duration of drainage, and possible surgical intervention.[37]
Isolated dense loculations may be challenging to differentiate from peripheral lung or pleural lesions, such as abscess or tumor.
Pleural Thickness
Normal visceral and parietal pleura are apposed and 0.2 to 0.3 mm thick.[38] Pleural effusions with parietal pleural thickness >10 mm, pleural nodularity, and diaphragmatic thickness >7 mm predicted underlying malignancy with high specificity and positive predictive value in 1 study.[39] As many as 20% of anechoic lesions of the pleura are solid rather than fluid. Color flow Doppler ultrasound can differentiate small pleural effusions from solid pleural abnormalities with sensitivity and specificity of 89% and 100%, respectively.[40]
PLEURAL FLUID DRAINAGE
Since its first description in 1967, use of ultrasound guidance for thoracentesis has evolved to become the standard of care in many hospitals in the United States.[41] The British Thoracic Society guidelines recommend that all thoracenteses be performed with ultrasound guidance.[42] The American College of Graduate Medical Education now requires proficiency in the use of ultrasound for thoracentesis and pleural catheter insertion by pulmonary and critical care fellows.[43]
The impetus for these recommendations stems from increased procedural success and decreased complications associated with ultrasound‐guided drainage of pleural effusions. A study evaluating thoracentesis site selection based on physical exam and chest radiographs demonstrated inaccurate site selection in 15% of patients, and use of ultrasound for site selection prevented possible accidental organ puncture in 10% of all cases.[44] The success rate of thoracentesis for small pleural effusions has been shown to increase from 66% to 90% with ultrasound guidance.[42] Using ultrasound, the distance from the skin to parietal and visceral pleura can be measured to determine whether thoracentesis can be safely performed, and to guide selection of an adequate length needle (Figure 5). A minimum pleural effusion depth of 1.5 cm between the visceral and parietal pleura has been recommended to perform diagnostic thoracentesis.[28] Diagnostic thoracentesis of complex septated pleural effusions or empyemas may be performed with a straight needle, but therapeutic drainage often requires temporary insertion of a catheter. Traditionally, large‐bore chest tubes (>24 F) had been advocated to drain viscid pus, but recent evidence suggests that small‐bore catheters (1014 F) with instillation of thrombolytics may be as effective and performed with less discomfort.[45] Video‐assisted thoracoscopy to lyse septations and evacuate infected materials is indicated when chest tube drainage has failed.
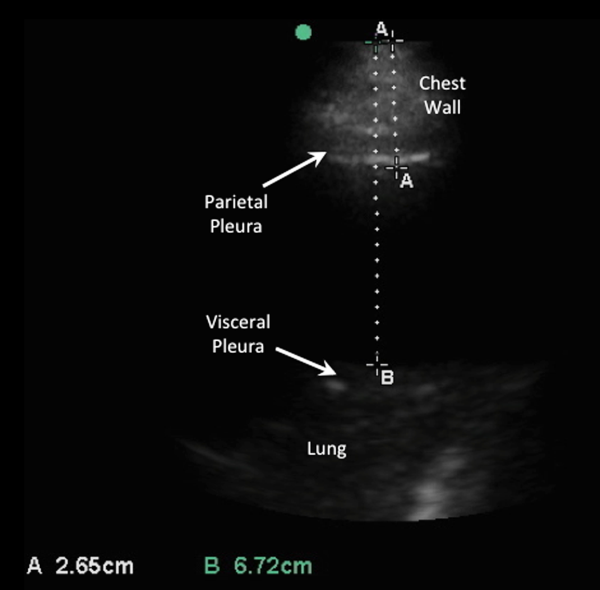
The most common complication of pleural drainage is pneumothorax. A meta‐analysis demonstrated a reduction in post‐thoracentesis pneumothorax rates from 9% to 4% with use of ultrasound.[46] Transporting patients to radiology for ultrasound marking has not been shown to decrease pneumothorax rates compared to thoracentesis without ultrasound guidance, likely due to changes in patient position and prolonged delays between marking and drainage.[47] Postprocedure pneumothorax can be ruled out if lung sliding is visualized. A meta‐analysis demonstrated superior sensitivity and similar specificity of pleural ultrasonography versus chest radiography to detect pneumothorax (sensitivity 91% vs 50% and specificity 98% vs 99%, respectively).[48] Real‐time ultrasound guidance for thoracentesis, or use of ultrasound to track the needle tip, has not been well studied but may be performed by experienced proceduralists to drain small effusions.
FUTURE RESEARCH
Future research should focus on the clinical effectiveness of point‐of‐care pleural ultrasonography when integrated with other diagnostic tools, and application of new ultrasound technologies to evaluate pleural diseases. Routine use of point‐of‐care ultrasound as the primary imaging modality in a medical ICU demonstrated a highly statistically significant reduction in chest x‐rays (3.75 vs 0.82, P < 0.0001) and chest CT scans (0.10 vs 0.04, P = 0.0007).[49] Similar studies have yet to be performed with the use of ultrasound specifically in the management of pleural diseases. Thus, clinical effectiveness studies are needed to assess the impact of routine use of pleural ultrasound on the initiation of appropriate therapies, length of stay, and costs in the management of pleural disease.
Point‐of‐care pleural ultrasound findings need to be evaluated in the context of other clinical findings and diagnostic tests. Certain ultrasound findings have been associated with exudative pleural effusions, but whether exudative and transudative effusions can be differentiated noninvasively using ultrasound findings alone, or in combination with other clinical data, warrants investigation. Similar to severity of illness scores, models that incorporate clinical, laboratory, and ultrasound findings need to be developed to guide treatment decisions, such as type of drainage procedure, as well as prognostication.
Finally, new technologies may advance the utility of point‐of‐care pleural ultrasonography. Even though 3‐dimensional ultrasonography has been available for over 2 decades, comparative studies of conventional 2‐dimensional versus 3‐dimensional ultrasonography to characterize pleural effusions have yet to be performed. Furthermore, computer‐aided detection has been shown to facilitate interpretation of ultrasound images, but this technology has yet to be applied to pleural ultrasonography.
CONCLUSIONS
Point‐of‐care pleural ultrasound is a powerful bedside tool in the hospitalist's armamentarium that is superior to physical examination and chest radiographs in the detection and characterization of pleural effusions. Furthermore, ultrasound performs similarly when compared to CT scans but offers the advantages of decreased cost, avoidance of ionizing radiation, and availability at the bedside. Ultrasound guidance reduces complications and increases the success rate of pleural drainage procedures, leading to improved patient safety. As clinical effectiveness studies emerge revealing its true value, point‐of‐care pleural ultrasonography is likely to become the standard diagnostic tool to evaluate and manage patients with pleural effusions.
Disclosures: Dr. Restrepo is partially supported by award number K23HL096054 from the National Heart, Lung, and Blood Institute. The content is solely the responsibility of the authors and does not necessarily represent the official views of the National Heart, Lung, and Blood Institute or the National Institutes of Health. The authors report no conflicts of interest.
Hospitalists commonly encounter pleural effusions, and their detection and characterization by point‐of‐care ultrasound can guide management. Approximately 44% to 57% of hospitalized patients with bacterial pneumonia,[1, 2] and up to 62% of intensive care unit (ICU) patients[3] have a pleural effusion. For patients with a parapneumonic effusion, hospitalists can use ultrasound to quantify and characterize pleural fluid to determine whether diagnostic or therapeutic drainage is indicated, as well as guide performance of thoracentesis. For patients with lung cancer, detection of a malignant pleural effusion changes staging to stage IV, regardless of tumor size or lymph node involvement, and hospitalists may discuss more appropriate treatment options with patients and consultants.
Routine use of pleural ultrasonography may help hospitalists provide high‐value care by reducing ancillary testing, including computerized tomography (CT) scans that expose patients to ionizing radiation, and reducing complications of thoracentesis. However, many hospitalists may not be familiar with the use of point‐of‐care ultrasound. A national survey in 2012 revealed only 25% of internal medicine residencies have formal curricula to teach point‐of‐care ultrasonography.[4] The purpose of this review is to provide an overview of how point‐of‐care ultrasound can be utilized by hospitalists in the care of patients with pleural effusions. We review the literature on the diagnosis and evaluation of pleural effusions with ultrasound, as well as techniques to examine and drain the pleural space.
DIAGNOSIS OF PLEURAL EFFUSION
History and Physical Exam
Pleural effusions are most commonly associated with heart failure, pneumonia, cancer, pulmonary embolism, viral disease, coronary artery bypass surgery, and cirrhosis with ascites. The most common symptoms related to pleural effusion are nonspecific and often indistinguishable from those of the underlying disease process, including cough, dyspnea, and pleuritic chest pain.[5]
Diagnostic accuracy of a physical examination to detect pleural fluid is highly dependent on the size of the effusion and is unlikely to detect effusions <300 mL. A systematic review found the most accurate physical exam findings to rule in a pleural effusion were dullness to percussion (positive likelihood ratio [LR]: 8.7; 95% CI: 2.2‐33.8) and asymmetric chest expansion (positive LR: 8.1; 95% CI: 5.2‐12.7). Normal tactile vocal fremitus was the most accurate physical exam finding to rule out a pleural effusion (negative LR: 0.21; 95% CI: 0.12‐0.37).[6] A major limitation of all these studies is that physical exam was compared to chest radiography as the reference standard, and posterior‐anterior chest radiographs are not sensitive for detection of pleural effusions until 200 mL of fluid has accumulated.[7] Further, chest percussion penetrates to a maximum depth of 6 cm, and its utility is limited in obese patients.[8] Characteristics of pleural fluid that can change management, such as loculations, cannot be detected by physical exam.
Chest Radiography
Chest radiography has traditionally been used to diagnose pleural effusions. Free‐flowing pleural fluid collects in the most dependent portions of the thorax, initially in the subpulmonic space followed by the costophrenic recesses. Pleural fluid is detectable in the costophrenic recesses on lateral upright chest radiograph after 50 mL has accumulated. On standard posterior‐anterior chest radiograph, blunting of the costophrenic recesses and obliteration of the hemidiaphragm are seen when >200 mL and >500 mL of pleural fluid have accumulated, respectively.[7] However, upright chest radiographs can miss a considerable number of effusions, including as many as 10% of parapneumonic effusions large enough to indicate need for drainage.[9] Supine anterior‐posterior chest radiographs can miss a significant proportion of large effusions seen on chest CT,[10] ultrasound,[11] and lateral decubitus radiographs.[12] Pleural effusions are frequently mistaken for parenchymal opacities on portable anterior‐posterior chest radiographs.[10]
Computerized Tomography
Chest CT serves as the reference standard in most modern diagnostic accuracy studies. Limitations of chest CT include difficulty distinguishing small effusions from pleural thickening, dependent atelectasis, or tumor; lower sensitivity for detecting pleural fluid septations compared to ultrasound[13]; exposure of patients to approximately 7 mSv of ionizing radiation (the equivalent radiation dose of 350 chest radiographs)[14]; high cost; and need to transport patients to radiology departments where CT scanners are located.
Pleural Ultrasonography
Ultrasound can rapidly differentiate conditions that demonstrate a nonspecific, radiopaque appearance of lower lung fields on chest radiographs, including pleural effusions, pneumonia, atelectasis, elevated hemidiaphragm, and lung or pleural masses. Advantages of point‐of‐care ultrasound include the ability of hospitalists to acquire and interpret images at the bedside and integrate findings into clinical decision making immediately. Multiple studies have demonstrated superior diagnostic accuracy of ultrasound compared to chest radiography for detection of pleural effusions. Pleural ultrasound can detect physiologic amounts of pleural fluid (5 mL),[15] but a minimal volume of 20 mL is more reliably detected,[16] and ultrasound is 100% sensitive for effusions >100 mL.[17] In a prospective study of critically ill patients with acute respiratory distress syndrome, the diagnostic accuracy of ultrasound for pleural effusions was superior (93%) compared to auscultation (61%) and anterior‐posterior chest radiograph (47%), using chest CT as the reference standard.[18] A meta‐analysis of 4 studies calculated a pooled sensitivity and specificity of ultrasound for detection of pleural effusions as 93% (95% CI: 89%‐96%) and 96% (95% CI: 95%‐98%), respectively.[18, 19, 20, 21, 22] Ultrasound has the additional benefit of characterizing underlying lung parenchyma, which is well described in the literature but beyond the scope of this review.[23]
Sensitivity and specificity of chest radiography and ultrasonography to detect a pleural effusion are displayed in Table 1.[9, 10, 11, 12, 18, 20, 21, 22, 24, 25, 26]
Exam | Reference Standard | Sensitivity | Specificity | Study | |
---|---|---|---|---|---|
| |||||
Chest radiograph | Supine AP | Upright PA/lateral | 92% | Woodring[24] | |
Lateral decubitus XR | 67% | 70% | Ruskin[12] | ||
Ultrasound | 82% | 82% | Emamian[11] | ||
Ultrasound or thoracentesis | 33% | Kocijancic[25] | |||
CT | 39% | 85% | Lichtenstein[18] | ||
CT | 66% | 89% | Kitazano[10] | ||
CT | 65% | 81% | Xirouchaki[26] | ||
CT | 78% | 76% | Brixey[9] | ||
Lateral decubitus | Ultrasound or thoracentesis | 94% | 100% | Kocijancic[25] | |
Upright PA | CT | 82% | 81% | Brixey[9] | |
Lateral upright | CT | 86% | 88% | Brixey[9] | |
Ultrasound | Cardiology | CT | 93% | 88% | Kataoka[20] |
Point of care | CT or tube thoracostomy | 96% | 100% | Ma[21] | |
CT | 92% | 93% | Lichtenstein[18] | ||
CT | 94% | 99% | Rocco[22] | ||
CT | 100% | 100% | Xirouchaki[26] |
PLEURAL ULTRASOUND EXAMINATION
A pleural ultrasound exam may be performed as part of a complete lung ultrasound exam, such as the BLUE (Bedside Lung Ultrasound in Emergency) protocol,[27] or a focused exam to evaluate a suspected or known pleural effusion seen on chest radiograph or CT scan.[27] Free‐flowing pleural effusions accumulate in the most dependent portions of the thorax, most commonly, the posterolateral costophrenic recesses in semirecumbent or seated patients, but anteriorly in mechanically ventilated patients in a prone position.
A low‐frequency (25 MHz) phased‐array transducer is generally preferred for imaging in between the ribs. High‐frequency linear transducers do not provide adequate penetration to visualize deep structures, but do provide superior visualization of the pleural line to assess pleural thickness, measure pleural depth, and evaluate for pneumothorax.
Pleural effusions are best evaluated starting at the level of the diaphragm. Place the transducer in a longitudinal plane on the posterior axillary line at the level of the diaphragm with the transducer orientation marker (notch) pointed cephalad (Figure 1). Five structures must be definitively identified to diagnose a pleural effusion: liver/spleen, diaphragm, pleural fluid, lung, and chest wall (Figure 2A). Large pleural effusions compress the adjacent lung causing atelectasis, which gives the lung a tissue‐like echogenicity similar to the liver (Figure 2B). Static air bronchograms are commonly seen in atelectatic lung bases with pleural effusions.[28]
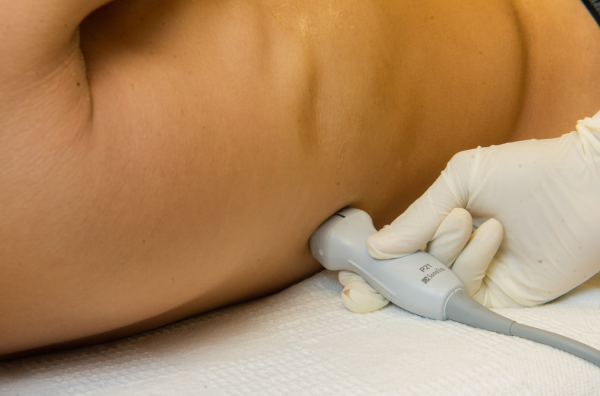
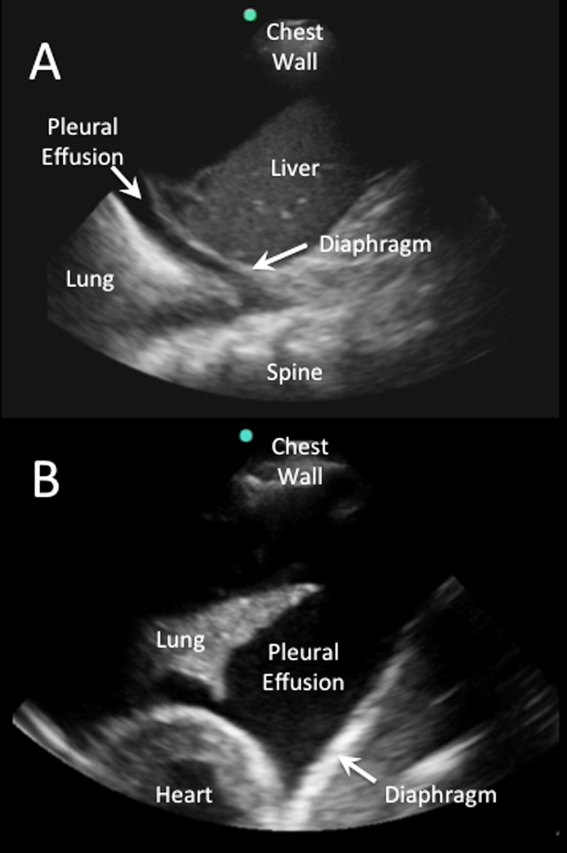
Color flow Doppler and M‐mode ultrasound may be utilized as adjuncts to routine 2‐dimensional ultrasonography. Free‐flowing pleural effusions will demonstrate flow by color Doppler (Figure 3A). Using M‐mode ultrasound, the lung can been seen moving within a pleural effusion to and from the chest wall (sinusoid sign).[29] Absence of flow or movement is seen with dense pleural loculations, pleural thickening, and peripheral lung or pleural masses (Figure 3B).
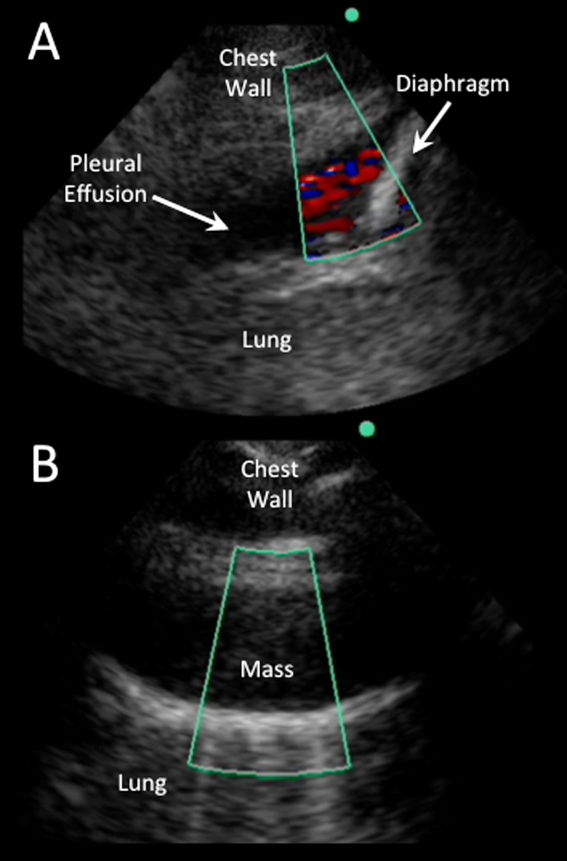
CHARACTERIZATION OF PLEURAL EFFUSION
Pleural Fluid Volume
Quantification of pleural fluid volume has been proposed using formulas with sonographic measurements.[30, 31, 32] These formulas are most accurate for moderate‐sized effusions but have not been validated beyond individual study cohorts. The largest study (n = 150) found a strong correlation between calculated and actual volumes drained by thoracentesis (r2 = 0.79; P < 0.001) using the formula (Volume [mL] = 16 parietal to visceral pleura distance (mm) at the mid‐diaphragm).[31] Although an accurate quantitative pleural fluid volume assessment may be possible, these formulas are not commonly used in clinical practice. A qualitative assessment is adequate for most clinical decision making using categories of minimal, small, moderate, or large volume.
Simple Versus Complex Effusions
Based on its sonographic appearance, pleural effusions are categorized as simple or complex. Simple pleural effusions are anechoic and usually transudative. Complex pleural effusions are subcategorized as homogeneously or heterogeneously echogenic, with or without septations, and are more often exudative.[33]
Effusions with heterogeneous echogenicity with swirling echoes suggest high cellular content that is often associated with malignancy.[34] Fibrinous stranding, septations, and loculations also suggest an exudative effusion (Figure 4A), and are more readily identified and characterized on lung ultrasound than CT scan.[35]
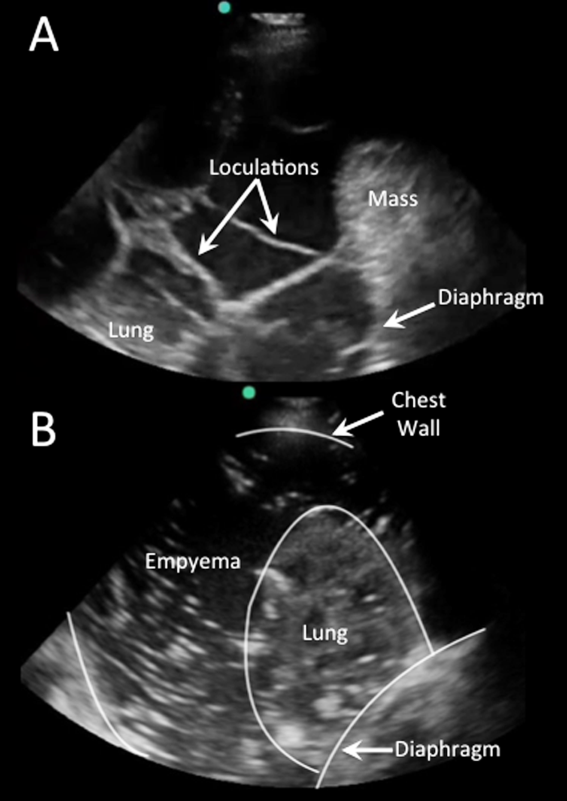
Homogenously echogenic effusions are most often due to hemothorax or empyema.[36] The high cell count of a hemothorax creates a layering effect in costophrenic recesses (hematocrit sign). Empyemas develop from complex effusions that organize into collections of pus and usually have a homogeneously echogenic, speckled appearance (Figure 4B). Sonographic evidence of septations in the presence of empyema predicts the need for intrapleural fibrinolytic therapy, longer duration of drainage, and possible surgical intervention.[37]
Isolated dense loculations may be challenging to differentiate from peripheral lung or pleural lesions, such as abscess or tumor.
Pleural Thickness
Normal visceral and parietal pleura are apposed and 0.2 to 0.3 mm thick.[38] Pleural effusions with parietal pleural thickness >10 mm, pleural nodularity, and diaphragmatic thickness >7 mm predicted underlying malignancy with high specificity and positive predictive value in 1 study.[39] As many as 20% of anechoic lesions of the pleura are solid rather than fluid. Color flow Doppler ultrasound can differentiate small pleural effusions from solid pleural abnormalities with sensitivity and specificity of 89% and 100%, respectively.[40]
PLEURAL FLUID DRAINAGE
Since its first description in 1967, use of ultrasound guidance for thoracentesis has evolved to become the standard of care in many hospitals in the United States.[41] The British Thoracic Society guidelines recommend that all thoracenteses be performed with ultrasound guidance.[42] The American College of Graduate Medical Education now requires proficiency in the use of ultrasound for thoracentesis and pleural catheter insertion by pulmonary and critical care fellows.[43]
The impetus for these recommendations stems from increased procedural success and decreased complications associated with ultrasound‐guided drainage of pleural effusions. A study evaluating thoracentesis site selection based on physical exam and chest radiographs demonstrated inaccurate site selection in 15% of patients, and use of ultrasound for site selection prevented possible accidental organ puncture in 10% of all cases.[44] The success rate of thoracentesis for small pleural effusions has been shown to increase from 66% to 90% with ultrasound guidance.[42] Using ultrasound, the distance from the skin to parietal and visceral pleura can be measured to determine whether thoracentesis can be safely performed, and to guide selection of an adequate length needle (Figure 5). A minimum pleural effusion depth of 1.5 cm between the visceral and parietal pleura has been recommended to perform diagnostic thoracentesis.[28] Diagnostic thoracentesis of complex septated pleural effusions or empyemas may be performed with a straight needle, but therapeutic drainage often requires temporary insertion of a catheter. Traditionally, large‐bore chest tubes (>24 F) had been advocated to drain viscid pus, but recent evidence suggests that small‐bore catheters (1014 F) with instillation of thrombolytics may be as effective and performed with less discomfort.[45] Video‐assisted thoracoscopy to lyse septations and evacuate infected materials is indicated when chest tube drainage has failed.
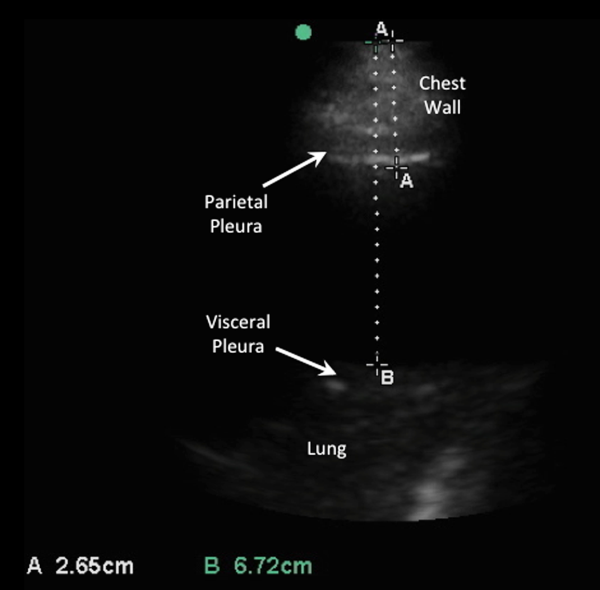
The most common complication of pleural drainage is pneumothorax. A meta‐analysis demonstrated a reduction in post‐thoracentesis pneumothorax rates from 9% to 4% with use of ultrasound.[46] Transporting patients to radiology for ultrasound marking has not been shown to decrease pneumothorax rates compared to thoracentesis without ultrasound guidance, likely due to changes in patient position and prolonged delays between marking and drainage.[47] Postprocedure pneumothorax can be ruled out if lung sliding is visualized. A meta‐analysis demonstrated superior sensitivity and similar specificity of pleural ultrasonography versus chest radiography to detect pneumothorax (sensitivity 91% vs 50% and specificity 98% vs 99%, respectively).[48] Real‐time ultrasound guidance for thoracentesis, or use of ultrasound to track the needle tip, has not been well studied but may be performed by experienced proceduralists to drain small effusions.
FUTURE RESEARCH
Future research should focus on the clinical effectiveness of point‐of‐care pleural ultrasonography when integrated with other diagnostic tools, and application of new ultrasound technologies to evaluate pleural diseases. Routine use of point‐of‐care ultrasound as the primary imaging modality in a medical ICU demonstrated a highly statistically significant reduction in chest x‐rays (3.75 vs 0.82, P < 0.0001) and chest CT scans (0.10 vs 0.04, P = 0.0007).[49] Similar studies have yet to be performed with the use of ultrasound specifically in the management of pleural diseases. Thus, clinical effectiveness studies are needed to assess the impact of routine use of pleural ultrasound on the initiation of appropriate therapies, length of stay, and costs in the management of pleural disease.
Point‐of‐care pleural ultrasound findings need to be evaluated in the context of other clinical findings and diagnostic tests. Certain ultrasound findings have been associated with exudative pleural effusions, but whether exudative and transudative effusions can be differentiated noninvasively using ultrasound findings alone, or in combination with other clinical data, warrants investigation. Similar to severity of illness scores, models that incorporate clinical, laboratory, and ultrasound findings need to be developed to guide treatment decisions, such as type of drainage procedure, as well as prognostication.
Finally, new technologies may advance the utility of point‐of‐care pleural ultrasonography. Even though 3‐dimensional ultrasonography has been available for over 2 decades, comparative studies of conventional 2‐dimensional versus 3‐dimensional ultrasonography to characterize pleural effusions have yet to be performed. Furthermore, computer‐aided detection has been shown to facilitate interpretation of ultrasound images, but this technology has yet to be applied to pleural ultrasonography.
CONCLUSIONS
Point‐of‐care pleural ultrasound is a powerful bedside tool in the hospitalist's armamentarium that is superior to physical examination and chest radiographs in the detection and characterization of pleural effusions. Furthermore, ultrasound performs similarly when compared to CT scans but offers the advantages of decreased cost, avoidance of ionizing radiation, and availability at the bedside. Ultrasound guidance reduces complications and increases the success rate of pleural drainage procedures, leading to improved patient safety. As clinical effectiveness studies emerge revealing its true value, point‐of‐care pleural ultrasonography is likely to become the standard diagnostic tool to evaluate and manage patients with pleural effusions.
Disclosures: Dr. Restrepo is partially supported by award number K23HL096054 from the National Heart, Lung, and Blood Institute. The content is solely the responsibility of the authors and does not necessarily represent the official views of the National Heart, Lung, and Blood Institute or the National Institutes of Health. The authors report no conflicts of interest.
- Parapneumonic effusions. Am J Med. 1980;69(4):507–512. , , , .
- The incidence and clinical correlates of parapneumonic effusions in pneumococcal pneumonia. Chest. 1978;74(2):170–173. , , .
- Pleural effusions in the medical ICU: prevalence, causes, and clinical implications. Chest. 1997;111(4):1018–1023. , , , , .
- Point‐of‐care ultrasound in internal medicine: a national survey of educational leadership. J Grad Med Educ. 2013;5(3):498–502. , , , .
- Pleural Diseases. Philadelphia, PA: Lippincott Williams 2007. .
- Does this patient have a pleural effusion? JAMA. 2009;301(3):309–317. , , .
- Pleural fluid volume estimation: a chest radiograph prediction rule. Acad Radiol. 1996;3(2):103–109. , , , .
- Accuracy of the physical examination in evaluating pleural effusion. Cleve Clin J Med. 2008;75(4):297–303. , .
- The efficacy of chest radiographs in detecting parapneumonic effusions. Respirology. 2011;16(6):1000–1004. , , , , .
- Differentiation of pleural effusions from parenchymal opacities: accuracy of bedside chest radiography. AJR Am J Roentgenol. 2010;194(2):407–412. , , , , .
- Accuracy of the diagnosis of pleural effusion on supine chest X‐ray. Eur Radiol. 1997;7(1):57–60. , , , .
- Detection of pleural effusions on supine chest radiographs. AJR Am J Roentgenol. 1987;148(4):681–683. , , , .
- Multiloculated pleural effusion detected by ultrasound only in a critically‐ill patient. Am J Case Rep. 2013;14:63–66. , , , , .
- Exposure to low‐dose ionizing radiation from medical imaging procedures. N Engl J Med. 2009;361(9):849–857. , , , et al.
- The diagnosis of pleural effusion by ultrasonic and radiologic techniques. Chest. 1976;70(1):33–37. , , .
- Ultrasound in blunt abdominal and thoracic trauma. J Trauma. 1993;34(4):488–495. , , , , , .
- Application of color Doppler ultrasound in the study of small pleural effusion. Med Ultrason. 2010;12(1):12–16. , , , et al.
- Comparative diagnostic performances of auscultation, chest radiography, and lung ultrasonography in acute respiratory distress syndrome. Anesthesiology. 2004;100(1):9–15. , , , , , .
- Diagnostic accuracy of sonography for pleural effusion: systematic review. Sao Paulo Med J. 2010;128(2):90–95. , , , , .
- The role of thoracic ultrasonography for evaluation of patients with decompensated chronic heart failure. J Am Coll Cardiol. 2000;35(6):1638–1646. , .
- Trauma ultrasound examination versus chest radiography in the detection of hemothorax. Ann Emerg Med. 1997;29(3):312–315; discussion 315–316. , .
- Diagnostic accuracy of bedside ultrasonography in the ICU: feasibility of detecting pulmonary effusion and lung contusion in patients on respiratory support after severe blunt thoracic trauma. Acta Anaesthesiol Scand. 2008;52(6):776–784. , , , et al.
- Lung ultrasound in the critically ill. Ann Intensive Care. 2014;4(1):1. .
- Recognition of pleural effusion on supine radiographs: how much fluid is required? AJR. Am J Roentgenol. 1984;142(1):59–64. .
- Chest sonography versus lateral decubitus radiography in the diagnosis of small pleural effusions. J Clin Ultrasound. 2003;31(2):69–74. , , .
- Impact of lung ultrasound on clinical decision making in critically ill patients. Intensive Care Med. 2014;40(1):57–65. , , , , .
- Lung ultrasound in acute respiratory failure an introduction to the BLUE‐protocol. Minerva Anestesiol. 2009;75(5):313–317. .
- Point‐of‐Care Ultrasound. 1st ed. Philadelphia, PA: Saunders; 2014. , , .
- International evidence‐based recommendations for point‐of‐care lung ultrasound. Intensive Care Med. 2012;38(4):577–591. , , , et al.
- Ultrasound estimation of volume of pleural fluid in mechanically ventilated patients. Intensive Care Med. 2006;32(2):318–321. , , , et al.
- Ultrasound estimation of volume of postoperative pleural effusion in cardiac surgery patients. Interact Cardiovasc Thorac Surg. 2010;10(2):204–207. , , .
- Multiplane ultrasound approach to quantify pleural effusion at the bedside. Intensive Care Med. 2010;36(4):656–664. , , , et al.
- Value of sonography in determining the nature of pleural effusion: analysis of 320 cases. AJR Am J Roentgenol. 1992;159(1):29–33. , , , , , .
- Echogenic swirling pattern as a predictor of malignant pleural effusions in patients with malignancies. Chest. 2004;126(1):129–134. , , , , , .
- Imaging the pleura: sonography, CT, and MR imaging. AJR Am J Roentgenol. 1991;156(6):1145–1153. , .
- Pleural effusions in febrile medical ICU patients: chest ultrasound study. Chest. 2004;126(4):1274–1280. , , , et al.
- Sonographic septation: a useful prognostic indicator of acute thoracic empyema. J Ultrasound Med. 2000;19(12):837–843. , , , , .
- Sonography of the pleura [in German]. Ultraschall Med. 2010;31(1):8–22, quiz 23–25. .
- Thoracic ultrasound in the diagnosis of malignant pleural effusion. Thorax. 2009;64(2):139–143. , , .
- “Fluid color” sign: a useful indicator for discrimination between pleural thickening and pleural effusion. J Ultrasound Med. 1995;14(10):767–769. , , , .
- Reflected ultrasound in the detection and localization of pleural effusion. JAMA. 1967;200(5):399–402. , , .
- Pleural procedures and thoracic ultrasound: British Thoracic Society Pleural Disease Guideline 2010. Thorax. 2010;65(suppl 2):ii61–ii76. , , , .
- Accreditation Council for Graduate Medical Education. http://www.acgme.org/acgmeweb. Accessed January 15, 2015.
- Accuracy of pleural puncture sites: a prospective comparison of clinical examination with ultrasound. Chest. 2003;123(2):436–441. , , .
- A double blind randomized cross over trial comparing rate of decortication and efficacy of intrapleural instillation of alteplase vs placebo in patients with empyemas and complicated parapneumonic effusions. Respir Med. 2012;106(5):716–723. , , , , , .
- Pneumothorax following thoracentesis: a systematic review and meta‐analysis. Arch Intern Med. 2010;170(4):332–339. , , , .
- Factors affecting the development of pneumothorax associated with thoracentesis. AJR Am J Roentgenol. 1991;156(5):917–920. , , , , , .
- Test characteristics of ultrasonography for the detection of pneumothorax: a systematic review and meta‐analysis. Chest. 2012;141(3):703–708. , , .
- The effect of point‐of‐care ultrasonography on imaging studies in the medical ICU: a comparative study. Chest. 2014;146(6):1574–1577. , , , et al.
- Parapneumonic effusions. Am J Med. 1980;69(4):507–512. , , , .
- The incidence and clinical correlates of parapneumonic effusions in pneumococcal pneumonia. Chest. 1978;74(2):170–173. , , .
- Pleural effusions in the medical ICU: prevalence, causes, and clinical implications. Chest. 1997;111(4):1018–1023. , , , , .
- Point‐of‐care ultrasound in internal medicine: a national survey of educational leadership. J Grad Med Educ. 2013;5(3):498–502. , , , .
- Pleural Diseases. Philadelphia, PA: Lippincott Williams 2007. .
- Does this patient have a pleural effusion? JAMA. 2009;301(3):309–317. , , .
- Pleural fluid volume estimation: a chest radiograph prediction rule. Acad Radiol. 1996;3(2):103–109. , , , .
- Accuracy of the physical examination in evaluating pleural effusion. Cleve Clin J Med. 2008;75(4):297–303. , .
- The efficacy of chest radiographs in detecting parapneumonic effusions. Respirology. 2011;16(6):1000–1004. , , , , .
- Differentiation of pleural effusions from parenchymal opacities: accuracy of bedside chest radiography. AJR Am J Roentgenol. 2010;194(2):407–412. , , , , .
- Accuracy of the diagnosis of pleural effusion on supine chest X‐ray. Eur Radiol. 1997;7(1):57–60. , , , .
- Detection of pleural effusions on supine chest radiographs. AJR Am J Roentgenol. 1987;148(4):681–683. , , , .
- Multiloculated pleural effusion detected by ultrasound only in a critically‐ill patient. Am J Case Rep. 2013;14:63–66. , , , , .
- Exposure to low‐dose ionizing radiation from medical imaging procedures. N Engl J Med. 2009;361(9):849–857. , , , et al.
- The diagnosis of pleural effusion by ultrasonic and radiologic techniques. Chest. 1976;70(1):33–37. , , .
- Ultrasound in blunt abdominal and thoracic trauma. J Trauma. 1993;34(4):488–495. , , , , , .
- Application of color Doppler ultrasound in the study of small pleural effusion. Med Ultrason. 2010;12(1):12–16. , , , et al.
- Comparative diagnostic performances of auscultation, chest radiography, and lung ultrasonography in acute respiratory distress syndrome. Anesthesiology. 2004;100(1):9–15. , , , , , .
- Diagnostic accuracy of sonography for pleural effusion: systematic review. Sao Paulo Med J. 2010;128(2):90–95. , , , , .
- The role of thoracic ultrasonography for evaluation of patients with decompensated chronic heart failure. J Am Coll Cardiol. 2000;35(6):1638–1646. , .
- Trauma ultrasound examination versus chest radiography in the detection of hemothorax. Ann Emerg Med. 1997;29(3):312–315; discussion 315–316. , .
- Diagnostic accuracy of bedside ultrasonography in the ICU: feasibility of detecting pulmonary effusion and lung contusion in patients on respiratory support after severe blunt thoracic trauma. Acta Anaesthesiol Scand. 2008;52(6):776–784. , , , et al.
- Lung ultrasound in the critically ill. Ann Intensive Care. 2014;4(1):1. .
- Recognition of pleural effusion on supine radiographs: how much fluid is required? AJR. Am J Roentgenol. 1984;142(1):59–64. .
- Chest sonography versus lateral decubitus radiography in the diagnosis of small pleural effusions. J Clin Ultrasound. 2003;31(2):69–74. , , .
- Impact of lung ultrasound on clinical decision making in critically ill patients. Intensive Care Med. 2014;40(1):57–65. , , , , .
- Lung ultrasound in acute respiratory failure an introduction to the BLUE‐protocol. Minerva Anestesiol. 2009;75(5):313–317. .
- Point‐of‐Care Ultrasound. 1st ed. Philadelphia, PA: Saunders; 2014. , , .
- International evidence‐based recommendations for point‐of‐care lung ultrasound. Intensive Care Med. 2012;38(4):577–591. , , , et al.
- Ultrasound estimation of volume of pleural fluid in mechanically ventilated patients. Intensive Care Med. 2006;32(2):318–321. , , , et al.
- Ultrasound estimation of volume of postoperative pleural effusion in cardiac surgery patients. Interact Cardiovasc Thorac Surg. 2010;10(2):204–207. , , .
- Multiplane ultrasound approach to quantify pleural effusion at the bedside. Intensive Care Med. 2010;36(4):656–664. , , , et al.
- Value of sonography in determining the nature of pleural effusion: analysis of 320 cases. AJR Am J Roentgenol. 1992;159(1):29–33. , , , , , .
- Echogenic swirling pattern as a predictor of malignant pleural effusions in patients with malignancies. Chest. 2004;126(1):129–134. , , , , , .
- Imaging the pleura: sonography, CT, and MR imaging. AJR Am J Roentgenol. 1991;156(6):1145–1153. , .
- Pleural effusions in febrile medical ICU patients: chest ultrasound study. Chest. 2004;126(4):1274–1280. , , , et al.
- Sonographic septation: a useful prognostic indicator of acute thoracic empyema. J Ultrasound Med. 2000;19(12):837–843. , , , , .
- Sonography of the pleura [in German]. Ultraschall Med. 2010;31(1):8–22, quiz 23–25. .
- Thoracic ultrasound in the diagnosis of malignant pleural effusion. Thorax. 2009;64(2):139–143. , , .
- “Fluid color” sign: a useful indicator for discrimination between pleural thickening and pleural effusion. J Ultrasound Med. 1995;14(10):767–769. , , , .
- Reflected ultrasound in the detection and localization of pleural effusion. JAMA. 1967;200(5):399–402. , , .
- Pleural procedures and thoracic ultrasound: British Thoracic Society Pleural Disease Guideline 2010. Thorax. 2010;65(suppl 2):ii61–ii76. , , , .
- Accreditation Council for Graduate Medical Education. http://www.acgme.org/acgmeweb. Accessed January 15, 2015.
- Accuracy of pleural puncture sites: a prospective comparison of clinical examination with ultrasound. Chest. 2003;123(2):436–441. , , .
- A double blind randomized cross over trial comparing rate of decortication and efficacy of intrapleural instillation of alteplase vs placebo in patients with empyemas and complicated parapneumonic effusions. Respir Med. 2012;106(5):716–723. , , , , , .
- Pneumothorax following thoracentesis: a systematic review and meta‐analysis. Arch Intern Med. 2010;170(4):332–339. , , , .
- Factors affecting the development of pneumothorax associated with thoracentesis. AJR Am J Roentgenol. 1991;156(5):917–920. , , , , , .
- Test characteristics of ultrasonography for the detection of pneumothorax: a systematic review and meta‐analysis. Chest. 2012;141(3):703–708. , , .
- The effect of point‐of‐care ultrasonography on imaging studies in the medical ICU: a comparative study. Chest. 2014;146(6):1574–1577. , , , et al.