User login
Safety and Efficacy of Percutaneous Injection of Lipogems Micro-Fractured Adipose Tissue for Osteoarthritic Knees
ABSTRACT
The aim of this study was to evaluate the safety and efficacy of using autologous, micro-fractured, minimally manipulated adipose tissue in patients with refractory knee osteoarthritis (OA). A total of 17 subjects (26 knees) with a median age of 72 years (range: 54-78 years) and a history of knee OA (Kellgren–Lawrence, grade of 3 or 4) underwent treatment with ultrasound-guided injection of micro-fractured adipose tissue. Micro-fractured fat was obtained using a minimal manipulation technique in a closed system (Lipogems), without the addition of enzymes or any other additives. The study subjects were clinically evaluated using the numerical pain rating scale (NPRS), the 100-point Knee Society Score (KSS) with its functional component (FXN), and the lower extremity activity scale (LEAS) at 6 weeks, 6 months, and 12 months following this procedure.
When compared with baseline, significant improvements were noted in the mean values of NPRS, FXN, and LEAS at 6 weeks, 6 months, and 12 months. The mean KSS significantly improved at 6 weeks and 12 months. In particular, the average KSS score improved from 74 to 82, the FXN score improved from 65 to 76, and the LEAS score improved from 36 to 47. These values were significantly greater than the previously published minimal clinically important difference described for KSS and FXN in patients who underwent total knee arthroplasty for primary OA. No serious adverse events were reported. The injection of autologous, micro-fractured, minimally manipulated adipose tissue appears to be a safe and effective treatment option for patients with refractory, severe (grade 3 or 4) knee OA.
This study demonstrated significant improvements in pain, quality of life, and function for at least 12 months in this study population. This intervention may represent a nonsurgical treatment option to avoid knee joint replacement in this population; however, further investigation is needed.
Continue to: Knee OA is...
Knee OA is a chronic disease that affects all races, genders, and ages, but it is most prevalent in obese and elderly people. Worldwide, arthritis is considered to be the fourth leading cause of disability.1 In developing and developed countries, knee OA may cause a significant decline in the quality of life for individuals >65 years due to joint pain and disability.1 Nonoperative treatment can be successful in patients with mild to moderate arthritis with pain.
Current treatment options for knee OA, including physical therapy and anti-inflammatory drugs, aim to remedy the symptoms, but they do little to treat the underlying causes of knee OA pain. When a patient presents with advanced arthritis of the knee as confirmed by radiographic findings (classified as Kellgren–Lawrence grade of 3 or 4), the standard approach has been a total knee arthroplasty (TKA) after the patient has failed conservative treatment. The annual rate of total knee replacement in the United States has doubled since 2000, especially in those 45 – 65 years.2 The total number of procedures performed each year now exceeds 640,000, at a total annual cost of about $10.2 billion.2 Multiple studies show that TKA has favorable outcomes in pain relief and functional improvement in patients >60 years when evaluated at a follow-up of 10 years after surgery.2
However, some patients are hesitant to proceed with surgery due to fear of surgical pain and procedural complications. The known complications include deep vein thrombosis, pulmonary embolism, nerve injury, and infection. In addition, up to 20% of patients continue to complain of pain following a total knee replacement.3 Finally, in the young population (<50 years), there are concerns related to the potential need of revision knee surgery in the future.3
Alternative treatments for knee OA have recently emerged, including the use of platelet-rich plasma (PRP). A recent meta-analysis that included 10 randomized controlled trials with a total of 1069 patients demonstrated that, compared with hyaluronic acid and saline, intra-articular PRP injection may have more benefits in pain relief and functional improvement in patients with symptomatic knee OA at 1-year post-injection.4 Another smaller study examined patients who had experienced mild knee OA (Kellgren–Lawrence grade <3) for an average of 14 months. Each patient underwent magnetic resonance imaging for the evaluation of joint damage and then received a single PRP injection. The patients were assessed at regular intervals, with improvement in pain lasting up to 12 months.5
Additional orthobiologic options include the use of bone marrow and adipose-derived stem cell (ASC) injections for a variety of knee conditions, including knee OA. Mesenchymal stem cells (MSCs) are multipotent cells that have been used for the treatment of OA in clinical trials because of their regeneration potential and anti-inflammatory effects.6 Bone marrow stem cells (BMSCs) were first used to repair cartilage damage in humans in 1998. However, BMSCs had particular challenges, including low stem cell yield, pain, and possible morbidities during bone marrow aspiration. An alternative is ASCs, which may be more suitable clinically because of the high stem cell yield from lipoaspirates, faster cell proliferation, and less discomfort and morbidities during the harvesting procedure.7 In addition, these adult stem cells can contribute to the chondrogenic, osteogenic, adipogenic, myogenic, and neurogenic lineages.8 One study demonstrated that the contents of cartilage glycosaminoglycans significantly increased in specific areas of a knee joint treated with ASCs.9,10 This increased glycosaminoglycan content in hyaline cartilage may explain the observed visual analog score (VAS) improvement and clinical results. Other studies suggest that the chondrogenic action of ASCs may depend more on regenerative signaling by activated perivascular cells and signaling of trophic and paracrine mediators, such as vascular endothelial growth factor.9,10 Finally, the mechanism of action may include providing volume, support, cushioning, and filling of soft tissue defects.11
The Lipogems method and device, approved by the U.S. Food and Drug Administration, is used to harvest ASCs, cleanse, and micro-fracture adipose tissue while maintaining the perivascular niche that contains pericytes. The purpose of this study was to evaluate the safety and efficacy of using autologous, micro-fractured, minimally manipulated adipose tissue in patients with severe refractory knee OA.
Continue to: This report details...
STUDY PRESENTATION
This report details the outcome of an IRB-approved study of 17 subjects with 26 symptomatic knees with a history of knee OA (Kellgren–Lawrence grade of 3 or 4) diagnosed by a radiograph. Patient demographics are described in the Table.
TABLE. Patient Demographics | |
Male n (%) | 10 (58.8) |
Age, mean ± SD (range) | 68.27 ± 7.43 |
BMI, mean ± SD (range) | 28.98 ± 4.50 |
Kellgren–Lawrence grade 3 (n) | 7 |
Kellgren–Lawrence grade 4 (n) | 19 |
Abbreviation: BMI, body mass index.
The study patients were evaluated by an orthopedic surgeon, Mitchell Sheinkop, who commonly performs total joint replacement in his practice and considers potential patients as candidates for TKA. These patients presented with a Kellgren-Lawrence grade of 3 or 4 knee OA, and all had significant pain that was refractory to conservative treatment, which included medications, physical therapy, and injections. The study patients were offered the Lipogems procedure as an alternative to TKA. Following this procedure, the study subjects were clinically evaluated using the numerical pain rating scale (NPRS), the 100-point Knee Society Score (KSS) with its functional component (FXN), and the lower extremity activity scale (LEAS) at 6 weeks, 6 months, and 12 months. The 1989 KSS12 was used for this study. Adverse reactions were also monitored throughout the study period.
METHODS
After obtaining informed consent, the subjects were taken into the operating room, moved to the procedure table, and placed in the prone position for aspiration. After scrubbing with Betadine and draping, 1 mL of lidocaine was used to anesthetize the skin, and a pre-prepared preparation of lidocaine, epinephrine, and sterile saline was infused into the subcutaneous tissue. The micro-fragmented adipose tissue was obtained with minimal manipulation using Lipogems, a closed system using mild mechanical forces and reduction filters. The system processes the lipoaspirate without the addition of enzymes or any other additives. The final product consists of adipose tissue clusters with preserved vascular stromal niche of approximately 500 microns. The lipoaspirate was processed in the same room via a closed system. During the processing, the subject’s puncture wounds were dressed. The knee injection site was prepped with a Betadine swab and DuraPrep. Then, Lipogems was injected intra-articularly under ultrasound guidance.
After the completion of the injection, manual range of motion was administered to the treated joint. The subject was then transferred to the recovery room where vital signs were monitored. Post-procedure instructions were reviewed with the patient by the study staff. The subject was instructed to use an assistive device and avoid weight-bearing for 48 hours and maintain the activities of daily living to a minimum on the day of the procedure. Non-weight-bearing for 48 hours was recommended for reducing discomfort to avoid the use of opioids. Nonsteroidal anti-inflammatory drugs, alcohol, and marijuana must be avoided for 4 weeks after the procedure. Pretreatment and post-treatment outcomes were collected using the NPRS, the 100-point KSS with its FXN, and the LEAS at 6 weeks, 6 months, and 12 months after this procedure. The 1989 KSS12 was used for this study since the same scale was used for previous TKA procedures by our authors, allowing for future comparisons of results.
STATISTICAL ANALYSIS
Mean and standard deviation were used to estimate central tendency and variability. Outcome measures were analyzed using the t test, with the pairwise t test was used for paired and subsequent measurements of the same patient or a knee. All analyses were performed with significance set at P <.05. The minimal clinically important difference (MCID) in patients who underwent TKA for primary OA was between 5.3 and 5.9 for KSS, while the MCID for FXN was between 6.1 and 6.4.13 These values were referenced for our analysis.
Continue to: No significant adverse...
RESULTS
No significant adverse events were reported in the subjects of this study. Common minor adverse events included pain and swelling, which generally resolved in 48 to 72 hours after the procedure.
Compared with baseline, significant improvements were noted in the mean values of NPRS (Figure 1) at 6 weeks, 6 months, and 12 months. The mean KSS significantly improved from baseline at 6 weeks and 12 months (Figure 2). Significant improvements were also noted in the mean values of FXN (Figure 3) and the mean LEAS significantly improved from baseline at 6 weeks and 6 months (Figure 4).
DISCUSSION
Knee OA is a disabling condition that affects a substantial proportion of the aging population. The current treatment methods do little to address the degenerative environment of the joint, which includes cytokines such as IL-1 and IL-2. Orthobiologic agents have been used recently to address these issues, which include PRP and MSCs from various sources, including bone marrow and adipose tissue.
A recent meta-analysis conducted by Cui and colleagues14 evaluated 18 studies of MSC treatment for knee OA with a total of 565 participants (226 males and 339 females). The duration from the onset of knee pain to registration in each study ranged from 3 months to ≥7 years. The follow-up period was 3 months -24 months. The majority of studies recruited patients with knee OA with a severity grade of 1-4 on the K-L scale; K-L grades 1 and 2 and grades 3 and 4 were defined as early OA and advanced OA, respectively. The results suggested that MSC treatment significantly improved pain and functional status, relative to the baseline evaluations in knee OA, and the beneficial effect was maintained for 2 years after treatment. Furthermore, the treatment effectiveness was not reduced over time.14
Included in the abovementioned meta-analysis were 2 papers by Koh and colleagues in 2012 and 2013 on the use of AMSCs for the treatment of OA. 15,16 The first study included 18 patients whose adipose tissue was harvested from the inner side of the infrapatellar fat pad via a skin incision after arthroscopic debridement. The cells were centrifuged and injected into the patient’s knee the same day. The results showed a significant reduction of pain and an increased quality of life for all patients, and a positive correlation was found between the number of cells injected and pain improvements. The authors concluded that AMSCs were a valid cell source for treating cartilage damage.15
In their second study, Koh and colleagues reported their results of treating 30 elderly patients with OA (≥65 years), who had failed conventional treatment, using intra-articular injections of AMSCs.16 This patient population is important since OA most commonly occurs in the elderly population. Patients underwent arthroscopic lavage and cartilage evaluation before receiving an injection of AMSCs delivered in PRP. The authors demonstrated that AMSC therapy for elderly patients with mild to moderate OA was an effective treatment resulting in reduction of pain and regeneration of cartilage.16
In another study, Adriani and colleagues17 performed autologous percutaneous fat injection from January 2012 to March 2015 for the treatment of knee OA. Their 30 patients (12 males and 18 females; mean age of 63.3 years; mean body mass index of 25.1) had stable or progressive knee OA for at least 12 months, no other injection treatments during the previous 12 months, and no prior knee surgeries. The patients were evaluated at baseline and 1 week and at 1, 3, 6, and 12 months after treatment using the NPRS and the Western Ontario and McMaster Universities Osteoarthritis Index (WOMAC) as outcome measures. The average VAS was 7.7 at baseline and improved to 4.3 at 3-month follow-up; however, a slight deterioration (VAS 5.0) was noted at 1 year. Total WOMAC score was 89.9 at baseline, 68.6 at 3 months, and 73.2 at 12-month follow-up.17
Continue to: The results of...
The results of this study demonstrated significant improvements in pain, quality of life, and function at 12 months after ultrasound-guided injection of ASCs in patients with severe knee OA. Significant improvement that was noted at 6 weeks was maintained through 12 months after the treatment. Improvement was noted in all scales, including the NPRS, the KSS, and the FXN beginning at 3 months and continuing through 12 months. The LEAS was statistically significant through 6 months after the treatment but not significant at 12 months. No serious adverse events were recorded.
In a study by Lee and colleagues,13 the MCID was described for KSS and FXN in patients who underwent TKA for primary OA. This is the minimal change in a scoring measure that is perceived by the patient to be beneficial or harmful. The MCID for KSS was noted to be between 5.3 and 5.9, while the MCID for FXN was between 6.1 and 6.4.13 In our study, the KSS score improved from an average of 74.0 at baseline to 79.6 at 6 months and 81.6 at 12 months (a difference of 5.6 and 7.6; P = .18 and.014, respectively). The FXN improved from an average of 65.4 at baseline to 75.2 at 6 months and 76.4 at 12 months (a difference of 9.9 and 11; P = .041 and.014, respectively). Therefore, a clinically important difference of KSS and FXN scores was noted at both 6 and 12 months.
The technique used in this study provides autologous, minimally manipulated, fat graft performed in a short time (60-90 minutes), without expansion and/or enzymatic treatment. In addition, the harvesting and the injection of stem cells on the same day is a simple, office-based procedure, and compliant with the U. S. Food and Drug Administration regulations.18 The cost of the procedure averages $3500.
A study limitation is that it is a case series with relatively small numbers and not a randomized controlled study. Therefore, a placebo effect may play a role in our results. Further study with a larger number of patients and randomized controlled studies would be beneficial to support the findings of this study.
CONCLUSION
The injection of autologous, micro-fractured, minimally manipulated adipose tissue appears to be a safe and effective treatment option in patients with refractory severe (grade 3 or 4) knee OA. This study showed significant improvements in pain, quality of life, and function for at least 12 months in this study population. This intervention may represent a nonsurgical treatment option to avoid knee joint replacement in this population; however, further investigation is needed.
- Yubo M, Yanyan L, Li L, Tao S, Bo L, Lin C. Clinical efficacy and safety of mesenchymal stem cell transplantation for osteoarthritis treatment: A meta-analysis. PLoS One. 2017;12(4):e0175449.
- Jauregui JJ, Cherian JJ, Pierce TP, Beaver WB, Issa K, Mont MA. Long-Term Survivorship and Clinical Outcomes Following Total Knee Arthroplasty. J Arthroplasty. 2015;30(12):2164-2166.
- Bourne RB, Chesworth BM, Davis AM, Mahomed NN, Charron KD. Patient satisfaction after total knee arthroplasty: who is satisfied and who is not? Clin Orthop Relat Res. 2010;468(1):57-63.
- Dai W-L, Zhou A-G, Zhang H, Zhang J. Efficacy of Platelet-Rich Plasma in the Treatment of Knee Osteoarthritis: A Meta-analysis of Randomized Controlled Trials. Arthroscopy.33(3):659-670.e651.
- Halpern B CS, Rodeo SA, Hayter C, Bogner E, Potter HG, Nguyen J. Clinical and MRI outcomes after platelet-rich plasma treatment for knee osteoarthritis. Clin J Sport Med. 2013 May;23.
- Mamidi MK, Das AK, Zakaria Z, Bhonde R. Mesenchymal stromal cells for cartilage repair in osteoarthritis. Osteoarthritis Cartilage. 2016;24(8):1307-1316.
- Tang Y, Pan ZY, Zou Y, et al. A comparative assessment of adipose-derived stem cells from subcutaneous and visceral fat as a potential cell source for knee osteoarthritis treatment. J Cell Mol Med. 2017.
- Izadpanah R, Trygg C, Patel B, et al. Biologic properties of mesenchymal stem cells derived from bone marrow and adipose tissue. Journal of cellular biochemistry. 2006;99(5):1285-1297.
- Ankrum J, Karp JM. Mesenchymal stem cell therapy: Two steps forward, one step back. Trends Mol Med. 2010;16(5):203-209.
- Togel F, Weiss K, Yang Y, Hu Z, Zhang P, Westenfelder C. Vasculotropic, paracrine actions of infused mesenchymal stem cells are important to the recovery from acute kidney injury. A J Physiol Renal Physiol. 2007;292(5):F1626-1635.
- Mestak O, Sukop A, Hsueh YS, et al. Centrifugation versus PureGraft for fatgrafting to the breast after breast-conserving therapy. World J Surg Oncol. 2014;12:178.
- Insall JN DL, Scott RD, Scott WN. Rationale of the Knee Society clinical rating system. Clin Orthop Relat Res. 1989 Nov;(248):13-4.
- Lee WC, Kwan YH, Chong HC, Yeo SJ. The minimal clinically important difference for Knee Society Clinical Rating System after total knee arthroplasty for primary osteoarthritis. Knee Surgery, Sports Traumatology, Arthroscopy. 2016.
- Cui GH, Wang YY, Li CJ, Shi CH, Wang WS. Efficacy of mesenchymal stem cells in treating patients with osteoarthritis of the knee: A meta-analysis. Exp Ther Med. 2016;12(5):3390-3400.
- Koh Y-GC, Yun-Jin. Infrapatellar fat pad-derived mesenchymal stem cell therapy for knee osteoarthritis. Knee. 2012;19(6):902-907.
- Koh Y-GC, Yun-Jin. Mesenchymal stem cell injections improve symptoms of knee osteoarthritis. Arthroscopy. 2013;29(4):748-755.
- Adriani E. MM, et al. Percutaneous Fat Transfer to Treat Knee Osteoarthritis Symptoms: Preliminary Results. Joints. 2017.
- Bianchi F, Maioli M, Leonardi E, et al. A New Nonenzymatic Method and Device to Obtain a Fat Tissue Derivative Highly Enriched in Pericyte-Like Elements by Mild Mechanical Forces From Human Lipoaspirates. Cell Transplantation. 2013;22(11):2063-2077
ABSTRACT
The aim of this study was to evaluate the safety and efficacy of using autologous, micro-fractured, minimally manipulated adipose tissue in patients with refractory knee osteoarthritis (OA). A total of 17 subjects (26 knees) with a median age of 72 years (range: 54-78 years) and a history of knee OA (Kellgren–Lawrence, grade of 3 or 4) underwent treatment with ultrasound-guided injection of micro-fractured adipose tissue. Micro-fractured fat was obtained using a minimal manipulation technique in a closed system (Lipogems), without the addition of enzymes or any other additives. The study subjects were clinically evaluated using the numerical pain rating scale (NPRS), the 100-point Knee Society Score (KSS) with its functional component (FXN), and the lower extremity activity scale (LEAS) at 6 weeks, 6 months, and 12 months following this procedure.
When compared with baseline, significant improvements were noted in the mean values of NPRS, FXN, and LEAS at 6 weeks, 6 months, and 12 months. The mean KSS significantly improved at 6 weeks and 12 months. In particular, the average KSS score improved from 74 to 82, the FXN score improved from 65 to 76, and the LEAS score improved from 36 to 47. These values were significantly greater than the previously published minimal clinically important difference described for KSS and FXN in patients who underwent total knee arthroplasty for primary OA. No serious adverse events were reported. The injection of autologous, micro-fractured, minimally manipulated adipose tissue appears to be a safe and effective treatment option for patients with refractory, severe (grade 3 or 4) knee OA.
This study demonstrated significant improvements in pain, quality of life, and function for at least 12 months in this study population. This intervention may represent a nonsurgical treatment option to avoid knee joint replacement in this population; however, further investigation is needed.
Continue to: Knee OA is...
Knee OA is a chronic disease that affects all races, genders, and ages, but it is most prevalent in obese and elderly people. Worldwide, arthritis is considered to be the fourth leading cause of disability.1 In developing and developed countries, knee OA may cause a significant decline in the quality of life for individuals >65 years due to joint pain and disability.1 Nonoperative treatment can be successful in patients with mild to moderate arthritis with pain.
Current treatment options for knee OA, including physical therapy and anti-inflammatory drugs, aim to remedy the symptoms, but they do little to treat the underlying causes of knee OA pain. When a patient presents with advanced arthritis of the knee as confirmed by radiographic findings (classified as Kellgren–Lawrence grade of 3 or 4), the standard approach has been a total knee arthroplasty (TKA) after the patient has failed conservative treatment. The annual rate of total knee replacement in the United States has doubled since 2000, especially in those 45 – 65 years.2 The total number of procedures performed each year now exceeds 640,000, at a total annual cost of about $10.2 billion.2 Multiple studies show that TKA has favorable outcomes in pain relief and functional improvement in patients >60 years when evaluated at a follow-up of 10 years after surgery.2
However, some patients are hesitant to proceed with surgery due to fear of surgical pain and procedural complications. The known complications include deep vein thrombosis, pulmonary embolism, nerve injury, and infection. In addition, up to 20% of patients continue to complain of pain following a total knee replacement.3 Finally, in the young population (<50 years), there are concerns related to the potential need of revision knee surgery in the future.3
Alternative treatments for knee OA have recently emerged, including the use of platelet-rich plasma (PRP). A recent meta-analysis that included 10 randomized controlled trials with a total of 1069 patients demonstrated that, compared with hyaluronic acid and saline, intra-articular PRP injection may have more benefits in pain relief and functional improvement in patients with symptomatic knee OA at 1-year post-injection.4 Another smaller study examined patients who had experienced mild knee OA (Kellgren–Lawrence grade <3) for an average of 14 months. Each patient underwent magnetic resonance imaging for the evaluation of joint damage and then received a single PRP injection. The patients were assessed at regular intervals, with improvement in pain lasting up to 12 months.5
Additional orthobiologic options include the use of bone marrow and adipose-derived stem cell (ASC) injections for a variety of knee conditions, including knee OA. Mesenchymal stem cells (MSCs) are multipotent cells that have been used for the treatment of OA in clinical trials because of their regeneration potential and anti-inflammatory effects.6 Bone marrow stem cells (BMSCs) were first used to repair cartilage damage in humans in 1998. However, BMSCs had particular challenges, including low stem cell yield, pain, and possible morbidities during bone marrow aspiration. An alternative is ASCs, which may be more suitable clinically because of the high stem cell yield from lipoaspirates, faster cell proliferation, and less discomfort and morbidities during the harvesting procedure.7 In addition, these adult stem cells can contribute to the chondrogenic, osteogenic, adipogenic, myogenic, and neurogenic lineages.8 One study demonstrated that the contents of cartilage glycosaminoglycans significantly increased in specific areas of a knee joint treated with ASCs.9,10 This increased glycosaminoglycan content in hyaline cartilage may explain the observed visual analog score (VAS) improvement and clinical results. Other studies suggest that the chondrogenic action of ASCs may depend more on regenerative signaling by activated perivascular cells and signaling of trophic and paracrine mediators, such as vascular endothelial growth factor.9,10 Finally, the mechanism of action may include providing volume, support, cushioning, and filling of soft tissue defects.11
The Lipogems method and device, approved by the U.S. Food and Drug Administration, is used to harvest ASCs, cleanse, and micro-fracture adipose tissue while maintaining the perivascular niche that contains pericytes. The purpose of this study was to evaluate the safety and efficacy of using autologous, micro-fractured, minimally manipulated adipose tissue in patients with severe refractory knee OA.
Continue to: This report details...
STUDY PRESENTATION
This report details the outcome of an IRB-approved study of 17 subjects with 26 symptomatic knees with a history of knee OA (Kellgren–Lawrence grade of 3 or 4) diagnosed by a radiograph. Patient demographics are described in the Table.
TABLE. Patient Demographics | |
Male n (%) | 10 (58.8) |
Age, mean ± SD (range) | 68.27 ± 7.43 |
BMI, mean ± SD (range) | 28.98 ± 4.50 |
Kellgren–Lawrence grade 3 (n) | 7 |
Kellgren–Lawrence grade 4 (n) | 19 |
Abbreviation: BMI, body mass index.
The study patients were evaluated by an orthopedic surgeon, Mitchell Sheinkop, who commonly performs total joint replacement in his practice and considers potential patients as candidates for TKA. These patients presented with a Kellgren-Lawrence grade of 3 or 4 knee OA, and all had significant pain that was refractory to conservative treatment, which included medications, physical therapy, and injections. The study patients were offered the Lipogems procedure as an alternative to TKA. Following this procedure, the study subjects were clinically evaluated using the numerical pain rating scale (NPRS), the 100-point Knee Society Score (KSS) with its functional component (FXN), and the lower extremity activity scale (LEAS) at 6 weeks, 6 months, and 12 months. The 1989 KSS12 was used for this study. Adverse reactions were also monitored throughout the study period.
METHODS
After obtaining informed consent, the subjects were taken into the operating room, moved to the procedure table, and placed in the prone position for aspiration. After scrubbing with Betadine and draping, 1 mL of lidocaine was used to anesthetize the skin, and a pre-prepared preparation of lidocaine, epinephrine, and sterile saline was infused into the subcutaneous tissue. The micro-fragmented adipose tissue was obtained with minimal manipulation using Lipogems, a closed system using mild mechanical forces and reduction filters. The system processes the lipoaspirate without the addition of enzymes or any other additives. The final product consists of adipose tissue clusters with preserved vascular stromal niche of approximately 500 microns. The lipoaspirate was processed in the same room via a closed system. During the processing, the subject’s puncture wounds were dressed. The knee injection site was prepped with a Betadine swab and DuraPrep. Then, Lipogems was injected intra-articularly under ultrasound guidance.
After the completion of the injection, manual range of motion was administered to the treated joint. The subject was then transferred to the recovery room where vital signs were monitored. Post-procedure instructions were reviewed with the patient by the study staff. The subject was instructed to use an assistive device and avoid weight-bearing for 48 hours and maintain the activities of daily living to a minimum on the day of the procedure. Non-weight-bearing for 48 hours was recommended for reducing discomfort to avoid the use of opioids. Nonsteroidal anti-inflammatory drugs, alcohol, and marijuana must be avoided for 4 weeks after the procedure. Pretreatment and post-treatment outcomes were collected using the NPRS, the 100-point KSS with its FXN, and the LEAS at 6 weeks, 6 months, and 12 months after this procedure. The 1989 KSS12 was used for this study since the same scale was used for previous TKA procedures by our authors, allowing for future comparisons of results.
STATISTICAL ANALYSIS
Mean and standard deviation were used to estimate central tendency and variability. Outcome measures were analyzed using the t test, with the pairwise t test was used for paired and subsequent measurements of the same patient or a knee. All analyses were performed with significance set at P <.05. The minimal clinically important difference (MCID) in patients who underwent TKA for primary OA was between 5.3 and 5.9 for KSS, while the MCID for FXN was between 6.1 and 6.4.13 These values were referenced for our analysis.
Continue to: No significant adverse...
RESULTS
No significant adverse events were reported in the subjects of this study. Common minor adverse events included pain and swelling, which generally resolved in 48 to 72 hours after the procedure.
Compared with baseline, significant improvements were noted in the mean values of NPRS (Figure 1) at 6 weeks, 6 months, and 12 months. The mean KSS significantly improved from baseline at 6 weeks and 12 months (Figure 2). Significant improvements were also noted in the mean values of FXN (Figure 3) and the mean LEAS significantly improved from baseline at 6 weeks and 6 months (Figure 4).
DISCUSSION
Knee OA is a disabling condition that affects a substantial proportion of the aging population. The current treatment methods do little to address the degenerative environment of the joint, which includes cytokines such as IL-1 and IL-2. Orthobiologic agents have been used recently to address these issues, which include PRP and MSCs from various sources, including bone marrow and adipose tissue.
A recent meta-analysis conducted by Cui and colleagues14 evaluated 18 studies of MSC treatment for knee OA with a total of 565 participants (226 males and 339 females). The duration from the onset of knee pain to registration in each study ranged from 3 months to ≥7 years. The follow-up period was 3 months -24 months. The majority of studies recruited patients with knee OA with a severity grade of 1-4 on the K-L scale; K-L grades 1 and 2 and grades 3 and 4 were defined as early OA and advanced OA, respectively. The results suggested that MSC treatment significantly improved pain and functional status, relative to the baseline evaluations in knee OA, and the beneficial effect was maintained for 2 years after treatment. Furthermore, the treatment effectiveness was not reduced over time.14
Included in the abovementioned meta-analysis were 2 papers by Koh and colleagues in 2012 and 2013 on the use of AMSCs for the treatment of OA. 15,16 The first study included 18 patients whose adipose tissue was harvested from the inner side of the infrapatellar fat pad via a skin incision after arthroscopic debridement. The cells were centrifuged and injected into the patient’s knee the same day. The results showed a significant reduction of pain and an increased quality of life for all patients, and a positive correlation was found between the number of cells injected and pain improvements. The authors concluded that AMSCs were a valid cell source for treating cartilage damage.15
In their second study, Koh and colleagues reported their results of treating 30 elderly patients with OA (≥65 years), who had failed conventional treatment, using intra-articular injections of AMSCs.16 This patient population is important since OA most commonly occurs in the elderly population. Patients underwent arthroscopic lavage and cartilage evaluation before receiving an injection of AMSCs delivered in PRP. The authors demonstrated that AMSC therapy for elderly patients with mild to moderate OA was an effective treatment resulting in reduction of pain and regeneration of cartilage.16
In another study, Adriani and colleagues17 performed autologous percutaneous fat injection from January 2012 to March 2015 for the treatment of knee OA. Their 30 patients (12 males and 18 females; mean age of 63.3 years; mean body mass index of 25.1) had stable or progressive knee OA for at least 12 months, no other injection treatments during the previous 12 months, and no prior knee surgeries. The patients were evaluated at baseline and 1 week and at 1, 3, 6, and 12 months after treatment using the NPRS and the Western Ontario and McMaster Universities Osteoarthritis Index (WOMAC) as outcome measures. The average VAS was 7.7 at baseline and improved to 4.3 at 3-month follow-up; however, a slight deterioration (VAS 5.0) was noted at 1 year. Total WOMAC score was 89.9 at baseline, 68.6 at 3 months, and 73.2 at 12-month follow-up.17
Continue to: The results of...
The results of this study demonstrated significant improvements in pain, quality of life, and function at 12 months after ultrasound-guided injection of ASCs in patients with severe knee OA. Significant improvement that was noted at 6 weeks was maintained through 12 months after the treatment. Improvement was noted in all scales, including the NPRS, the KSS, and the FXN beginning at 3 months and continuing through 12 months. The LEAS was statistically significant through 6 months after the treatment but not significant at 12 months. No serious adverse events were recorded.
In a study by Lee and colleagues,13 the MCID was described for KSS and FXN in patients who underwent TKA for primary OA. This is the minimal change in a scoring measure that is perceived by the patient to be beneficial or harmful. The MCID for KSS was noted to be between 5.3 and 5.9, while the MCID for FXN was between 6.1 and 6.4.13 In our study, the KSS score improved from an average of 74.0 at baseline to 79.6 at 6 months and 81.6 at 12 months (a difference of 5.6 and 7.6; P = .18 and.014, respectively). The FXN improved from an average of 65.4 at baseline to 75.2 at 6 months and 76.4 at 12 months (a difference of 9.9 and 11; P = .041 and.014, respectively). Therefore, a clinically important difference of KSS and FXN scores was noted at both 6 and 12 months.
The technique used in this study provides autologous, minimally manipulated, fat graft performed in a short time (60-90 minutes), without expansion and/or enzymatic treatment. In addition, the harvesting and the injection of stem cells on the same day is a simple, office-based procedure, and compliant with the U. S. Food and Drug Administration regulations.18 The cost of the procedure averages $3500.
A study limitation is that it is a case series with relatively small numbers and not a randomized controlled study. Therefore, a placebo effect may play a role in our results. Further study with a larger number of patients and randomized controlled studies would be beneficial to support the findings of this study.
CONCLUSION
The injection of autologous, micro-fractured, minimally manipulated adipose tissue appears to be a safe and effective treatment option in patients with refractory severe (grade 3 or 4) knee OA. This study showed significant improvements in pain, quality of life, and function for at least 12 months in this study population. This intervention may represent a nonsurgical treatment option to avoid knee joint replacement in this population; however, further investigation is needed.
ABSTRACT
The aim of this study was to evaluate the safety and efficacy of using autologous, micro-fractured, minimally manipulated adipose tissue in patients with refractory knee osteoarthritis (OA). A total of 17 subjects (26 knees) with a median age of 72 years (range: 54-78 years) and a history of knee OA (Kellgren–Lawrence, grade of 3 or 4) underwent treatment with ultrasound-guided injection of micro-fractured adipose tissue. Micro-fractured fat was obtained using a minimal manipulation technique in a closed system (Lipogems), without the addition of enzymes or any other additives. The study subjects were clinically evaluated using the numerical pain rating scale (NPRS), the 100-point Knee Society Score (KSS) with its functional component (FXN), and the lower extremity activity scale (LEAS) at 6 weeks, 6 months, and 12 months following this procedure.
When compared with baseline, significant improvements were noted in the mean values of NPRS, FXN, and LEAS at 6 weeks, 6 months, and 12 months. The mean KSS significantly improved at 6 weeks and 12 months. In particular, the average KSS score improved from 74 to 82, the FXN score improved from 65 to 76, and the LEAS score improved from 36 to 47. These values were significantly greater than the previously published minimal clinically important difference described for KSS and FXN in patients who underwent total knee arthroplasty for primary OA. No serious adverse events were reported. The injection of autologous, micro-fractured, minimally manipulated adipose tissue appears to be a safe and effective treatment option for patients with refractory, severe (grade 3 or 4) knee OA.
This study demonstrated significant improvements in pain, quality of life, and function for at least 12 months in this study population. This intervention may represent a nonsurgical treatment option to avoid knee joint replacement in this population; however, further investigation is needed.
Continue to: Knee OA is...
Knee OA is a chronic disease that affects all races, genders, and ages, but it is most prevalent in obese and elderly people. Worldwide, arthritis is considered to be the fourth leading cause of disability.1 In developing and developed countries, knee OA may cause a significant decline in the quality of life for individuals >65 years due to joint pain and disability.1 Nonoperative treatment can be successful in patients with mild to moderate arthritis with pain.
Current treatment options for knee OA, including physical therapy and anti-inflammatory drugs, aim to remedy the symptoms, but they do little to treat the underlying causes of knee OA pain. When a patient presents with advanced arthritis of the knee as confirmed by radiographic findings (classified as Kellgren–Lawrence grade of 3 or 4), the standard approach has been a total knee arthroplasty (TKA) after the patient has failed conservative treatment. The annual rate of total knee replacement in the United States has doubled since 2000, especially in those 45 – 65 years.2 The total number of procedures performed each year now exceeds 640,000, at a total annual cost of about $10.2 billion.2 Multiple studies show that TKA has favorable outcomes in pain relief and functional improvement in patients >60 years when evaluated at a follow-up of 10 years after surgery.2
However, some patients are hesitant to proceed with surgery due to fear of surgical pain and procedural complications. The known complications include deep vein thrombosis, pulmonary embolism, nerve injury, and infection. In addition, up to 20% of patients continue to complain of pain following a total knee replacement.3 Finally, in the young population (<50 years), there are concerns related to the potential need of revision knee surgery in the future.3
Alternative treatments for knee OA have recently emerged, including the use of platelet-rich plasma (PRP). A recent meta-analysis that included 10 randomized controlled trials with a total of 1069 patients demonstrated that, compared with hyaluronic acid and saline, intra-articular PRP injection may have more benefits in pain relief and functional improvement in patients with symptomatic knee OA at 1-year post-injection.4 Another smaller study examined patients who had experienced mild knee OA (Kellgren–Lawrence grade <3) for an average of 14 months. Each patient underwent magnetic resonance imaging for the evaluation of joint damage and then received a single PRP injection. The patients were assessed at regular intervals, with improvement in pain lasting up to 12 months.5
Additional orthobiologic options include the use of bone marrow and adipose-derived stem cell (ASC) injections for a variety of knee conditions, including knee OA. Mesenchymal stem cells (MSCs) are multipotent cells that have been used for the treatment of OA in clinical trials because of their regeneration potential and anti-inflammatory effects.6 Bone marrow stem cells (BMSCs) were first used to repair cartilage damage in humans in 1998. However, BMSCs had particular challenges, including low stem cell yield, pain, and possible morbidities during bone marrow aspiration. An alternative is ASCs, which may be more suitable clinically because of the high stem cell yield from lipoaspirates, faster cell proliferation, and less discomfort and morbidities during the harvesting procedure.7 In addition, these adult stem cells can contribute to the chondrogenic, osteogenic, adipogenic, myogenic, and neurogenic lineages.8 One study demonstrated that the contents of cartilage glycosaminoglycans significantly increased in specific areas of a knee joint treated with ASCs.9,10 This increased glycosaminoglycan content in hyaline cartilage may explain the observed visual analog score (VAS) improvement and clinical results. Other studies suggest that the chondrogenic action of ASCs may depend more on regenerative signaling by activated perivascular cells and signaling of trophic and paracrine mediators, such as vascular endothelial growth factor.9,10 Finally, the mechanism of action may include providing volume, support, cushioning, and filling of soft tissue defects.11
The Lipogems method and device, approved by the U.S. Food and Drug Administration, is used to harvest ASCs, cleanse, and micro-fracture adipose tissue while maintaining the perivascular niche that contains pericytes. The purpose of this study was to evaluate the safety and efficacy of using autologous, micro-fractured, minimally manipulated adipose tissue in patients with severe refractory knee OA.
Continue to: This report details...
STUDY PRESENTATION
This report details the outcome of an IRB-approved study of 17 subjects with 26 symptomatic knees with a history of knee OA (Kellgren–Lawrence grade of 3 or 4) diagnosed by a radiograph. Patient demographics are described in the Table.
TABLE. Patient Demographics | |
Male n (%) | 10 (58.8) |
Age, mean ± SD (range) | 68.27 ± 7.43 |
BMI, mean ± SD (range) | 28.98 ± 4.50 |
Kellgren–Lawrence grade 3 (n) | 7 |
Kellgren–Lawrence grade 4 (n) | 19 |
Abbreviation: BMI, body mass index.
The study patients were evaluated by an orthopedic surgeon, Mitchell Sheinkop, who commonly performs total joint replacement in his practice and considers potential patients as candidates for TKA. These patients presented with a Kellgren-Lawrence grade of 3 or 4 knee OA, and all had significant pain that was refractory to conservative treatment, which included medications, physical therapy, and injections. The study patients were offered the Lipogems procedure as an alternative to TKA. Following this procedure, the study subjects were clinically evaluated using the numerical pain rating scale (NPRS), the 100-point Knee Society Score (KSS) with its functional component (FXN), and the lower extremity activity scale (LEAS) at 6 weeks, 6 months, and 12 months. The 1989 KSS12 was used for this study. Adverse reactions were also monitored throughout the study period.
METHODS
After obtaining informed consent, the subjects were taken into the operating room, moved to the procedure table, and placed in the prone position for aspiration. After scrubbing with Betadine and draping, 1 mL of lidocaine was used to anesthetize the skin, and a pre-prepared preparation of lidocaine, epinephrine, and sterile saline was infused into the subcutaneous tissue. The micro-fragmented adipose tissue was obtained with minimal manipulation using Lipogems, a closed system using mild mechanical forces and reduction filters. The system processes the lipoaspirate without the addition of enzymes or any other additives. The final product consists of adipose tissue clusters with preserved vascular stromal niche of approximately 500 microns. The lipoaspirate was processed in the same room via a closed system. During the processing, the subject’s puncture wounds were dressed. The knee injection site was prepped with a Betadine swab and DuraPrep. Then, Lipogems was injected intra-articularly under ultrasound guidance.
After the completion of the injection, manual range of motion was administered to the treated joint. The subject was then transferred to the recovery room where vital signs were monitored. Post-procedure instructions were reviewed with the patient by the study staff. The subject was instructed to use an assistive device and avoid weight-bearing for 48 hours and maintain the activities of daily living to a minimum on the day of the procedure. Non-weight-bearing for 48 hours was recommended for reducing discomfort to avoid the use of opioids. Nonsteroidal anti-inflammatory drugs, alcohol, and marijuana must be avoided for 4 weeks after the procedure. Pretreatment and post-treatment outcomes were collected using the NPRS, the 100-point KSS with its FXN, and the LEAS at 6 weeks, 6 months, and 12 months after this procedure. The 1989 KSS12 was used for this study since the same scale was used for previous TKA procedures by our authors, allowing for future comparisons of results.
STATISTICAL ANALYSIS
Mean and standard deviation were used to estimate central tendency and variability. Outcome measures were analyzed using the t test, with the pairwise t test was used for paired and subsequent measurements of the same patient or a knee. All analyses were performed with significance set at P <.05. The minimal clinically important difference (MCID) in patients who underwent TKA for primary OA was between 5.3 and 5.9 for KSS, while the MCID for FXN was between 6.1 and 6.4.13 These values were referenced for our analysis.
Continue to: No significant adverse...
RESULTS
No significant adverse events were reported in the subjects of this study. Common minor adverse events included pain and swelling, which generally resolved in 48 to 72 hours after the procedure.
Compared with baseline, significant improvements were noted in the mean values of NPRS (Figure 1) at 6 weeks, 6 months, and 12 months. The mean KSS significantly improved from baseline at 6 weeks and 12 months (Figure 2). Significant improvements were also noted in the mean values of FXN (Figure 3) and the mean LEAS significantly improved from baseline at 6 weeks and 6 months (Figure 4).
DISCUSSION
Knee OA is a disabling condition that affects a substantial proportion of the aging population. The current treatment methods do little to address the degenerative environment of the joint, which includes cytokines such as IL-1 and IL-2. Orthobiologic agents have been used recently to address these issues, which include PRP and MSCs from various sources, including bone marrow and adipose tissue.
A recent meta-analysis conducted by Cui and colleagues14 evaluated 18 studies of MSC treatment for knee OA with a total of 565 participants (226 males and 339 females). The duration from the onset of knee pain to registration in each study ranged from 3 months to ≥7 years. The follow-up period was 3 months -24 months. The majority of studies recruited patients with knee OA with a severity grade of 1-4 on the K-L scale; K-L grades 1 and 2 and grades 3 and 4 were defined as early OA and advanced OA, respectively. The results suggested that MSC treatment significantly improved pain and functional status, relative to the baseline evaluations in knee OA, and the beneficial effect was maintained for 2 years after treatment. Furthermore, the treatment effectiveness was not reduced over time.14
Included in the abovementioned meta-analysis were 2 papers by Koh and colleagues in 2012 and 2013 on the use of AMSCs for the treatment of OA. 15,16 The first study included 18 patients whose adipose tissue was harvested from the inner side of the infrapatellar fat pad via a skin incision after arthroscopic debridement. The cells were centrifuged and injected into the patient’s knee the same day. The results showed a significant reduction of pain and an increased quality of life for all patients, and a positive correlation was found between the number of cells injected and pain improvements. The authors concluded that AMSCs were a valid cell source for treating cartilage damage.15
In their second study, Koh and colleagues reported their results of treating 30 elderly patients with OA (≥65 years), who had failed conventional treatment, using intra-articular injections of AMSCs.16 This patient population is important since OA most commonly occurs in the elderly population. Patients underwent arthroscopic lavage and cartilage evaluation before receiving an injection of AMSCs delivered in PRP. The authors demonstrated that AMSC therapy for elderly patients with mild to moderate OA was an effective treatment resulting in reduction of pain and regeneration of cartilage.16
In another study, Adriani and colleagues17 performed autologous percutaneous fat injection from January 2012 to March 2015 for the treatment of knee OA. Their 30 patients (12 males and 18 females; mean age of 63.3 years; mean body mass index of 25.1) had stable or progressive knee OA for at least 12 months, no other injection treatments during the previous 12 months, and no prior knee surgeries. The patients were evaluated at baseline and 1 week and at 1, 3, 6, and 12 months after treatment using the NPRS and the Western Ontario and McMaster Universities Osteoarthritis Index (WOMAC) as outcome measures. The average VAS was 7.7 at baseline and improved to 4.3 at 3-month follow-up; however, a slight deterioration (VAS 5.0) was noted at 1 year. Total WOMAC score was 89.9 at baseline, 68.6 at 3 months, and 73.2 at 12-month follow-up.17
Continue to: The results of...
The results of this study demonstrated significant improvements in pain, quality of life, and function at 12 months after ultrasound-guided injection of ASCs in patients with severe knee OA. Significant improvement that was noted at 6 weeks was maintained through 12 months after the treatment. Improvement was noted in all scales, including the NPRS, the KSS, and the FXN beginning at 3 months and continuing through 12 months. The LEAS was statistically significant through 6 months after the treatment but not significant at 12 months. No serious adverse events were recorded.
In a study by Lee and colleagues,13 the MCID was described for KSS and FXN in patients who underwent TKA for primary OA. This is the minimal change in a scoring measure that is perceived by the patient to be beneficial or harmful. The MCID for KSS was noted to be between 5.3 and 5.9, while the MCID for FXN was between 6.1 and 6.4.13 In our study, the KSS score improved from an average of 74.0 at baseline to 79.6 at 6 months and 81.6 at 12 months (a difference of 5.6 and 7.6; P = .18 and.014, respectively). The FXN improved from an average of 65.4 at baseline to 75.2 at 6 months and 76.4 at 12 months (a difference of 9.9 and 11; P = .041 and.014, respectively). Therefore, a clinically important difference of KSS and FXN scores was noted at both 6 and 12 months.
The technique used in this study provides autologous, minimally manipulated, fat graft performed in a short time (60-90 minutes), without expansion and/or enzymatic treatment. In addition, the harvesting and the injection of stem cells on the same day is a simple, office-based procedure, and compliant with the U. S. Food and Drug Administration regulations.18 The cost of the procedure averages $3500.
A study limitation is that it is a case series with relatively small numbers and not a randomized controlled study. Therefore, a placebo effect may play a role in our results. Further study with a larger number of patients and randomized controlled studies would be beneficial to support the findings of this study.
CONCLUSION
The injection of autologous, micro-fractured, minimally manipulated adipose tissue appears to be a safe and effective treatment option in patients with refractory severe (grade 3 or 4) knee OA. This study showed significant improvements in pain, quality of life, and function for at least 12 months in this study population. This intervention may represent a nonsurgical treatment option to avoid knee joint replacement in this population; however, further investigation is needed.
- Yubo M, Yanyan L, Li L, Tao S, Bo L, Lin C. Clinical efficacy and safety of mesenchymal stem cell transplantation for osteoarthritis treatment: A meta-analysis. PLoS One. 2017;12(4):e0175449.
- Jauregui JJ, Cherian JJ, Pierce TP, Beaver WB, Issa K, Mont MA. Long-Term Survivorship and Clinical Outcomes Following Total Knee Arthroplasty. J Arthroplasty. 2015;30(12):2164-2166.
- Bourne RB, Chesworth BM, Davis AM, Mahomed NN, Charron KD. Patient satisfaction after total knee arthroplasty: who is satisfied and who is not? Clin Orthop Relat Res. 2010;468(1):57-63.
- Dai W-L, Zhou A-G, Zhang H, Zhang J. Efficacy of Platelet-Rich Plasma in the Treatment of Knee Osteoarthritis: A Meta-analysis of Randomized Controlled Trials. Arthroscopy.33(3):659-670.e651.
- Halpern B CS, Rodeo SA, Hayter C, Bogner E, Potter HG, Nguyen J. Clinical and MRI outcomes after platelet-rich plasma treatment for knee osteoarthritis. Clin J Sport Med. 2013 May;23.
- Mamidi MK, Das AK, Zakaria Z, Bhonde R. Mesenchymal stromal cells for cartilage repair in osteoarthritis. Osteoarthritis Cartilage. 2016;24(8):1307-1316.
- Tang Y, Pan ZY, Zou Y, et al. A comparative assessment of adipose-derived stem cells from subcutaneous and visceral fat as a potential cell source for knee osteoarthritis treatment. J Cell Mol Med. 2017.
- Izadpanah R, Trygg C, Patel B, et al. Biologic properties of mesenchymal stem cells derived from bone marrow and adipose tissue. Journal of cellular biochemistry. 2006;99(5):1285-1297.
- Ankrum J, Karp JM. Mesenchymal stem cell therapy: Two steps forward, one step back. Trends Mol Med. 2010;16(5):203-209.
- Togel F, Weiss K, Yang Y, Hu Z, Zhang P, Westenfelder C. Vasculotropic, paracrine actions of infused mesenchymal stem cells are important to the recovery from acute kidney injury. A J Physiol Renal Physiol. 2007;292(5):F1626-1635.
- Mestak O, Sukop A, Hsueh YS, et al. Centrifugation versus PureGraft for fatgrafting to the breast after breast-conserving therapy. World J Surg Oncol. 2014;12:178.
- Insall JN DL, Scott RD, Scott WN. Rationale of the Knee Society clinical rating system. Clin Orthop Relat Res. 1989 Nov;(248):13-4.
- Lee WC, Kwan YH, Chong HC, Yeo SJ. The minimal clinically important difference for Knee Society Clinical Rating System after total knee arthroplasty for primary osteoarthritis. Knee Surgery, Sports Traumatology, Arthroscopy. 2016.
- Cui GH, Wang YY, Li CJ, Shi CH, Wang WS. Efficacy of mesenchymal stem cells in treating patients with osteoarthritis of the knee: A meta-analysis. Exp Ther Med. 2016;12(5):3390-3400.
- Koh Y-GC, Yun-Jin. Infrapatellar fat pad-derived mesenchymal stem cell therapy for knee osteoarthritis. Knee. 2012;19(6):902-907.
- Koh Y-GC, Yun-Jin. Mesenchymal stem cell injections improve symptoms of knee osteoarthritis. Arthroscopy. 2013;29(4):748-755.
- Adriani E. MM, et al. Percutaneous Fat Transfer to Treat Knee Osteoarthritis Symptoms: Preliminary Results. Joints. 2017.
- Bianchi F, Maioli M, Leonardi E, et al. A New Nonenzymatic Method and Device to Obtain a Fat Tissue Derivative Highly Enriched in Pericyte-Like Elements by Mild Mechanical Forces From Human Lipoaspirates. Cell Transplantation. 2013;22(11):2063-2077
- Yubo M, Yanyan L, Li L, Tao S, Bo L, Lin C. Clinical efficacy and safety of mesenchymal stem cell transplantation for osteoarthritis treatment: A meta-analysis. PLoS One. 2017;12(4):e0175449.
- Jauregui JJ, Cherian JJ, Pierce TP, Beaver WB, Issa K, Mont MA. Long-Term Survivorship and Clinical Outcomes Following Total Knee Arthroplasty. J Arthroplasty. 2015;30(12):2164-2166.
- Bourne RB, Chesworth BM, Davis AM, Mahomed NN, Charron KD. Patient satisfaction after total knee arthroplasty: who is satisfied and who is not? Clin Orthop Relat Res. 2010;468(1):57-63.
- Dai W-L, Zhou A-G, Zhang H, Zhang J. Efficacy of Platelet-Rich Plasma in the Treatment of Knee Osteoarthritis: A Meta-analysis of Randomized Controlled Trials. Arthroscopy.33(3):659-670.e651.
- Halpern B CS, Rodeo SA, Hayter C, Bogner E, Potter HG, Nguyen J. Clinical and MRI outcomes after platelet-rich plasma treatment for knee osteoarthritis. Clin J Sport Med. 2013 May;23.
- Mamidi MK, Das AK, Zakaria Z, Bhonde R. Mesenchymal stromal cells for cartilage repair in osteoarthritis. Osteoarthritis Cartilage. 2016;24(8):1307-1316.
- Tang Y, Pan ZY, Zou Y, et al. A comparative assessment of adipose-derived stem cells from subcutaneous and visceral fat as a potential cell source for knee osteoarthritis treatment. J Cell Mol Med. 2017.
- Izadpanah R, Trygg C, Patel B, et al. Biologic properties of mesenchymal stem cells derived from bone marrow and adipose tissue. Journal of cellular biochemistry. 2006;99(5):1285-1297.
- Ankrum J, Karp JM. Mesenchymal stem cell therapy: Two steps forward, one step back. Trends Mol Med. 2010;16(5):203-209.
- Togel F, Weiss K, Yang Y, Hu Z, Zhang P, Westenfelder C. Vasculotropic, paracrine actions of infused mesenchymal stem cells are important to the recovery from acute kidney injury. A J Physiol Renal Physiol. 2007;292(5):F1626-1635.
- Mestak O, Sukop A, Hsueh YS, et al. Centrifugation versus PureGraft for fatgrafting to the breast after breast-conserving therapy. World J Surg Oncol. 2014;12:178.
- Insall JN DL, Scott RD, Scott WN. Rationale of the Knee Society clinical rating system. Clin Orthop Relat Res. 1989 Nov;(248):13-4.
- Lee WC, Kwan YH, Chong HC, Yeo SJ. The minimal clinically important difference for Knee Society Clinical Rating System after total knee arthroplasty for primary osteoarthritis. Knee Surgery, Sports Traumatology, Arthroscopy. 2016.
- Cui GH, Wang YY, Li CJ, Shi CH, Wang WS. Efficacy of mesenchymal stem cells in treating patients with osteoarthritis of the knee: A meta-analysis. Exp Ther Med. 2016;12(5):3390-3400.
- Koh Y-GC, Yun-Jin. Infrapatellar fat pad-derived mesenchymal stem cell therapy for knee osteoarthritis. Knee. 2012;19(6):902-907.
- Koh Y-GC, Yun-Jin. Mesenchymal stem cell injections improve symptoms of knee osteoarthritis. Arthroscopy. 2013;29(4):748-755.
- Adriani E. MM, et al. Percutaneous Fat Transfer to Treat Knee Osteoarthritis Symptoms: Preliminary Results. Joints. 2017.
- Bianchi F, Maioli M, Leonardi E, et al. A New Nonenzymatic Method and Device to Obtain a Fat Tissue Derivative Highly Enriched in Pericyte-Like Elements by Mild Mechanical Forces From Human Lipoaspirates. Cell Transplantation. 2013;22(11):2063-2077
TAKE-HOME POINTS
- Severe knee osteoarthritis causes pain and limits functions in a substantial proportion of the aging population.
- Total knee arthroplasty is often recommended in this group of patients when conservative management has failed.
- Many patients in this group continue to seek a nonsurgical option for this process.
- Autologous, micro-fractured, minimally manipulated adipose tissue is easy to harvest, and injection into a knee joint resulted in significant improvement in pain and function for at least 12 months in this study population.
- This intervention may represent a nonsurgical treatment option to avoid knee joint replacement in this population.
Use of Musculoskeletal Ultrasound and Regenerative Therapies in Soccer
ABSTRACT
Improvements in ultrasound technology have increased the popularity and use of ultrasound as a diagnostic and therapeutic modality for many soccer-related musculoskeletal (MSK) injuries. As a dynamic imaging modality, ultrasound offers increased accuracy and efficacy with minimally invasive procedures, such as guided injections, percutaneous tenotomy, and regenerative therapies, in the clinical setting. Emerging evidence indicates that regenerative therapies, such as platelet-rich-plasma (PRP), mesenchymal stem cells, and amniotic products, are a promising treatment for many MSK injuries and are gaining popularity among professional athletes. PRP is a safe treatment for a number of MSK conditions and has been included in the standard of care. However, conflicting evidence on return-to-play timeframes and efficacy in certain MSK conditions have led to inconsistent recommendations on indications for use, dose, and timing of treatment. Mesenchymal stem cell therapy, while promising, lacks high-level evidence of efficacy despite its increasing use among athletes. Currently, no data are available regarding the outcome of the use of amniotic products for the treatment of injuries in athletes. Furthermore, preparation of many regenerative therapies eclipses the concept of minimal manipulation and is subject to US Food and Drug Administration phase I to III trials. High-level research on regenerative medicine therapies should be continuously conducted to establish their clinical efficacy and safety data.
ULTRASOUND
Ultrasound (US) was first introduced for musculoskeletal (MSK) evaluation in 1957.1 Since then, US has gained increasing attention due to its ease of utilization in the clinical setting, repeatability, noninvasiveness, capability for contralateral comparison, lack of radiation exposure, and capability to provide real-time dynamic tissue assessment.1 Compared with magnetic resonance imaging or computed tomography, US presents limitations, including decreased resolution of certain tissues, limited field of view, limited penetration beyond osseous structures, incomplete evaluation of a joint or structure, and operator experience. However, advancements in technology, image resolution, and portability have improved the visualization of multiple anatomic structures and the accuracy of minimally invasive ultrasound-guided procedures at the point of care. The use of US for guided hip injections possibly decreases the cost relative to fluoroscopic guidance.2 Other studies have reported that US, as a result of its safety profile, has replaced fluoroscopy for certain procedures, such as barbotage of calcific tendinosis.3 US has been used for diagnostic purposes and guidance for therapeutic interventions, such as needle aspiration, diagnostic or therapeutic injection, needle tenotomy, tissue release, hydro-dissection, and biopsy.3 Given its expanding application, US has been increasingly used in the clinical setting, athletic training room, and sidelines of athletic events.
DIAGNOSTIC ULTRASOUND
An epidemiologic review of the National Collegiate Athletic Association (NCAA) men’s and women’s soccer injuries from 1988 to 2003 reported over 24,000 combined injuries. Over 70% of these injuries are MSK in nature and often affect the lower extremities.4,5 Ekstrand and colleagues6 also conducted an epidemiological review of muscle injuries among professional soccer players from 2001 to 2009. They found that 92% of all muscle injuries involved the lower extremities. The portability of US allows it to serve as an ideal modality for diagnostic evaluation of acute MSK injuries. Klauser and colleagues7 developed consensus based on the recommendations of the European Society of Musculoskeletal Radiology (ESSR) for the clinical indication of diagnostic ultrasound. A grading system was developed to describe the clinical utility of diagnostic US evaluation of MSK structures:
• Grade 0: Ultrasound is not indicated;
• Grade 1: Ultrasound is indicated if other imaging techniques are not appropriate;
• Grade 2: Ultrasound indication is equivalent to other imaging modalities;
• Grade 3: Ultrasound is the first-choice technique.
Henderson and colleagues8 conducted a review of 95 studies (12 systemic reviews and 83 diagnostic studies) that investigated the accuracy of diagnostic US imaging on soft tissue MSK injuries of the upper and lower extremities. They reported the sensitivity and specificity of the method for detection of over 40 hip, knee, ankle, and foot injuries and assigned corresponding grades based on diagnostic accuracy by using the same system developed by Klauser and colleagues.7,8 Common MSK injuries of the lower extremity and their corresponding ESSR grades are listed in the Table. This study demonstrated that diagnostic US is highly accurate for a number of soft tissue MSK injuries of the lower extremity and consistently matches the recommendation grades issued by Klauser and colleagues.7 In the hands of a skilled operator, US has become an increasingly popular and cost-effective modality for diagnosis and monitoring of acute muscle injuries and chronic tendinopathies among soccer athletes.
Table. Clinical Indication Grades for Diagnostic Ultrasound Evaluation of Common Lower Extremity Injuries7,8
Hip | Knee | Foot/Ankle |
Synovitis/Effusion: 3 | Quadricep tendinosis/tear: 3 | Anterior talofibular ligament injury: 3 |
Snapping hip (extra-articular): 3 | Patella tendinopathy: 3 | Calcaneofibular ligament injury: 3 |
Gluteal tendon tear: 3 | Pes anserine bursitis: 3 | Peroneal tendon tear/subluxation: 3 |
Meralgia paresthetica: 3 | Periarticular bursitis & ganglion: 3 | Posterior tibial tendinopathy: 3 |
Lateral femoral cutaneous nerve injury: 3 | Osgood-Schlatter & Sinding-Larsen: 3 | Plantaris tendon tear: 3 |
Femoral nerve injury: 3 | Synovitis/Effusion: 3 | Plantar fasciitis: 3 |
Sports hernia: 3 | Baker’s Cyst: 2-3 | Calcific tendonitis: 3 |
Morel-Lavallée lesions: 3 | MCL injury: 2 | Retrocalcaneal bursitis: 3 |
Muscle injury (high grade): 3 | IT band friction: 2 | Joint effusion: 3 |
Trochanteric bursitis: 2 | Medial patella plica syndrome: 2 | Ganglion cyst: 3 |
Proximal hamstring injury: 2 | Meniscal cyst: 2 | Retinacula pathology: 3 |
Sciatica: 1-2 | Common perineal neuropathy: 2 | Achilles tendinopathy: 2 |
Muscle injury (low grade): 1 | Distal hamstring tendon injury: 1-2 | Haglund disease: 2 |
Psoas tendon pathology: 1 | Intra-articular ganglion: 1 | Deltoid ligament injury: 2 |
Osteoarthritis: 0 | Hoffa’s fat pad syndrome: 1 | Plantar plate tear: 2 |
Labral tear: 0 | Loose bodies: 1 | Syndesmotic injury: 2 |
| LCL injury: 0-1 | Morton’s neuroma: 2 |
| Popliteal injury: 0-1 | Deltoid ligament injury: 1 |
| Plica syndrome: 0 | Spring ligament injury: 1 |
| Full/partial ACL tear: 0 | Anterolateral ankle impingement: 0 |
| PCL tear: 0 | Posterior talofibular ligament injury: 0 |
| Medial/lateral meniscus tear: 0 |
|
| Osteochondritis dissecans: 0 |
|
Abbreviations: ACL, anterior cruciate ligament; IT, iliotibial; LCL, lateral collateral ligament; MCL, medial collateral ligament; PCL, posterior cruciate ligament.
ULTRASOUND-GUIDED THERAPEUTIC PROCEDURES
The use of US at the point of care for needle guidance has led to its widespread application for therapeutic procedures, including injections and multiple regenerative therapies. Intra-articular US-guided injection and aspiration are common therapeutic interventions performed in the clinical setting. In a position statement of the American Medical Society for Sports Medicine, US-guided injections were found to be more accurate (SORT A evidence), effective (SORT B evidence), and cost effective (SORT B evidence) than landmark-guided injections.3 A recent meta-analysis conducted by Daniels and colleagues1 demonstrated the improved accuracy and efficacy of US-guided injections at the knee, ankle, and foot. Injections may serve a diagnostic purpose when anesthetics, such as lidocaine, are used in isolation, a therapeutic purpose, or both.
Continue to: Percutaneous tenotomy involve...
REGENERATIVE THERAPIES FOR MUSCULOSKELETAL CONDITIONS
PERCUTANEOUS TENOTOMY
Percutaneous tenotomy involves the introduction of a needle into damaged soft tissues, most often tendons (“needling”), in an effort to stimulate a healing response and resect the diseased tendon tissue. Although tenotomy was initially performed as an open or arthroscopic surgical technique, advances in US technology have led to improved sensitivity and specificity identifying areas of tendinous injury (hypervascularity, hypoechogenicity, and calcification); as such, the combination of these techniques has been used in the outpatient setting. New commercial models incorporate ultrasound guidance with needles or micro-resection probes for real-time débridement of damaged tissues. Percutaneous tenotomy has been described in the management of tendinopathy involving the rotator cuff, medial and lateral epicondyles, patellar and Achilles tendons, and plantar fascia.
Housner and colleagues9 evaluated the safety and short-term efficacy of US-guided needle tenotomy in 13 patients with chronic tendinosis of the patella, Achilles tendon, gluteus medius, iliotibial tract, hamstring, and rectus femoris. They reported no procedural complications and a significant decrease in pain scores at 4 and 12 weeks of follow-up.
Koh and colleagues10 conducted a prospective case series to evaluate the safety and efficacy of office-based, US-guided percutaneous tenotomy (using a commercial model) on 20 patients with chronic lateral epicondylitis. The authors reported no wound complications and significant improvement in pain scores at each follow-up period up to 1 year. Subsequent post-procedural US evaluation of injured tissues revealed evidence of healing (decreased tendon thickness, vascularity, and hypoechogenicity) in over half the cohort after 6 months compared with the baseline.11
Lee and colleagues12 evaluated the efficacy of US-guided needle tenotomy combined with platelet-rich plasma (PRP) injection on chronic recalcitrant gluteus medius tendinopathy. In this case series, 21 patients underwent PRP and “needling” through the hypoechoic regions of the injured tendon under direct US guidance. After a period of rest, all patients completed the structured rehabilitation protocol. After an average follow-up of 10 months, all patients displayed significant improvements in all outcome questionnaires and did not report any significant adverse events. The authors concluded that tenotomy combined with PRP is a safe and effective method for treatment for recalcitrant gluteus medius tendinopathy.
These studies indicate that US-guided percutaneous tenotomy, alone or in combination with regenerative therapies, such as PRP, is a safe and effective treatment option for various tendinopathies. However, while tenotomy appears safe with promising results and no reported major adverse events, the level of evidence remains low.
ORTHOBIOLOGICS
Orthobiologics are substances composed of biological materials that can be used to aid or even hasten the healing of bones, muscles, tendons, and ligaments. Orthobiologics may contain growth factors, which initiate or stimulate the body’s reparative process; matrix proteins, which serve as scaffolding for healing tissues; or stem cells, specifically adult stem cells, which are multipotent and can differentiate into several cell lines. Adult stem cells are categorized as hematopoietic, neural, epithelial, skin, and mesenchymal types. Mesenchymal stem cells (MSCs) are of particular interest in sports medicine applications because they secrete growth factors and cytokines with trophic, chemotactic, and immunosuppressive properties.13 MSCs are also multipotent and can differentiate into bones, muscles, cartilages, and tendons.14-17MSCs are readily isolated from many sources, including bone marrow, adipose tissues, synovial tissues, peripheral blood, skeletal muscles, umbilical cord blood, and placenta.13,14Several types of regenerative therapies used in orthopedic and sports medicine practice include PRP, stem cell therapy, and amniotic membrane/fluid preparations. While each therapy possesses the potential for promising results, the paucity of research and discrepancies among studies regarding the description of stem cell lines used limit the available evidence on the true clinical benefits of these regenerative therapies.
[HEAD 3] PLATELET-RICH PLASMA
PRP is an autologous product that has been used to stimulate biological factors and promote healing since the 1970s. Through the activation of platelets, PRP improves localized recruitment, proliferation, and differentiation of cells involved in tissue repair. Platelets, which are non-nucleated bodies located in peripheral blood, contain and release 3 groups of bioactive factors that enhance the healing process. Growth factors and cytokines released from alpha-granules play a role in cell proliferation, chemotaxis, cell differentiation, and angiogenesis. Bioactive factors, such as serotonin and histamine, released from dense granules, increase capillary permeability and improve cell recruitment and migration. Adhesion molecules also assist in cell migration and creation of an extracellular matrix, which acts as a scaffold for wound healing.18 Platelets are activated by mechanical trauma or contact with multiple activators, including Von Willebrand factor, collagen, thrombin, or calcium chloride. When activated, platelets release growth factors and cytokines, which create a pro-inflammatory environment that mediates the tissue repair process. After the procedure, the pro-inflammatory environment may result in patient discomfort, which can be managed with ice and acetaminophen. Use of nonsteroidal anti-inflammatory drugs may theoretically inhibit the inflammatory cascade induced by PRP, and they are avoided before and after the procedure, although evidence regarding necessary time frames is lacking.
Continue to: PRP consists of...
PRP consists of the fractionated liquid component of autologous whole blood, which contains increased concentrations of platelets and cytokines. Different methods and commercial preparations are available for collecting and preparing PRP. Variations in the amount of blood drawn, use of anticoagulants, presence or absence of an activating agent, number of centrifuge spins, and overall platelet and white blood cell concentrations lead to difficulty in evaluating and interpreting the available evidence regarding PRP therapy.
In vitro and animal studies demonstrated promising and safe results regarding the healing effect of PRP on injured soft tissues, such as tendons, ligaments, and muscles. In this regard, a number of studies have evaluated the effect of PRP on human MSK injuries. However, in addition to the above-mentioned variabilities in PRP, many of such studies lack standardization and randomization techniques and include a small number of patients only, thereby limiting the overall comparison and clinical application.
A landmark study conducted by Mishra and Pavelko19 concluded that PRP significantly reduced pain in patients with chronic elbow tendinosis. Similar findings were reported in high-level overhead athletes with ulnar collateral ligament insufficiency, which did not improve with conservative management.20 Fitzpatrick and colleagues21 found improvements in pain with the use of single PRP injection as treatment for chronic gluteal tendinopathy. PRP can effectively improve pain and recovery in chronic ligament and tendon injuries, such as lateral epicondylitis, patellar tendinopathy, and plantar fasciitis, when patients are unresponsive to traditional conservative management. The application of PRP to treat acute MSK injuries has produced mixed results. Hamid and colleagues22 conducted a level II randomized controlled trial to evaluate the effect of PRP combined with a rehabilitation program for treatment of grade 2 hamstring injuries on return-to-play compared with rehabilitation alone. Fourteen athletes were randomized into the study and control groups. Hamid and colleagues22 reported improved return-to-play in the study group compared with that in the control (26.7 and 42.5 days, respectively). This study also reported lower pain scores in the PRP group over time, but the difference was not statistically significant. Zanon and colleagues23 conducted a prospective study to evaluate return-to-play in professional soccer players with acute hamstring strains treated with PRP and a rehabilitation program. This study determined that athletes treated with PRP were “match fit,” meaning they would be available for match selection in an average of 36.8 days. However, Zanon and colleagues23 did not include a control group for comparison. Other studies reported that PRP treatment of acutely injured muscles and medial collateral ligaments of soccer and basketball players decreased their return-to-play interval.18 Reviews by Hamilton and colleagues24 and Pas and colleagues25 concluded that PRP treatment of acutely injured tissues with good blood supply (eg, hamstring muscles) did not improve pain or return-to-play compared with standardized rehabilitation protocols. Similarly, in a double-blinded placebo controlled trial, Reurink and colleagues26 evaluated return-to-play in 80 athletes with acute hamstring injuries treated with a rehabilitation program and either PRP or placebo. Reurink and colleagues26 found no difference in return-to-play (42 days for both groups), but the difference was not statistically significant. PRP has also been used intraoperatively and shows promising results in total knee arthroplasty, anterior cruciate ligament reconstruction, acute Achilles tendon repair, rotator cuff repair, and cartilage repair. However, many of these intraoperative studies are limited to animal models.
In 2009, the World Anti-Doping Agency (WADA) prohibited the use of PRP because it contains autologous growth factors and IGF-1, which could produce an anabolic effect. Recent studies have failed to demonstrate any athletic advantages of using PRP. WADA has since removed PRP from its prohibited list. PRP is also not prohibited by the US Anti-Doping Agency (USADA) and many major professional sporting leagues in the United States. However, care must be taken in reviewing the components of PRP because many commercially available products differ in PRP formulation. Since 2010, many team physicians have increasingly used PRP to treat a wide range of athletic injuries. A recent anonymous survey conducted by a team of physicians on PRP use in elite athletes revealed minimal complications but significant variability among physicians with regard to timing, belief in evidence, and formulation and dosing of PRP treatments. Many physicians did implicate athlete desire as the main indication for treatment.27
As an autologous treatment, PRP injection has no serious adverse effects beyond mild discomfort as a result of the procedure and pro-inflammatory state in the days following injection. Recent concerns regarding the potential of PRP treatment for heterotopic ossification have been reported, but published information is limited to case reports. PRP can improve pain and function in patients with chronic MSK injury. PRP appears to be a safe and effective alternative to surgery for patients with injury to poorly perfused tissue, which has not improved with conservative measures, such as rest, physical therapy, and anti-inflammatory medications. Care should be taken when treating athletes with PRP to establish regulations on doping by individual governing bodies.
Continue to: Use of stem...
STEM CELL THERAPY
Use of stem cell therapy is based on the properties of the proliferation and differentiation of multipoint MSC lines. These stem cells can theoretically regenerate injured tissues and influence repair through immunomodulation; paracrine activity through the release of bioactive agents, such as cytokines, trophic, and chemotactic molecules; and cell differentiation into various cell lineages.15,16,13,17 Orthopedic surgeons have used microfracture to recruit MSCs during cartilage repair procedures for over 20 years. This procedure draws multipotent MSCs to the injured site to induce chondrogenic proliferation and fibrocartilage repair.28
Adult MSCs provide a readily accessible autologous source of stem cells for regenerative therapies. MSCs can be isolated from a variety of tissues, including bone marrow, adipose tissues, synovia, human umbilical cord blood, and peripheral blood. The majority of stem cell therapies in the United States for sports medicine purposes are conducted using bone marrow aspirate concentrate (BMAC) and adipose tissues. The US Food and Drug Administration (FDA) allows the use of minimally manipulated autologous stem cells to be injected into the same patient on the same day. However, some studies reported that culturing stem cells or introducing products, such as collagenase to stem cells, can increase the stem cell concentration prior to injection. These processes constitute more than “minimal manipulation” and therefore would require drug trials prior to use in the United States.
Although MSCs can be readily obtained from a variety of tissue sources, the makeup of the cell concentrate differs. Bone marrow and adipose tissues are readily available sources of homogenous MSCs. Harvesting stem cells from adipose tissues provides a less invasive route of collection than from BMAC. Harvested BMAC and adipose tissues consist of heterogeneous cell populations that are composed of precursor and accessory cells, such as pericytes, endothelial cells, smooth muscle cells, fibroblasts, and macrophages in addition to MSCs.
Animal studies reported promising results when evaluating soft tissue lesions in small and large animal models.14,15 Although clinical and human evidence remains limited, the potential of MSCs for regenerative repair has led to a recent increase in the number of related clinical studies. Multiple systematic reviews have concluded that MSC therapy is safe for the treatment of osteoarthritis, cartilage lesions, and tendinopathies. Limited evidence is available regarding the safety of intramuscular use, and a theoretical concern arises on the development of heterotopic bone formation as a result of treatment.13,16 The efficacy of MSC therapy is difficult to determine due to the lack of standardization in stem cell populations, adjuvants (eg, PRP, hyaluronic acid, and scaffolding preparations), and delivery methods used.13,17
Similar to PRP, the increased use of MSC therapy among high-profile athletes has led to the promotion of these therapies as safe and effective despite limited evidence.29 Although MSC therapy is a promising and safe treatment option for patients with soft tissue injuries, the paucity in data and human studies limit its clinical use. Moreover, data of MSC efficacy is complicated because of the disparity between clinical studies regarding MSC collection method (many of which eclipse the “minimal manipulation” standard), description of isolated cell concentrates, dosage, method of delivery, use of adjuvants, and lack of randomization. Further studies using [standardized] methods are needed before establishing a true consensus on the safety and efficacy of MSC therapy.
AMNIOTIC MEMBRANE
The placenta is a source of MSCs, a collagen-rich extracellular matrix, and bioactive growth and regulatory factors. The capacity of the placenta to modulate biological activities and tissue formation is thought to provide a means of tissue repair and healing. The placenta consists of amniotic fluid, amniotic membrane (AM), chorionic membrane, and umbilical cord blood and tissues. Although MSCs have been isolated from each component of placental tissues, amniotic and chorionic membranes and umbilical cord tissues yield the highest concentration.
The majority of regenerative studies involving the placenta used AM alone or in combination with other placental tissues. AM is a metabolically active tissue that consists of an epithelial layer, a basement membrane, and a mesenchymal tissue layer. In addition to being a source of stem cells, AM synthesizes many growth factors, vasoactive peptides, and cytokines, which are capable of tissue regeneration. AM was initially used as a biological scaffold for the treatment of skin burns and wounds. Other intrinsic properties of AM include the provision of a matrix for cellular migration and proliferation, enhanced wound healing with reduced scar formation, antibacterial activity, and lastly, non-immunogenic and immunosuppressive properties. These inherent characteristics have spurred studies on the potential use of AM in sports medicine as a minimally invasive means to treat osteoarthritis and injuries of tendons, ligaments, muscles, fascia, and cartilages.
Continue to: Animal studies reported...
Animal studies reported positive results with the use of AM to treat osteoarthritis, cartilage defects, and tendon and ligament injuries. Few studies involving human participants also revealed favorable results with regard to the use of AM for the treatment of plantar fasciitis and osteoarthritis; however, these studies are industry-sponsored and employed small sample sizes. The unique mixture of a collagen-rich extracellular matrix, bioactive growth factors, and pluripotent stem cells may allow AM to become an effective treatment for MSK injuries. Although initial animal and human studies show promising results, variabilities regarding models (animal and human), pathologies, placental tissues, and methods of preparation, preservation, and delivery used limit the ability for comparison, analysis, and drawing of definitive conclusions. Thus far, no studies have evaluated the use of currently available AM products for the treatment of injuries sustained by soccer players.
Despite the current popularity of AM as regenerative therapy in academic research and potential use in clinical treatment in sports medicine, physicians should remain aware of the limited evidence available. Other barriers to research and use AM as a regenerative therapy include regulatory classifications based on the concept of “minimal manipulation” in biologic therapies. Minimally manipulated placental allografts are less regulated, less costly to study, and more easily commercialized. These products are not required to undergo FDA phase I to III trials prior to premarket approval. In 2000, the FDA position on all AM products falls into 2 categories. The first position states that AM that contains allogenic stem cells mixed with another drug that is micronized and/or cryopreserved is more than “minimally manipulated” and therefore categorized as “biologic” and would be subject to phase I to III trials. Dehydrated and decellularized AM, however, may meet the concept of minimal manipulation and is only approved by the FDA as a wound covering. Thus, any application of AM for the treatment of sports medicine pathology is not currently FDA-approved, considered off-label, not covered by insurance, and subject to out-of-pocket pay.30,31
CONCLUSION
With improvements in technology and portability, US has become an effective imaging modality for point-of-care evaluation, diagnosis, and continuous monitoring of many MSK injuries. Additionally, as a dynamic imaging modality, US allows for increased accuracy and efficacy when combined with minimally invasive procedures, such as diagnostic and therapeutic guided injections and percutaneous tenotomy, in the clinical setting; thereby decreasing the overall healthcare costs. PRP is proven to be a safe treatment for several MSK conditions, such as lateral epicondylitis, patellar tendonitis, and plantar fasciitis. Although PRP has been included in the standard of care in some areas, this technique may be predominantly athlete driven. Conflicting evidence with regard to return-to-play timeframes following PRP treatment for muscular injuries and poor evidence in conditions, such as Achilles tendonitis, have led to inconsistent indications for use, dose, and timing of treatment. Although early evidence of MSC therapy is promising, high-level evidence for MSC therapy is insufficient, despite its increased use among athletes. Thus far, no data are available regarding the outcomes of the use of amniotic products for the treatment of injuries among athletes. Furthermore, the preparation of amniotic products has many regulatory concerns. The authors advocate for continuous high-level research on regenerative medicine therapies to establish clinical efficacy and safety data.
1. Daniels E, Cole D, Jacobs B, Phillips S. Existing Evidence on ultrasound-guided injections in sports medicine. Orthop J Sports Med. 2018;6(2):2325967118756576. doi:10.1177/2325967118756576.
2. Henne M, Centurion A, Rosas S, Youmans H, Osbahr D. Trends in utilization of image-guided hip joint injections. Unpublished. 2018.
3. Finnoff JT, Hall MM, Adams E, et al. American Medical Society for Sports Medicine position statement: Interventional musculoskeletal ultrasound in sports medicine. Clin J Sport Med. 2015;25:6-22. doi:10.1097/JSM.0000000000000175.
4. Agel J, Evans TA, Dick R, Putukian M, Marshal S. Descriptive epidemiology of collegiate men’s soccer injuries: National Collegiate Athletic Association Injury Surveillance System, 1988-1989 through 2002-2003. J Athl Train. 2007;42(2):270-277.
5. Dick R, Putukian M, Agel J, Evans T, Marshall S. Descriptive epidemiology of collegiate women’s soccer injuries: National Collegiate Athletic Association Injury Surveillance System, 1988-1989 through 2002-2003. J Athl Train. 2007;42(2):278-285.
6. Ekstrand J, Hagglund M, Walden M. Epidemiology of muscle injuries in professional football (soccer). Am J Sports Med. 2011;39(6):1226-1232. doi:10.1177/0363546510395879.
7. Klauser A, Tagliafico A, Allen G, et al. Clinical indications for musculoskeletal ultrasound: A Delphi-based consensus paper of the European society of musculoskeletal radiology. Eur Radiol. 2012;22(5):1140-1148. doi:10.1007/s00330-011-2356-3.
8. Henderson R, Walker B, Young K. The accuracy of diagnostic ultrasound imaging for musculoskeletal soft tissue pathology of the extremities: a comprehensive review of the literature. Chiropr Man Therap. 2015;23(1):31. doi:10.1186/s12998-015-0076-5.
9. Housner JA, Jacobson JA, Misko R. Sonographically guided percutaneous needle tenotomy for the treatment of chronic tendinosis. J Ultrasound Med. 2009;28(9):1187-1192. doi:10.7863/jum.2009.28.9.1187.
10. Koh J, Mohan PC, Howe TS, et al. Fasciotomy and surgical tenotomy for recalcitrant lateral elbow tendinopathy: early clinical experience with a novel device for minimally invasive percutaneous microresection. Am J Sports Med. 2013;41(3):636-644. doi:10.1177/0363546512470625.
11. Seng C, Mohan PC, Koh J, et al. Ultrasonic percutaneous tenotomy for recalcitrant lateral elbow tendinopathy: sustainability and sonographic progression at 3 years. Am J Sports Med. 2015;44(2):504-510. doi:10.1177/0363546515612758.
12. Lee J, Harrison J, Boachie-Adjei K, Vargas E, Moley P. Platelet-rich plasma injections with needle tenotomy for gluteus medius tendinopathy: A registry study with prospective follow-up. Orthop J Sports Med. 2016;4(11):2325967116671692. doi:10.1177/2325967116671692.
13. Osborne H, Anderson L, Burt P, Young M, Gerrard D. Australasian College of Sports Physicians-Position statement: the place of mesenchymal stem/stromal cell therapies in sport and exercise medicine. Br J Sports Med. 2016;50:1237-1244. doi:10.1136/bjsports-2015-095711.
14. Anderson J, Little D, Toth A, et al. Stem cell therapies for knee cartilage repair. The current status of preclinical and clinical studies. Am J Sports Med. 2013;42(9)2253-2261. doi:10.1177/0363546513508744.
15. Lee S, Kwon B, Lee Kyoungbun, Son Y, Chung S. Therapeutic mechanisms of human adipose-derived mesenchymal stem cells in a rat tendon injury model. Am J Sports Med. 2017;45(6):1429-1439. doi:10.1177/0363546517689874.
16. McIntyre J, Jones I, Han B, Vangsness C. Intra-articular mesenchymal stem cell therapy for the human joint. A systematic review. Am J Sports Med. 2017;0363546517735844. doi:10.1177/0363546517735844.
17. Pas HIMFL, Moen M, Haisma J, Winters M. No evidence for the use of stem cell therapy for tendon disorders: a systematic review. Br J Sports Med. 2017;51:996-1002. doi:10.1136/bjsports-2016-096794.
18. Foster T, Puskas B, Mandelbaum B, Gerhardt M, Rodeo S. Platelet-rich plasma: from basic science to clinical applications. Am J Sports Med. 2009;37(11):2259-2272. doi:10.1177/0363546509349921.
19. Mishra A, Pavelko T. Treatment of chronic elbow tendinosis with buffered platelet-rich plasma. Am J Sports Med. 2006;34(11):1774-1778. doi:10.1177/0363546506288850.
20. Dines J, Williams P, ElAttrache N, et al. Platelet-rich plasma can be used to successfully treat elbow ulnar collateral ligament insufficiency in high-level throwers. Am J Orthop. 2016;45(4):296-300.
21. Fitzpatrick J, Bulsara M, O’Donnel J, McCrory P, Zheng M. The effectiveness of platelet-rich plasma injections in gluteal tendinopathy. A randomized, double-blind controlled trial comparing a single platelet-rich plasma injection with a single corticosteroid injection. Am J Sports Med. 2018;46(4)933-939. doi:10.1177/0363546517745525.
22. Hamid M, Ali M, Yusof A, George J, Lee L. Platelet-rich plasma injections for the treatment of hamstring injuries: A randomized controlled trial. Am J Sports Med. 2014;42(10):2410-2418. doi:10.1177/0363546514541540.
23. Zanon G, Combi F, Combi A, Perticarini L, Sammarchi L, Benazzo F. Platelet-rich plasma in the treatment of acute hamstring injuries in professional football players. Joints. 2016;4(1):17-23. doi:10.11138/jts/2016.4.1.017.
24. Hamilton B, Tol JL, Almusa E, et al. Platelet-rich plasma does not enhance return to play in hamstring injuries: a randomized controlled trial. Br J Sports Med. 2015;49:943-950. doi:10.1136/bjsports-2015-094603.
25. Pas HIMFL, Reurink G, Tol JL, Wier A, Winters M, Moen M. Efficacy of rehabilitation (lengthening) exercises, platelet-rich plasma injections, and other conservative interventions in acute hamstring injuries: an updated systematic review and meta-analysis. Br J Sports Med. 2015;49:1197-1205. doi:10.1136/bjsports-2015-094879.
26. Reurink G, Goudswaard G, Moen M, et al. Platelet-rich plasma injections in acute muscle injury. N Engl J Med. 2014;370:2546-2547. doi:10.1056/NEJMc1402340.
27. Kantrowitz D, Padaki A, Ahmad C, Lynch T. Defining platelet-rich plasma usage by team physicians in elite athletes. Orthop J Sports Med. 2018;6(4):2325967118767077. doi:10.1177/2325967118767077.
28. Mithoefer K, Peterson L, Zenobi-Wong M, Mandelbaum B. Cartilage issues in football-today’s problems and tomorrow’s solutions. Br J Sports Med. 2015;49(9):590-596. doi:1136/bjsports-2015-094772.
29. Matthews K, Cuchiara M. Regional regulatory insights: U.S. National Football League Athletes seeking unproven stem cell treatments. Stem Cells Dev. 2014;23(S1):60-64. doi:10.1089/scd.2014.0358.
30. McIntyre J, Jones I, Danilkovich A, Vangsness T. The placenta: applications in orthopaedic sports medicine. Am J Sports Med. 2018;46(1):234-247. doi:10.1177/0363546517697682.
31. Riboh J, Saltzman B, Yankee A, Cole BJ. Human amniotic membrane-derived products in sports medicine: Basic science, early results, and potential clinical applications. Am J Sports Med. 2015;44(9)2425-2434. doi:10.1177/0363546515612750.
ABSTRACT
Improvements in ultrasound technology have increased the popularity and use of ultrasound as a diagnostic and therapeutic modality for many soccer-related musculoskeletal (MSK) injuries. As a dynamic imaging modality, ultrasound offers increased accuracy and efficacy with minimally invasive procedures, such as guided injections, percutaneous tenotomy, and regenerative therapies, in the clinical setting. Emerging evidence indicates that regenerative therapies, such as platelet-rich-plasma (PRP), mesenchymal stem cells, and amniotic products, are a promising treatment for many MSK injuries and are gaining popularity among professional athletes. PRP is a safe treatment for a number of MSK conditions and has been included in the standard of care. However, conflicting evidence on return-to-play timeframes and efficacy in certain MSK conditions have led to inconsistent recommendations on indications for use, dose, and timing of treatment. Mesenchymal stem cell therapy, while promising, lacks high-level evidence of efficacy despite its increasing use among athletes. Currently, no data are available regarding the outcome of the use of amniotic products for the treatment of injuries in athletes. Furthermore, preparation of many regenerative therapies eclipses the concept of minimal manipulation and is subject to US Food and Drug Administration phase I to III trials. High-level research on regenerative medicine therapies should be continuously conducted to establish their clinical efficacy and safety data.
ULTRASOUND
Ultrasound (US) was first introduced for musculoskeletal (MSK) evaluation in 1957.1 Since then, US has gained increasing attention due to its ease of utilization in the clinical setting, repeatability, noninvasiveness, capability for contralateral comparison, lack of radiation exposure, and capability to provide real-time dynamic tissue assessment.1 Compared with magnetic resonance imaging or computed tomography, US presents limitations, including decreased resolution of certain tissues, limited field of view, limited penetration beyond osseous structures, incomplete evaluation of a joint or structure, and operator experience. However, advancements in technology, image resolution, and portability have improved the visualization of multiple anatomic structures and the accuracy of minimally invasive ultrasound-guided procedures at the point of care. The use of US for guided hip injections possibly decreases the cost relative to fluoroscopic guidance.2 Other studies have reported that US, as a result of its safety profile, has replaced fluoroscopy for certain procedures, such as barbotage of calcific tendinosis.3 US has been used for diagnostic purposes and guidance for therapeutic interventions, such as needle aspiration, diagnostic or therapeutic injection, needle tenotomy, tissue release, hydro-dissection, and biopsy.3 Given its expanding application, US has been increasingly used in the clinical setting, athletic training room, and sidelines of athletic events.
DIAGNOSTIC ULTRASOUND
An epidemiologic review of the National Collegiate Athletic Association (NCAA) men’s and women’s soccer injuries from 1988 to 2003 reported over 24,000 combined injuries. Over 70% of these injuries are MSK in nature and often affect the lower extremities.4,5 Ekstrand and colleagues6 also conducted an epidemiological review of muscle injuries among professional soccer players from 2001 to 2009. They found that 92% of all muscle injuries involved the lower extremities. The portability of US allows it to serve as an ideal modality for diagnostic evaluation of acute MSK injuries. Klauser and colleagues7 developed consensus based on the recommendations of the European Society of Musculoskeletal Radiology (ESSR) for the clinical indication of diagnostic ultrasound. A grading system was developed to describe the clinical utility of diagnostic US evaluation of MSK structures:
• Grade 0: Ultrasound is not indicated;
• Grade 1: Ultrasound is indicated if other imaging techniques are not appropriate;
• Grade 2: Ultrasound indication is equivalent to other imaging modalities;
• Grade 3: Ultrasound is the first-choice technique.
Henderson and colleagues8 conducted a review of 95 studies (12 systemic reviews and 83 diagnostic studies) that investigated the accuracy of diagnostic US imaging on soft tissue MSK injuries of the upper and lower extremities. They reported the sensitivity and specificity of the method for detection of over 40 hip, knee, ankle, and foot injuries and assigned corresponding grades based on diagnostic accuracy by using the same system developed by Klauser and colleagues.7,8 Common MSK injuries of the lower extremity and their corresponding ESSR grades are listed in the Table. This study demonstrated that diagnostic US is highly accurate for a number of soft tissue MSK injuries of the lower extremity and consistently matches the recommendation grades issued by Klauser and colleagues.7 In the hands of a skilled operator, US has become an increasingly popular and cost-effective modality for diagnosis and monitoring of acute muscle injuries and chronic tendinopathies among soccer athletes.
Table. Clinical Indication Grades for Diagnostic Ultrasound Evaluation of Common Lower Extremity Injuries7,8
Hip | Knee | Foot/Ankle |
Synovitis/Effusion: 3 | Quadricep tendinosis/tear: 3 | Anterior talofibular ligament injury: 3 |
Snapping hip (extra-articular): 3 | Patella tendinopathy: 3 | Calcaneofibular ligament injury: 3 |
Gluteal tendon tear: 3 | Pes anserine bursitis: 3 | Peroneal tendon tear/subluxation: 3 |
Meralgia paresthetica: 3 | Periarticular bursitis & ganglion: 3 | Posterior tibial tendinopathy: 3 |
Lateral femoral cutaneous nerve injury: 3 | Osgood-Schlatter & Sinding-Larsen: 3 | Plantaris tendon tear: 3 |
Femoral nerve injury: 3 | Synovitis/Effusion: 3 | Plantar fasciitis: 3 |
Sports hernia: 3 | Baker’s Cyst: 2-3 | Calcific tendonitis: 3 |
Morel-Lavallée lesions: 3 | MCL injury: 2 | Retrocalcaneal bursitis: 3 |
Muscle injury (high grade): 3 | IT band friction: 2 | Joint effusion: 3 |
Trochanteric bursitis: 2 | Medial patella plica syndrome: 2 | Ganglion cyst: 3 |
Proximal hamstring injury: 2 | Meniscal cyst: 2 | Retinacula pathology: 3 |
Sciatica: 1-2 | Common perineal neuropathy: 2 | Achilles tendinopathy: 2 |
Muscle injury (low grade): 1 | Distal hamstring tendon injury: 1-2 | Haglund disease: 2 |
Psoas tendon pathology: 1 | Intra-articular ganglion: 1 | Deltoid ligament injury: 2 |
Osteoarthritis: 0 | Hoffa’s fat pad syndrome: 1 | Plantar plate tear: 2 |
Labral tear: 0 | Loose bodies: 1 | Syndesmotic injury: 2 |
| LCL injury: 0-1 | Morton’s neuroma: 2 |
| Popliteal injury: 0-1 | Deltoid ligament injury: 1 |
| Plica syndrome: 0 | Spring ligament injury: 1 |
| Full/partial ACL tear: 0 | Anterolateral ankle impingement: 0 |
| PCL tear: 0 | Posterior talofibular ligament injury: 0 |
| Medial/lateral meniscus tear: 0 |
|
| Osteochondritis dissecans: 0 |
|
Abbreviations: ACL, anterior cruciate ligament; IT, iliotibial; LCL, lateral collateral ligament; MCL, medial collateral ligament; PCL, posterior cruciate ligament.
ULTRASOUND-GUIDED THERAPEUTIC PROCEDURES
The use of US at the point of care for needle guidance has led to its widespread application for therapeutic procedures, including injections and multiple regenerative therapies. Intra-articular US-guided injection and aspiration are common therapeutic interventions performed in the clinical setting. In a position statement of the American Medical Society for Sports Medicine, US-guided injections were found to be more accurate (SORT A evidence), effective (SORT B evidence), and cost effective (SORT B evidence) than landmark-guided injections.3 A recent meta-analysis conducted by Daniels and colleagues1 demonstrated the improved accuracy and efficacy of US-guided injections at the knee, ankle, and foot. Injections may serve a diagnostic purpose when anesthetics, such as lidocaine, are used in isolation, a therapeutic purpose, or both.
Continue to: Percutaneous tenotomy involve...
REGENERATIVE THERAPIES FOR MUSCULOSKELETAL CONDITIONS
PERCUTANEOUS TENOTOMY
Percutaneous tenotomy involves the introduction of a needle into damaged soft tissues, most often tendons (“needling”), in an effort to stimulate a healing response and resect the diseased tendon tissue. Although tenotomy was initially performed as an open or arthroscopic surgical technique, advances in US technology have led to improved sensitivity and specificity identifying areas of tendinous injury (hypervascularity, hypoechogenicity, and calcification); as such, the combination of these techniques has been used in the outpatient setting. New commercial models incorporate ultrasound guidance with needles or micro-resection probes for real-time débridement of damaged tissues. Percutaneous tenotomy has been described in the management of tendinopathy involving the rotator cuff, medial and lateral epicondyles, patellar and Achilles tendons, and plantar fascia.
Housner and colleagues9 evaluated the safety and short-term efficacy of US-guided needle tenotomy in 13 patients with chronic tendinosis of the patella, Achilles tendon, gluteus medius, iliotibial tract, hamstring, and rectus femoris. They reported no procedural complications and a significant decrease in pain scores at 4 and 12 weeks of follow-up.
Koh and colleagues10 conducted a prospective case series to evaluate the safety and efficacy of office-based, US-guided percutaneous tenotomy (using a commercial model) on 20 patients with chronic lateral epicondylitis. The authors reported no wound complications and significant improvement in pain scores at each follow-up period up to 1 year. Subsequent post-procedural US evaluation of injured tissues revealed evidence of healing (decreased tendon thickness, vascularity, and hypoechogenicity) in over half the cohort after 6 months compared with the baseline.11
Lee and colleagues12 evaluated the efficacy of US-guided needle tenotomy combined with platelet-rich plasma (PRP) injection on chronic recalcitrant gluteus medius tendinopathy. In this case series, 21 patients underwent PRP and “needling” through the hypoechoic regions of the injured tendon under direct US guidance. After a period of rest, all patients completed the structured rehabilitation protocol. After an average follow-up of 10 months, all patients displayed significant improvements in all outcome questionnaires and did not report any significant adverse events. The authors concluded that tenotomy combined with PRP is a safe and effective method for treatment for recalcitrant gluteus medius tendinopathy.
These studies indicate that US-guided percutaneous tenotomy, alone or in combination with regenerative therapies, such as PRP, is a safe and effective treatment option for various tendinopathies. However, while tenotomy appears safe with promising results and no reported major adverse events, the level of evidence remains low.
ORTHOBIOLOGICS
Orthobiologics are substances composed of biological materials that can be used to aid or even hasten the healing of bones, muscles, tendons, and ligaments. Orthobiologics may contain growth factors, which initiate or stimulate the body’s reparative process; matrix proteins, which serve as scaffolding for healing tissues; or stem cells, specifically adult stem cells, which are multipotent and can differentiate into several cell lines. Adult stem cells are categorized as hematopoietic, neural, epithelial, skin, and mesenchymal types. Mesenchymal stem cells (MSCs) are of particular interest in sports medicine applications because they secrete growth factors and cytokines with trophic, chemotactic, and immunosuppressive properties.13 MSCs are also multipotent and can differentiate into bones, muscles, cartilages, and tendons.14-17MSCs are readily isolated from many sources, including bone marrow, adipose tissues, synovial tissues, peripheral blood, skeletal muscles, umbilical cord blood, and placenta.13,14Several types of regenerative therapies used in orthopedic and sports medicine practice include PRP, stem cell therapy, and amniotic membrane/fluid preparations. While each therapy possesses the potential for promising results, the paucity of research and discrepancies among studies regarding the description of stem cell lines used limit the available evidence on the true clinical benefits of these regenerative therapies.
[HEAD 3] PLATELET-RICH PLASMA
PRP is an autologous product that has been used to stimulate biological factors and promote healing since the 1970s. Through the activation of platelets, PRP improves localized recruitment, proliferation, and differentiation of cells involved in tissue repair. Platelets, which are non-nucleated bodies located in peripheral blood, contain and release 3 groups of bioactive factors that enhance the healing process. Growth factors and cytokines released from alpha-granules play a role in cell proliferation, chemotaxis, cell differentiation, and angiogenesis. Bioactive factors, such as serotonin and histamine, released from dense granules, increase capillary permeability and improve cell recruitment and migration. Adhesion molecules also assist in cell migration and creation of an extracellular matrix, which acts as a scaffold for wound healing.18 Platelets are activated by mechanical trauma or contact with multiple activators, including Von Willebrand factor, collagen, thrombin, or calcium chloride. When activated, platelets release growth factors and cytokines, which create a pro-inflammatory environment that mediates the tissue repair process. After the procedure, the pro-inflammatory environment may result in patient discomfort, which can be managed with ice and acetaminophen. Use of nonsteroidal anti-inflammatory drugs may theoretically inhibit the inflammatory cascade induced by PRP, and they are avoided before and after the procedure, although evidence regarding necessary time frames is lacking.
Continue to: PRP consists of...
PRP consists of the fractionated liquid component of autologous whole blood, which contains increased concentrations of platelets and cytokines. Different methods and commercial preparations are available for collecting and preparing PRP. Variations in the amount of blood drawn, use of anticoagulants, presence or absence of an activating agent, number of centrifuge spins, and overall platelet and white blood cell concentrations lead to difficulty in evaluating and interpreting the available evidence regarding PRP therapy.
In vitro and animal studies demonstrated promising and safe results regarding the healing effect of PRP on injured soft tissues, such as tendons, ligaments, and muscles. In this regard, a number of studies have evaluated the effect of PRP on human MSK injuries. However, in addition to the above-mentioned variabilities in PRP, many of such studies lack standardization and randomization techniques and include a small number of patients only, thereby limiting the overall comparison and clinical application.
A landmark study conducted by Mishra and Pavelko19 concluded that PRP significantly reduced pain in patients with chronic elbow tendinosis. Similar findings were reported in high-level overhead athletes with ulnar collateral ligament insufficiency, which did not improve with conservative management.20 Fitzpatrick and colleagues21 found improvements in pain with the use of single PRP injection as treatment for chronic gluteal tendinopathy. PRP can effectively improve pain and recovery in chronic ligament and tendon injuries, such as lateral epicondylitis, patellar tendinopathy, and plantar fasciitis, when patients are unresponsive to traditional conservative management. The application of PRP to treat acute MSK injuries has produced mixed results. Hamid and colleagues22 conducted a level II randomized controlled trial to evaluate the effect of PRP combined with a rehabilitation program for treatment of grade 2 hamstring injuries on return-to-play compared with rehabilitation alone. Fourteen athletes were randomized into the study and control groups. Hamid and colleagues22 reported improved return-to-play in the study group compared with that in the control (26.7 and 42.5 days, respectively). This study also reported lower pain scores in the PRP group over time, but the difference was not statistically significant. Zanon and colleagues23 conducted a prospective study to evaluate return-to-play in professional soccer players with acute hamstring strains treated with PRP and a rehabilitation program. This study determined that athletes treated with PRP were “match fit,” meaning they would be available for match selection in an average of 36.8 days. However, Zanon and colleagues23 did not include a control group for comparison. Other studies reported that PRP treatment of acutely injured muscles and medial collateral ligaments of soccer and basketball players decreased their return-to-play interval.18 Reviews by Hamilton and colleagues24 and Pas and colleagues25 concluded that PRP treatment of acutely injured tissues with good blood supply (eg, hamstring muscles) did not improve pain or return-to-play compared with standardized rehabilitation protocols. Similarly, in a double-blinded placebo controlled trial, Reurink and colleagues26 evaluated return-to-play in 80 athletes with acute hamstring injuries treated with a rehabilitation program and either PRP or placebo. Reurink and colleagues26 found no difference in return-to-play (42 days for both groups), but the difference was not statistically significant. PRP has also been used intraoperatively and shows promising results in total knee arthroplasty, anterior cruciate ligament reconstruction, acute Achilles tendon repair, rotator cuff repair, and cartilage repair. However, many of these intraoperative studies are limited to animal models.
In 2009, the World Anti-Doping Agency (WADA) prohibited the use of PRP because it contains autologous growth factors and IGF-1, which could produce an anabolic effect. Recent studies have failed to demonstrate any athletic advantages of using PRP. WADA has since removed PRP from its prohibited list. PRP is also not prohibited by the US Anti-Doping Agency (USADA) and many major professional sporting leagues in the United States. However, care must be taken in reviewing the components of PRP because many commercially available products differ in PRP formulation. Since 2010, many team physicians have increasingly used PRP to treat a wide range of athletic injuries. A recent anonymous survey conducted by a team of physicians on PRP use in elite athletes revealed minimal complications but significant variability among physicians with regard to timing, belief in evidence, and formulation and dosing of PRP treatments. Many physicians did implicate athlete desire as the main indication for treatment.27
As an autologous treatment, PRP injection has no serious adverse effects beyond mild discomfort as a result of the procedure and pro-inflammatory state in the days following injection. Recent concerns regarding the potential of PRP treatment for heterotopic ossification have been reported, but published information is limited to case reports. PRP can improve pain and function in patients with chronic MSK injury. PRP appears to be a safe and effective alternative to surgery for patients with injury to poorly perfused tissue, which has not improved with conservative measures, such as rest, physical therapy, and anti-inflammatory medications. Care should be taken when treating athletes with PRP to establish regulations on doping by individual governing bodies.
Continue to: Use of stem...
STEM CELL THERAPY
Use of stem cell therapy is based on the properties of the proliferation and differentiation of multipoint MSC lines. These stem cells can theoretically regenerate injured tissues and influence repair through immunomodulation; paracrine activity through the release of bioactive agents, such as cytokines, trophic, and chemotactic molecules; and cell differentiation into various cell lineages.15,16,13,17 Orthopedic surgeons have used microfracture to recruit MSCs during cartilage repair procedures for over 20 years. This procedure draws multipotent MSCs to the injured site to induce chondrogenic proliferation and fibrocartilage repair.28
Adult MSCs provide a readily accessible autologous source of stem cells for regenerative therapies. MSCs can be isolated from a variety of tissues, including bone marrow, adipose tissues, synovia, human umbilical cord blood, and peripheral blood. The majority of stem cell therapies in the United States for sports medicine purposes are conducted using bone marrow aspirate concentrate (BMAC) and adipose tissues. The US Food and Drug Administration (FDA) allows the use of minimally manipulated autologous stem cells to be injected into the same patient on the same day. However, some studies reported that culturing stem cells or introducing products, such as collagenase to stem cells, can increase the stem cell concentration prior to injection. These processes constitute more than “minimal manipulation” and therefore would require drug trials prior to use in the United States.
Although MSCs can be readily obtained from a variety of tissue sources, the makeup of the cell concentrate differs. Bone marrow and adipose tissues are readily available sources of homogenous MSCs. Harvesting stem cells from adipose tissues provides a less invasive route of collection than from BMAC. Harvested BMAC and adipose tissues consist of heterogeneous cell populations that are composed of precursor and accessory cells, such as pericytes, endothelial cells, smooth muscle cells, fibroblasts, and macrophages in addition to MSCs.
Animal studies reported promising results when evaluating soft tissue lesions in small and large animal models.14,15 Although clinical and human evidence remains limited, the potential of MSCs for regenerative repair has led to a recent increase in the number of related clinical studies. Multiple systematic reviews have concluded that MSC therapy is safe for the treatment of osteoarthritis, cartilage lesions, and tendinopathies. Limited evidence is available regarding the safety of intramuscular use, and a theoretical concern arises on the development of heterotopic bone formation as a result of treatment.13,16 The efficacy of MSC therapy is difficult to determine due to the lack of standardization in stem cell populations, adjuvants (eg, PRP, hyaluronic acid, and scaffolding preparations), and delivery methods used.13,17
Similar to PRP, the increased use of MSC therapy among high-profile athletes has led to the promotion of these therapies as safe and effective despite limited evidence.29 Although MSC therapy is a promising and safe treatment option for patients with soft tissue injuries, the paucity in data and human studies limit its clinical use. Moreover, data of MSC efficacy is complicated because of the disparity between clinical studies regarding MSC collection method (many of which eclipse the “minimal manipulation” standard), description of isolated cell concentrates, dosage, method of delivery, use of adjuvants, and lack of randomization. Further studies using [standardized] methods are needed before establishing a true consensus on the safety and efficacy of MSC therapy.
AMNIOTIC MEMBRANE
The placenta is a source of MSCs, a collagen-rich extracellular matrix, and bioactive growth and regulatory factors. The capacity of the placenta to modulate biological activities and tissue formation is thought to provide a means of tissue repair and healing. The placenta consists of amniotic fluid, amniotic membrane (AM), chorionic membrane, and umbilical cord blood and tissues. Although MSCs have been isolated from each component of placental tissues, amniotic and chorionic membranes and umbilical cord tissues yield the highest concentration.
The majority of regenerative studies involving the placenta used AM alone or in combination with other placental tissues. AM is a metabolically active tissue that consists of an epithelial layer, a basement membrane, and a mesenchymal tissue layer. In addition to being a source of stem cells, AM synthesizes many growth factors, vasoactive peptides, and cytokines, which are capable of tissue regeneration. AM was initially used as a biological scaffold for the treatment of skin burns and wounds. Other intrinsic properties of AM include the provision of a matrix for cellular migration and proliferation, enhanced wound healing with reduced scar formation, antibacterial activity, and lastly, non-immunogenic and immunosuppressive properties. These inherent characteristics have spurred studies on the potential use of AM in sports medicine as a minimally invasive means to treat osteoarthritis and injuries of tendons, ligaments, muscles, fascia, and cartilages.
Continue to: Animal studies reported...
Animal studies reported positive results with the use of AM to treat osteoarthritis, cartilage defects, and tendon and ligament injuries. Few studies involving human participants also revealed favorable results with regard to the use of AM for the treatment of plantar fasciitis and osteoarthritis; however, these studies are industry-sponsored and employed small sample sizes. The unique mixture of a collagen-rich extracellular matrix, bioactive growth factors, and pluripotent stem cells may allow AM to become an effective treatment for MSK injuries. Although initial animal and human studies show promising results, variabilities regarding models (animal and human), pathologies, placental tissues, and methods of preparation, preservation, and delivery used limit the ability for comparison, analysis, and drawing of definitive conclusions. Thus far, no studies have evaluated the use of currently available AM products for the treatment of injuries sustained by soccer players.
Despite the current popularity of AM as regenerative therapy in academic research and potential use in clinical treatment in sports medicine, physicians should remain aware of the limited evidence available. Other barriers to research and use AM as a regenerative therapy include regulatory classifications based on the concept of “minimal manipulation” in biologic therapies. Minimally manipulated placental allografts are less regulated, less costly to study, and more easily commercialized. These products are not required to undergo FDA phase I to III trials prior to premarket approval. In 2000, the FDA position on all AM products falls into 2 categories. The first position states that AM that contains allogenic stem cells mixed with another drug that is micronized and/or cryopreserved is more than “minimally manipulated” and therefore categorized as “biologic” and would be subject to phase I to III trials. Dehydrated and decellularized AM, however, may meet the concept of minimal manipulation and is only approved by the FDA as a wound covering. Thus, any application of AM for the treatment of sports medicine pathology is not currently FDA-approved, considered off-label, not covered by insurance, and subject to out-of-pocket pay.30,31
CONCLUSION
With improvements in technology and portability, US has become an effective imaging modality for point-of-care evaluation, diagnosis, and continuous monitoring of many MSK injuries. Additionally, as a dynamic imaging modality, US allows for increased accuracy and efficacy when combined with minimally invasive procedures, such as diagnostic and therapeutic guided injections and percutaneous tenotomy, in the clinical setting; thereby decreasing the overall healthcare costs. PRP is proven to be a safe treatment for several MSK conditions, such as lateral epicondylitis, patellar tendonitis, and plantar fasciitis. Although PRP has been included in the standard of care in some areas, this technique may be predominantly athlete driven. Conflicting evidence with regard to return-to-play timeframes following PRP treatment for muscular injuries and poor evidence in conditions, such as Achilles tendonitis, have led to inconsistent indications for use, dose, and timing of treatment. Although early evidence of MSC therapy is promising, high-level evidence for MSC therapy is insufficient, despite its increased use among athletes. Thus far, no data are available regarding the outcomes of the use of amniotic products for the treatment of injuries among athletes. Furthermore, the preparation of amniotic products has many regulatory concerns. The authors advocate for continuous high-level research on regenerative medicine therapies to establish clinical efficacy and safety data.
ABSTRACT
Improvements in ultrasound technology have increased the popularity and use of ultrasound as a diagnostic and therapeutic modality for many soccer-related musculoskeletal (MSK) injuries. As a dynamic imaging modality, ultrasound offers increased accuracy and efficacy with minimally invasive procedures, such as guided injections, percutaneous tenotomy, and regenerative therapies, in the clinical setting. Emerging evidence indicates that regenerative therapies, such as platelet-rich-plasma (PRP), mesenchymal stem cells, and amniotic products, are a promising treatment for many MSK injuries and are gaining popularity among professional athletes. PRP is a safe treatment for a number of MSK conditions and has been included in the standard of care. However, conflicting evidence on return-to-play timeframes and efficacy in certain MSK conditions have led to inconsistent recommendations on indications for use, dose, and timing of treatment. Mesenchymal stem cell therapy, while promising, lacks high-level evidence of efficacy despite its increasing use among athletes. Currently, no data are available regarding the outcome of the use of amniotic products for the treatment of injuries in athletes. Furthermore, preparation of many regenerative therapies eclipses the concept of minimal manipulation and is subject to US Food and Drug Administration phase I to III trials. High-level research on regenerative medicine therapies should be continuously conducted to establish their clinical efficacy and safety data.
ULTRASOUND
Ultrasound (US) was first introduced for musculoskeletal (MSK) evaluation in 1957.1 Since then, US has gained increasing attention due to its ease of utilization in the clinical setting, repeatability, noninvasiveness, capability for contralateral comparison, lack of radiation exposure, and capability to provide real-time dynamic tissue assessment.1 Compared with magnetic resonance imaging or computed tomography, US presents limitations, including decreased resolution of certain tissues, limited field of view, limited penetration beyond osseous structures, incomplete evaluation of a joint or structure, and operator experience. However, advancements in technology, image resolution, and portability have improved the visualization of multiple anatomic structures and the accuracy of minimally invasive ultrasound-guided procedures at the point of care. The use of US for guided hip injections possibly decreases the cost relative to fluoroscopic guidance.2 Other studies have reported that US, as a result of its safety profile, has replaced fluoroscopy for certain procedures, such as barbotage of calcific tendinosis.3 US has been used for diagnostic purposes and guidance for therapeutic interventions, such as needle aspiration, diagnostic or therapeutic injection, needle tenotomy, tissue release, hydro-dissection, and biopsy.3 Given its expanding application, US has been increasingly used in the clinical setting, athletic training room, and sidelines of athletic events.
DIAGNOSTIC ULTRASOUND
An epidemiologic review of the National Collegiate Athletic Association (NCAA) men’s and women’s soccer injuries from 1988 to 2003 reported over 24,000 combined injuries. Over 70% of these injuries are MSK in nature and often affect the lower extremities.4,5 Ekstrand and colleagues6 also conducted an epidemiological review of muscle injuries among professional soccer players from 2001 to 2009. They found that 92% of all muscle injuries involved the lower extremities. The portability of US allows it to serve as an ideal modality for diagnostic evaluation of acute MSK injuries. Klauser and colleagues7 developed consensus based on the recommendations of the European Society of Musculoskeletal Radiology (ESSR) for the clinical indication of diagnostic ultrasound. A grading system was developed to describe the clinical utility of diagnostic US evaluation of MSK structures:
• Grade 0: Ultrasound is not indicated;
• Grade 1: Ultrasound is indicated if other imaging techniques are not appropriate;
• Grade 2: Ultrasound indication is equivalent to other imaging modalities;
• Grade 3: Ultrasound is the first-choice technique.
Henderson and colleagues8 conducted a review of 95 studies (12 systemic reviews and 83 diagnostic studies) that investigated the accuracy of diagnostic US imaging on soft tissue MSK injuries of the upper and lower extremities. They reported the sensitivity and specificity of the method for detection of over 40 hip, knee, ankle, and foot injuries and assigned corresponding grades based on diagnostic accuracy by using the same system developed by Klauser and colleagues.7,8 Common MSK injuries of the lower extremity and their corresponding ESSR grades are listed in the Table. This study demonstrated that diagnostic US is highly accurate for a number of soft tissue MSK injuries of the lower extremity and consistently matches the recommendation grades issued by Klauser and colleagues.7 In the hands of a skilled operator, US has become an increasingly popular and cost-effective modality for diagnosis and monitoring of acute muscle injuries and chronic tendinopathies among soccer athletes.
Table. Clinical Indication Grades for Diagnostic Ultrasound Evaluation of Common Lower Extremity Injuries7,8
Hip | Knee | Foot/Ankle |
Synovitis/Effusion: 3 | Quadricep tendinosis/tear: 3 | Anterior talofibular ligament injury: 3 |
Snapping hip (extra-articular): 3 | Patella tendinopathy: 3 | Calcaneofibular ligament injury: 3 |
Gluteal tendon tear: 3 | Pes anserine bursitis: 3 | Peroneal tendon tear/subluxation: 3 |
Meralgia paresthetica: 3 | Periarticular bursitis & ganglion: 3 | Posterior tibial tendinopathy: 3 |
Lateral femoral cutaneous nerve injury: 3 | Osgood-Schlatter & Sinding-Larsen: 3 | Plantaris tendon tear: 3 |
Femoral nerve injury: 3 | Synovitis/Effusion: 3 | Plantar fasciitis: 3 |
Sports hernia: 3 | Baker’s Cyst: 2-3 | Calcific tendonitis: 3 |
Morel-Lavallée lesions: 3 | MCL injury: 2 | Retrocalcaneal bursitis: 3 |
Muscle injury (high grade): 3 | IT band friction: 2 | Joint effusion: 3 |
Trochanteric bursitis: 2 | Medial patella plica syndrome: 2 | Ganglion cyst: 3 |
Proximal hamstring injury: 2 | Meniscal cyst: 2 | Retinacula pathology: 3 |
Sciatica: 1-2 | Common perineal neuropathy: 2 | Achilles tendinopathy: 2 |
Muscle injury (low grade): 1 | Distal hamstring tendon injury: 1-2 | Haglund disease: 2 |
Psoas tendon pathology: 1 | Intra-articular ganglion: 1 | Deltoid ligament injury: 2 |
Osteoarthritis: 0 | Hoffa’s fat pad syndrome: 1 | Plantar plate tear: 2 |
Labral tear: 0 | Loose bodies: 1 | Syndesmotic injury: 2 |
| LCL injury: 0-1 | Morton’s neuroma: 2 |
| Popliteal injury: 0-1 | Deltoid ligament injury: 1 |
| Plica syndrome: 0 | Spring ligament injury: 1 |
| Full/partial ACL tear: 0 | Anterolateral ankle impingement: 0 |
| PCL tear: 0 | Posterior talofibular ligament injury: 0 |
| Medial/lateral meniscus tear: 0 |
|
| Osteochondritis dissecans: 0 |
|
Abbreviations: ACL, anterior cruciate ligament; IT, iliotibial; LCL, lateral collateral ligament; MCL, medial collateral ligament; PCL, posterior cruciate ligament.
ULTRASOUND-GUIDED THERAPEUTIC PROCEDURES
The use of US at the point of care for needle guidance has led to its widespread application for therapeutic procedures, including injections and multiple regenerative therapies. Intra-articular US-guided injection and aspiration are common therapeutic interventions performed in the clinical setting. In a position statement of the American Medical Society for Sports Medicine, US-guided injections were found to be more accurate (SORT A evidence), effective (SORT B evidence), and cost effective (SORT B evidence) than landmark-guided injections.3 A recent meta-analysis conducted by Daniels and colleagues1 demonstrated the improved accuracy and efficacy of US-guided injections at the knee, ankle, and foot. Injections may serve a diagnostic purpose when anesthetics, such as lidocaine, are used in isolation, a therapeutic purpose, or both.
Continue to: Percutaneous tenotomy involve...
REGENERATIVE THERAPIES FOR MUSCULOSKELETAL CONDITIONS
PERCUTANEOUS TENOTOMY
Percutaneous tenotomy involves the introduction of a needle into damaged soft tissues, most often tendons (“needling”), in an effort to stimulate a healing response and resect the diseased tendon tissue. Although tenotomy was initially performed as an open or arthroscopic surgical technique, advances in US technology have led to improved sensitivity and specificity identifying areas of tendinous injury (hypervascularity, hypoechogenicity, and calcification); as such, the combination of these techniques has been used in the outpatient setting. New commercial models incorporate ultrasound guidance with needles or micro-resection probes for real-time débridement of damaged tissues. Percutaneous tenotomy has been described in the management of tendinopathy involving the rotator cuff, medial and lateral epicondyles, patellar and Achilles tendons, and plantar fascia.
Housner and colleagues9 evaluated the safety and short-term efficacy of US-guided needle tenotomy in 13 patients with chronic tendinosis of the patella, Achilles tendon, gluteus medius, iliotibial tract, hamstring, and rectus femoris. They reported no procedural complications and a significant decrease in pain scores at 4 and 12 weeks of follow-up.
Koh and colleagues10 conducted a prospective case series to evaluate the safety and efficacy of office-based, US-guided percutaneous tenotomy (using a commercial model) on 20 patients with chronic lateral epicondylitis. The authors reported no wound complications and significant improvement in pain scores at each follow-up period up to 1 year. Subsequent post-procedural US evaluation of injured tissues revealed evidence of healing (decreased tendon thickness, vascularity, and hypoechogenicity) in over half the cohort after 6 months compared with the baseline.11
Lee and colleagues12 evaluated the efficacy of US-guided needle tenotomy combined with platelet-rich plasma (PRP) injection on chronic recalcitrant gluteus medius tendinopathy. In this case series, 21 patients underwent PRP and “needling” through the hypoechoic regions of the injured tendon under direct US guidance. After a period of rest, all patients completed the structured rehabilitation protocol. After an average follow-up of 10 months, all patients displayed significant improvements in all outcome questionnaires and did not report any significant adverse events. The authors concluded that tenotomy combined with PRP is a safe and effective method for treatment for recalcitrant gluteus medius tendinopathy.
These studies indicate that US-guided percutaneous tenotomy, alone or in combination with regenerative therapies, such as PRP, is a safe and effective treatment option for various tendinopathies. However, while tenotomy appears safe with promising results and no reported major adverse events, the level of evidence remains low.
ORTHOBIOLOGICS
Orthobiologics are substances composed of biological materials that can be used to aid or even hasten the healing of bones, muscles, tendons, and ligaments. Orthobiologics may contain growth factors, which initiate or stimulate the body’s reparative process; matrix proteins, which serve as scaffolding for healing tissues; or stem cells, specifically adult stem cells, which are multipotent and can differentiate into several cell lines. Adult stem cells are categorized as hematopoietic, neural, epithelial, skin, and mesenchymal types. Mesenchymal stem cells (MSCs) are of particular interest in sports medicine applications because they secrete growth factors and cytokines with trophic, chemotactic, and immunosuppressive properties.13 MSCs are also multipotent and can differentiate into bones, muscles, cartilages, and tendons.14-17MSCs are readily isolated from many sources, including bone marrow, adipose tissues, synovial tissues, peripheral blood, skeletal muscles, umbilical cord blood, and placenta.13,14Several types of regenerative therapies used in orthopedic and sports medicine practice include PRP, stem cell therapy, and amniotic membrane/fluid preparations. While each therapy possesses the potential for promising results, the paucity of research and discrepancies among studies regarding the description of stem cell lines used limit the available evidence on the true clinical benefits of these regenerative therapies.
[HEAD 3] PLATELET-RICH PLASMA
PRP is an autologous product that has been used to stimulate biological factors and promote healing since the 1970s. Through the activation of platelets, PRP improves localized recruitment, proliferation, and differentiation of cells involved in tissue repair. Platelets, which are non-nucleated bodies located in peripheral blood, contain and release 3 groups of bioactive factors that enhance the healing process. Growth factors and cytokines released from alpha-granules play a role in cell proliferation, chemotaxis, cell differentiation, and angiogenesis. Bioactive factors, such as serotonin and histamine, released from dense granules, increase capillary permeability and improve cell recruitment and migration. Adhesion molecules also assist in cell migration and creation of an extracellular matrix, which acts as a scaffold for wound healing.18 Platelets are activated by mechanical trauma or contact with multiple activators, including Von Willebrand factor, collagen, thrombin, or calcium chloride. When activated, platelets release growth factors and cytokines, which create a pro-inflammatory environment that mediates the tissue repair process. After the procedure, the pro-inflammatory environment may result in patient discomfort, which can be managed with ice and acetaminophen. Use of nonsteroidal anti-inflammatory drugs may theoretically inhibit the inflammatory cascade induced by PRP, and they are avoided before and after the procedure, although evidence regarding necessary time frames is lacking.
Continue to: PRP consists of...
PRP consists of the fractionated liquid component of autologous whole blood, which contains increased concentrations of platelets and cytokines. Different methods and commercial preparations are available for collecting and preparing PRP. Variations in the amount of blood drawn, use of anticoagulants, presence or absence of an activating agent, number of centrifuge spins, and overall platelet and white blood cell concentrations lead to difficulty in evaluating and interpreting the available evidence regarding PRP therapy.
In vitro and animal studies demonstrated promising and safe results regarding the healing effect of PRP on injured soft tissues, such as tendons, ligaments, and muscles. In this regard, a number of studies have evaluated the effect of PRP on human MSK injuries. However, in addition to the above-mentioned variabilities in PRP, many of such studies lack standardization and randomization techniques and include a small number of patients only, thereby limiting the overall comparison and clinical application.
A landmark study conducted by Mishra and Pavelko19 concluded that PRP significantly reduced pain in patients with chronic elbow tendinosis. Similar findings were reported in high-level overhead athletes with ulnar collateral ligament insufficiency, which did not improve with conservative management.20 Fitzpatrick and colleagues21 found improvements in pain with the use of single PRP injection as treatment for chronic gluteal tendinopathy. PRP can effectively improve pain and recovery in chronic ligament and tendon injuries, such as lateral epicondylitis, patellar tendinopathy, and plantar fasciitis, when patients are unresponsive to traditional conservative management. The application of PRP to treat acute MSK injuries has produced mixed results. Hamid and colleagues22 conducted a level II randomized controlled trial to evaluate the effect of PRP combined with a rehabilitation program for treatment of grade 2 hamstring injuries on return-to-play compared with rehabilitation alone. Fourteen athletes were randomized into the study and control groups. Hamid and colleagues22 reported improved return-to-play in the study group compared with that in the control (26.7 and 42.5 days, respectively). This study also reported lower pain scores in the PRP group over time, but the difference was not statistically significant. Zanon and colleagues23 conducted a prospective study to evaluate return-to-play in professional soccer players with acute hamstring strains treated with PRP and a rehabilitation program. This study determined that athletes treated with PRP were “match fit,” meaning they would be available for match selection in an average of 36.8 days. However, Zanon and colleagues23 did not include a control group for comparison. Other studies reported that PRP treatment of acutely injured muscles and medial collateral ligaments of soccer and basketball players decreased their return-to-play interval.18 Reviews by Hamilton and colleagues24 and Pas and colleagues25 concluded that PRP treatment of acutely injured tissues with good blood supply (eg, hamstring muscles) did not improve pain or return-to-play compared with standardized rehabilitation protocols. Similarly, in a double-blinded placebo controlled trial, Reurink and colleagues26 evaluated return-to-play in 80 athletes with acute hamstring injuries treated with a rehabilitation program and either PRP or placebo. Reurink and colleagues26 found no difference in return-to-play (42 days for both groups), but the difference was not statistically significant. PRP has also been used intraoperatively and shows promising results in total knee arthroplasty, anterior cruciate ligament reconstruction, acute Achilles tendon repair, rotator cuff repair, and cartilage repair. However, many of these intraoperative studies are limited to animal models.
In 2009, the World Anti-Doping Agency (WADA) prohibited the use of PRP because it contains autologous growth factors and IGF-1, which could produce an anabolic effect. Recent studies have failed to demonstrate any athletic advantages of using PRP. WADA has since removed PRP from its prohibited list. PRP is also not prohibited by the US Anti-Doping Agency (USADA) and many major professional sporting leagues in the United States. However, care must be taken in reviewing the components of PRP because many commercially available products differ in PRP formulation. Since 2010, many team physicians have increasingly used PRP to treat a wide range of athletic injuries. A recent anonymous survey conducted by a team of physicians on PRP use in elite athletes revealed minimal complications but significant variability among physicians with regard to timing, belief in evidence, and formulation and dosing of PRP treatments. Many physicians did implicate athlete desire as the main indication for treatment.27
As an autologous treatment, PRP injection has no serious adverse effects beyond mild discomfort as a result of the procedure and pro-inflammatory state in the days following injection. Recent concerns regarding the potential of PRP treatment for heterotopic ossification have been reported, but published information is limited to case reports. PRP can improve pain and function in patients with chronic MSK injury. PRP appears to be a safe and effective alternative to surgery for patients with injury to poorly perfused tissue, which has not improved with conservative measures, such as rest, physical therapy, and anti-inflammatory medications. Care should be taken when treating athletes with PRP to establish regulations on doping by individual governing bodies.
Continue to: Use of stem...
STEM CELL THERAPY
Use of stem cell therapy is based on the properties of the proliferation and differentiation of multipoint MSC lines. These stem cells can theoretically regenerate injured tissues and influence repair through immunomodulation; paracrine activity through the release of bioactive agents, such as cytokines, trophic, and chemotactic molecules; and cell differentiation into various cell lineages.15,16,13,17 Orthopedic surgeons have used microfracture to recruit MSCs during cartilage repair procedures for over 20 years. This procedure draws multipotent MSCs to the injured site to induce chondrogenic proliferation and fibrocartilage repair.28
Adult MSCs provide a readily accessible autologous source of stem cells for regenerative therapies. MSCs can be isolated from a variety of tissues, including bone marrow, adipose tissues, synovia, human umbilical cord blood, and peripheral blood. The majority of stem cell therapies in the United States for sports medicine purposes are conducted using bone marrow aspirate concentrate (BMAC) and adipose tissues. The US Food and Drug Administration (FDA) allows the use of minimally manipulated autologous stem cells to be injected into the same patient on the same day. However, some studies reported that culturing stem cells or introducing products, such as collagenase to stem cells, can increase the stem cell concentration prior to injection. These processes constitute more than “minimal manipulation” and therefore would require drug trials prior to use in the United States.
Although MSCs can be readily obtained from a variety of tissue sources, the makeup of the cell concentrate differs. Bone marrow and adipose tissues are readily available sources of homogenous MSCs. Harvesting stem cells from adipose tissues provides a less invasive route of collection than from BMAC. Harvested BMAC and adipose tissues consist of heterogeneous cell populations that are composed of precursor and accessory cells, such as pericytes, endothelial cells, smooth muscle cells, fibroblasts, and macrophages in addition to MSCs.
Animal studies reported promising results when evaluating soft tissue lesions in small and large animal models.14,15 Although clinical and human evidence remains limited, the potential of MSCs for regenerative repair has led to a recent increase in the number of related clinical studies. Multiple systematic reviews have concluded that MSC therapy is safe for the treatment of osteoarthritis, cartilage lesions, and tendinopathies. Limited evidence is available regarding the safety of intramuscular use, and a theoretical concern arises on the development of heterotopic bone formation as a result of treatment.13,16 The efficacy of MSC therapy is difficult to determine due to the lack of standardization in stem cell populations, adjuvants (eg, PRP, hyaluronic acid, and scaffolding preparations), and delivery methods used.13,17
Similar to PRP, the increased use of MSC therapy among high-profile athletes has led to the promotion of these therapies as safe and effective despite limited evidence.29 Although MSC therapy is a promising and safe treatment option for patients with soft tissue injuries, the paucity in data and human studies limit its clinical use. Moreover, data of MSC efficacy is complicated because of the disparity between clinical studies regarding MSC collection method (many of which eclipse the “minimal manipulation” standard), description of isolated cell concentrates, dosage, method of delivery, use of adjuvants, and lack of randomization. Further studies using [standardized] methods are needed before establishing a true consensus on the safety and efficacy of MSC therapy.
AMNIOTIC MEMBRANE
The placenta is a source of MSCs, a collagen-rich extracellular matrix, and bioactive growth and regulatory factors. The capacity of the placenta to modulate biological activities and tissue formation is thought to provide a means of tissue repair and healing. The placenta consists of amniotic fluid, amniotic membrane (AM), chorionic membrane, and umbilical cord blood and tissues. Although MSCs have been isolated from each component of placental tissues, amniotic and chorionic membranes and umbilical cord tissues yield the highest concentration.
The majority of regenerative studies involving the placenta used AM alone or in combination with other placental tissues. AM is a metabolically active tissue that consists of an epithelial layer, a basement membrane, and a mesenchymal tissue layer. In addition to being a source of stem cells, AM synthesizes many growth factors, vasoactive peptides, and cytokines, which are capable of tissue regeneration. AM was initially used as a biological scaffold for the treatment of skin burns and wounds. Other intrinsic properties of AM include the provision of a matrix for cellular migration and proliferation, enhanced wound healing with reduced scar formation, antibacterial activity, and lastly, non-immunogenic and immunosuppressive properties. These inherent characteristics have spurred studies on the potential use of AM in sports medicine as a minimally invasive means to treat osteoarthritis and injuries of tendons, ligaments, muscles, fascia, and cartilages.
Continue to: Animal studies reported...
Animal studies reported positive results with the use of AM to treat osteoarthritis, cartilage defects, and tendon and ligament injuries. Few studies involving human participants also revealed favorable results with regard to the use of AM for the treatment of plantar fasciitis and osteoarthritis; however, these studies are industry-sponsored and employed small sample sizes. The unique mixture of a collagen-rich extracellular matrix, bioactive growth factors, and pluripotent stem cells may allow AM to become an effective treatment for MSK injuries. Although initial animal and human studies show promising results, variabilities regarding models (animal and human), pathologies, placental tissues, and methods of preparation, preservation, and delivery used limit the ability for comparison, analysis, and drawing of definitive conclusions. Thus far, no studies have evaluated the use of currently available AM products for the treatment of injuries sustained by soccer players.
Despite the current popularity of AM as regenerative therapy in academic research and potential use in clinical treatment in sports medicine, physicians should remain aware of the limited evidence available. Other barriers to research and use AM as a regenerative therapy include regulatory classifications based on the concept of “minimal manipulation” in biologic therapies. Minimally manipulated placental allografts are less regulated, less costly to study, and more easily commercialized. These products are not required to undergo FDA phase I to III trials prior to premarket approval. In 2000, the FDA position on all AM products falls into 2 categories. The first position states that AM that contains allogenic stem cells mixed with another drug that is micronized and/or cryopreserved is more than “minimally manipulated” and therefore categorized as “biologic” and would be subject to phase I to III trials. Dehydrated and decellularized AM, however, may meet the concept of minimal manipulation and is only approved by the FDA as a wound covering. Thus, any application of AM for the treatment of sports medicine pathology is not currently FDA-approved, considered off-label, not covered by insurance, and subject to out-of-pocket pay.30,31
CONCLUSION
With improvements in technology and portability, US has become an effective imaging modality for point-of-care evaluation, diagnosis, and continuous monitoring of many MSK injuries. Additionally, as a dynamic imaging modality, US allows for increased accuracy and efficacy when combined with minimally invasive procedures, such as diagnostic and therapeutic guided injections and percutaneous tenotomy, in the clinical setting; thereby decreasing the overall healthcare costs. PRP is proven to be a safe treatment for several MSK conditions, such as lateral epicondylitis, patellar tendonitis, and plantar fasciitis. Although PRP has been included in the standard of care in some areas, this technique may be predominantly athlete driven. Conflicting evidence with regard to return-to-play timeframes following PRP treatment for muscular injuries and poor evidence in conditions, such as Achilles tendonitis, have led to inconsistent indications for use, dose, and timing of treatment. Although early evidence of MSC therapy is promising, high-level evidence for MSC therapy is insufficient, despite its increased use among athletes. Thus far, no data are available regarding the outcomes of the use of amniotic products for the treatment of injuries among athletes. Furthermore, the preparation of amniotic products has many regulatory concerns. The authors advocate for continuous high-level research on regenerative medicine therapies to establish clinical efficacy and safety data.
1. Daniels E, Cole D, Jacobs B, Phillips S. Existing Evidence on ultrasound-guided injections in sports medicine. Orthop J Sports Med. 2018;6(2):2325967118756576. doi:10.1177/2325967118756576.
2. Henne M, Centurion A, Rosas S, Youmans H, Osbahr D. Trends in utilization of image-guided hip joint injections. Unpublished. 2018.
3. Finnoff JT, Hall MM, Adams E, et al. American Medical Society for Sports Medicine position statement: Interventional musculoskeletal ultrasound in sports medicine. Clin J Sport Med. 2015;25:6-22. doi:10.1097/JSM.0000000000000175.
4. Agel J, Evans TA, Dick R, Putukian M, Marshal S. Descriptive epidemiology of collegiate men’s soccer injuries: National Collegiate Athletic Association Injury Surveillance System, 1988-1989 through 2002-2003. J Athl Train. 2007;42(2):270-277.
5. Dick R, Putukian M, Agel J, Evans T, Marshall S. Descriptive epidemiology of collegiate women’s soccer injuries: National Collegiate Athletic Association Injury Surveillance System, 1988-1989 through 2002-2003. J Athl Train. 2007;42(2):278-285.
6. Ekstrand J, Hagglund M, Walden M. Epidemiology of muscle injuries in professional football (soccer). Am J Sports Med. 2011;39(6):1226-1232. doi:10.1177/0363546510395879.
7. Klauser A, Tagliafico A, Allen G, et al. Clinical indications for musculoskeletal ultrasound: A Delphi-based consensus paper of the European society of musculoskeletal radiology. Eur Radiol. 2012;22(5):1140-1148. doi:10.1007/s00330-011-2356-3.
8. Henderson R, Walker B, Young K. The accuracy of diagnostic ultrasound imaging for musculoskeletal soft tissue pathology of the extremities: a comprehensive review of the literature. Chiropr Man Therap. 2015;23(1):31. doi:10.1186/s12998-015-0076-5.
9. Housner JA, Jacobson JA, Misko R. Sonographically guided percutaneous needle tenotomy for the treatment of chronic tendinosis. J Ultrasound Med. 2009;28(9):1187-1192. doi:10.7863/jum.2009.28.9.1187.
10. Koh J, Mohan PC, Howe TS, et al. Fasciotomy and surgical tenotomy for recalcitrant lateral elbow tendinopathy: early clinical experience with a novel device for minimally invasive percutaneous microresection. Am J Sports Med. 2013;41(3):636-644. doi:10.1177/0363546512470625.
11. Seng C, Mohan PC, Koh J, et al. Ultrasonic percutaneous tenotomy for recalcitrant lateral elbow tendinopathy: sustainability and sonographic progression at 3 years. Am J Sports Med. 2015;44(2):504-510. doi:10.1177/0363546515612758.
12. Lee J, Harrison J, Boachie-Adjei K, Vargas E, Moley P. Platelet-rich plasma injections with needle tenotomy for gluteus medius tendinopathy: A registry study with prospective follow-up. Orthop J Sports Med. 2016;4(11):2325967116671692. doi:10.1177/2325967116671692.
13. Osborne H, Anderson L, Burt P, Young M, Gerrard D. Australasian College of Sports Physicians-Position statement: the place of mesenchymal stem/stromal cell therapies in sport and exercise medicine. Br J Sports Med. 2016;50:1237-1244. doi:10.1136/bjsports-2015-095711.
14. Anderson J, Little D, Toth A, et al. Stem cell therapies for knee cartilage repair. The current status of preclinical and clinical studies. Am J Sports Med. 2013;42(9)2253-2261. doi:10.1177/0363546513508744.
15. Lee S, Kwon B, Lee Kyoungbun, Son Y, Chung S. Therapeutic mechanisms of human adipose-derived mesenchymal stem cells in a rat tendon injury model. Am J Sports Med. 2017;45(6):1429-1439. doi:10.1177/0363546517689874.
16. McIntyre J, Jones I, Han B, Vangsness C. Intra-articular mesenchymal stem cell therapy for the human joint. A systematic review. Am J Sports Med. 2017;0363546517735844. doi:10.1177/0363546517735844.
17. Pas HIMFL, Moen M, Haisma J, Winters M. No evidence for the use of stem cell therapy for tendon disorders: a systematic review. Br J Sports Med. 2017;51:996-1002. doi:10.1136/bjsports-2016-096794.
18. Foster T, Puskas B, Mandelbaum B, Gerhardt M, Rodeo S. Platelet-rich plasma: from basic science to clinical applications. Am J Sports Med. 2009;37(11):2259-2272. doi:10.1177/0363546509349921.
19. Mishra A, Pavelko T. Treatment of chronic elbow tendinosis with buffered platelet-rich plasma. Am J Sports Med. 2006;34(11):1774-1778. doi:10.1177/0363546506288850.
20. Dines J, Williams P, ElAttrache N, et al. Platelet-rich plasma can be used to successfully treat elbow ulnar collateral ligament insufficiency in high-level throwers. Am J Orthop. 2016;45(4):296-300.
21. Fitzpatrick J, Bulsara M, O’Donnel J, McCrory P, Zheng M. The effectiveness of platelet-rich plasma injections in gluteal tendinopathy. A randomized, double-blind controlled trial comparing a single platelet-rich plasma injection with a single corticosteroid injection. Am J Sports Med. 2018;46(4)933-939. doi:10.1177/0363546517745525.
22. Hamid M, Ali M, Yusof A, George J, Lee L. Platelet-rich plasma injections for the treatment of hamstring injuries: A randomized controlled trial. Am J Sports Med. 2014;42(10):2410-2418. doi:10.1177/0363546514541540.
23. Zanon G, Combi F, Combi A, Perticarini L, Sammarchi L, Benazzo F. Platelet-rich plasma in the treatment of acute hamstring injuries in professional football players. Joints. 2016;4(1):17-23. doi:10.11138/jts/2016.4.1.017.
24. Hamilton B, Tol JL, Almusa E, et al. Platelet-rich plasma does not enhance return to play in hamstring injuries: a randomized controlled trial. Br J Sports Med. 2015;49:943-950. doi:10.1136/bjsports-2015-094603.
25. Pas HIMFL, Reurink G, Tol JL, Wier A, Winters M, Moen M. Efficacy of rehabilitation (lengthening) exercises, platelet-rich plasma injections, and other conservative interventions in acute hamstring injuries: an updated systematic review and meta-analysis. Br J Sports Med. 2015;49:1197-1205. doi:10.1136/bjsports-2015-094879.
26. Reurink G, Goudswaard G, Moen M, et al. Platelet-rich plasma injections in acute muscle injury. N Engl J Med. 2014;370:2546-2547. doi:10.1056/NEJMc1402340.
27. Kantrowitz D, Padaki A, Ahmad C, Lynch T. Defining platelet-rich plasma usage by team physicians in elite athletes. Orthop J Sports Med. 2018;6(4):2325967118767077. doi:10.1177/2325967118767077.
28. Mithoefer K, Peterson L, Zenobi-Wong M, Mandelbaum B. Cartilage issues in football-today’s problems and tomorrow’s solutions. Br J Sports Med. 2015;49(9):590-596. doi:1136/bjsports-2015-094772.
29. Matthews K, Cuchiara M. Regional regulatory insights: U.S. National Football League Athletes seeking unproven stem cell treatments. Stem Cells Dev. 2014;23(S1):60-64. doi:10.1089/scd.2014.0358.
30. McIntyre J, Jones I, Danilkovich A, Vangsness T. The placenta: applications in orthopaedic sports medicine. Am J Sports Med. 2018;46(1):234-247. doi:10.1177/0363546517697682.
31. Riboh J, Saltzman B, Yankee A, Cole BJ. Human amniotic membrane-derived products in sports medicine: Basic science, early results, and potential clinical applications. Am J Sports Med. 2015;44(9)2425-2434. doi:10.1177/0363546515612750.
1. Daniels E, Cole D, Jacobs B, Phillips S. Existing Evidence on ultrasound-guided injections in sports medicine. Orthop J Sports Med. 2018;6(2):2325967118756576. doi:10.1177/2325967118756576.
2. Henne M, Centurion A, Rosas S, Youmans H, Osbahr D. Trends in utilization of image-guided hip joint injections. Unpublished. 2018.
3. Finnoff JT, Hall MM, Adams E, et al. American Medical Society for Sports Medicine position statement: Interventional musculoskeletal ultrasound in sports medicine. Clin J Sport Med. 2015;25:6-22. doi:10.1097/JSM.0000000000000175.
4. Agel J, Evans TA, Dick R, Putukian M, Marshal S. Descriptive epidemiology of collegiate men’s soccer injuries: National Collegiate Athletic Association Injury Surveillance System, 1988-1989 through 2002-2003. J Athl Train. 2007;42(2):270-277.
5. Dick R, Putukian M, Agel J, Evans T, Marshall S. Descriptive epidemiology of collegiate women’s soccer injuries: National Collegiate Athletic Association Injury Surveillance System, 1988-1989 through 2002-2003. J Athl Train. 2007;42(2):278-285.
6. Ekstrand J, Hagglund M, Walden M. Epidemiology of muscle injuries in professional football (soccer). Am J Sports Med. 2011;39(6):1226-1232. doi:10.1177/0363546510395879.
7. Klauser A, Tagliafico A, Allen G, et al. Clinical indications for musculoskeletal ultrasound: A Delphi-based consensus paper of the European society of musculoskeletal radiology. Eur Radiol. 2012;22(5):1140-1148. doi:10.1007/s00330-011-2356-3.
8. Henderson R, Walker B, Young K. The accuracy of diagnostic ultrasound imaging for musculoskeletal soft tissue pathology of the extremities: a comprehensive review of the literature. Chiropr Man Therap. 2015;23(1):31. doi:10.1186/s12998-015-0076-5.
9. Housner JA, Jacobson JA, Misko R. Sonographically guided percutaneous needle tenotomy for the treatment of chronic tendinosis. J Ultrasound Med. 2009;28(9):1187-1192. doi:10.7863/jum.2009.28.9.1187.
10. Koh J, Mohan PC, Howe TS, et al. Fasciotomy and surgical tenotomy for recalcitrant lateral elbow tendinopathy: early clinical experience with a novel device for minimally invasive percutaneous microresection. Am J Sports Med. 2013;41(3):636-644. doi:10.1177/0363546512470625.
11. Seng C, Mohan PC, Koh J, et al. Ultrasonic percutaneous tenotomy for recalcitrant lateral elbow tendinopathy: sustainability and sonographic progression at 3 years. Am J Sports Med. 2015;44(2):504-510. doi:10.1177/0363546515612758.
12. Lee J, Harrison J, Boachie-Adjei K, Vargas E, Moley P. Platelet-rich plasma injections with needle tenotomy for gluteus medius tendinopathy: A registry study with prospective follow-up. Orthop J Sports Med. 2016;4(11):2325967116671692. doi:10.1177/2325967116671692.
13. Osborne H, Anderson L, Burt P, Young M, Gerrard D. Australasian College of Sports Physicians-Position statement: the place of mesenchymal stem/stromal cell therapies in sport and exercise medicine. Br J Sports Med. 2016;50:1237-1244. doi:10.1136/bjsports-2015-095711.
14. Anderson J, Little D, Toth A, et al. Stem cell therapies for knee cartilage repair. The current status of preclinical and clinical studies. Am J Sports Med. 2013;42(9)2253-2261. doi:10.1177/0363546513508744.
15. Lee S, Kwon B, Lee Kyoungbun, Son Y, Chung S. Therapeutic mechanisms of human adipose-derived mesenchymal stem cells in a rat tendon injury model. Am J Sports Med. 2017;45(6):1429-1439. doi:10.1177/0363546517689874.
16. McIntyre J, Jones I, Han B, Vangsness C. Intra-articular mesenchymal stem cell therapy for the human joint. A systematic review. Am J Sports Med. 2017;0363546517735844. doi:10.1177/0363546517735844.
17. Pas HIMFL, Moen M, Haisma J, Winters M. No evidence for the use of stem cell therapy for tendon disorders: a systematic review. Br J Sports Med. 2017;51:996-1002. doi:10.1136/bjsports-2016-096794.
18. Foster T, Puskas B, Mandelbaum B, Gerhardt M, Rodeo S. Platelet-rich plasma: from basic science to clinical applications. Am J Sports Med. 2009;37(11):2259-2272. doi:10.1177/0363546509349921.
19. Mishra A, Pavelko T. Treatment of chronic elbow tendinosis with buffered platelet-rich plasma. Am J Sports Med. 2006;34(11):1774-1778. doi:10.1177/0363546506288850.
20. Dines J, Williams P, ElAttrache N, et al. Platelet-rich plasma can be used to successfully treat elbow ulnar collateral ligament insufficiency in high-level throwers. Am J Orthop. 2016;45(4):296-300.
21. Fitzpatrick J, Bulsara M, O’Donnel J, McCrory P, Zheng M. The effectiveness of platelet-rich plasma injections in gluteal tendinopathy. A randomized, double-blind controlled trial comparing a single platelet-rich plasma injection with a single corticosteroid injection. Am J Sports Med. 2018;46(4)933-939. doi:10.1177/0363546517745525.
22. Hamid M, Ali M, Yusof A, George J, Lee L. Platelet-rich plasma injections for the treatment of hamstring injuries: A randomized controlled trial. Am J Sports Med. 2014;42(10):2410-2418. doi:10.1177/0363546514541540.
23. Zanon G, Combi F, Combi A, Perticarini L, Sammarchi L, Benazzo F. Platelet-rich plasma in the treatment of acute hamstring injuries in professional football players. Joints. 2016;4(1):17-23. doi:10.11138/jts/2016.4.1.017.
24. Hamilton B, Tol JL, Almusa E, et al. Platelet-rich plasma does not enhance return to play in hamstring injuries: a randomized controlled trial. Br J Sports Med. 2015;49:943-950. doi:10.1136/bjsports-2015-094603.
25. Pas HIMFL, Reurink G, Tol JL, Wier A, Winters M, Moen M. Efficacy of rehabilitation (lengthening) exercises, platelet-rich plasma injections, and other conservative interventions in acute hamstring injuries: an updated systematic review and meta-analysis. Br J Sports Med. 2015;49:1197-1205. doi:10.1136/bjsports-2015-094879.
26. Reurink G, Goudswaard G, Moen M, et al. Platelet-rich plasma injections in acute muscle injury. N Engl J Med. 2014;370:2546-2547. doi:10.1056/NEJMc1402340.
27. Kantrowitz D, Padaki A, Ahmad C, Lynch T. Defining platelet-rich plasma usage by team physicians in elite athletes. Orthop J Sports Med. 2018;6(4):2325967118767077. doi:10.1177/2325967118767077.
28. Mithoefer K, Peterson L, Zenobi-Wong M, Mandelbaum B. Cartilage issues in football-today’s problems and tomorrow’s solutions. Br J Sports Med. 2015;49(9):590-596. doi:1136/bjsports-2015-094772.
29. Matthews K, Cuchiara M. Regional regulatory insights: U.S. National Football League Athletes seeking unproven stem cell treatments. Stem Cells Dev. 2014;23(S1):60-64. doi:10.1089/scd.2014.0358.
30. McIntyre J, Jones I, Danilkovich A, Vangsness T. The placenta: applications in orthopaedic sports medicine. Am J Sports Med. 2018;46(1):234-247. doi:10.1177/0363546517697682.
31. Riboh J, Saltzman B, Yankee A, Cole BJ. Human amniotic membrane-derived products in sports medicine: Basic science, early results, and potential clinical applications. Am J Sports Med. 2015;44(9)2425-2434. doi:10.1177/0363546515612750.
TAKE-HOME POINTS
- Improvements in ultrasound technology have increased its use as a therapeutic and diagnostic modality.
- Ultrasound offers increased accuracy and efficacy with minimally invasive procedures.
- PRP is a safe and effective treatment for many musculoskeletal injuries, however return-to-play time frames limit its efficacy.
- While stem cell and amniotic products offer promising results, the paucity in data limits overall use.
- Care should be taken when discussing regenerative therapy as many products eclipse the concept of “minimal manipulation” and therefore require USFDA trials to establish safety data.
The In Vivo Impact of Leukocyte Injections on Normal Rat Achilles Tendons: Potential Detriment to Tendon Morphology, Cellularity, and Vascularity
ABSTRACT
In this study, we determine the in vivo effects of injecting sub-populations of leukocytes into normal rat Achilles tendons via a controlled laboratory study. Allogenic monocytes, granulocytes, or plasma were injected into 24 healthy rat Achilles tendons. Treated and contralateral un-treated control tendons then assessed for cellularity, histologic morphology, and vascularity after 7 and 14 days. Significant increases of 221% and 249% in cellularity (P = 0.014) were seen on day 14 within Achilles tendons injected with granulocytes as compared to plasma and monocytes, respectively. Also, significant improvement in morphology (P = 0.029) between days 7 and 14 was seen for the granulocyte injected Achilles tendons. Significant increases in cellularity after an injection of granulocytes, compared to monocytes and plasma, corresponds to a significant increase in inflammation within the tissue, suggesting that leukocyte-rich platelet-rich plasma (PRP) preparations are proinflammatory and potentially catabolic when injected into tendon tissue. The concentration and composition of white blood cells within PRP preparations is variable and needs to be better understood in order to optimize clinical utility of PRP injections.
Continue to: Tendinopathies are debilitating conditions...
Tendinopathies are debilitating conditions affecting patients worldwide every day. They arise most frequently from tendon overuse resulting in pathology.1 There are 2 major subtypes of tendinopathy: tendinosis and tendinitis. Tendinosis, the more common condition, is characterized by long-term, chronic degradation of tendon tissue resulting in fibrosis from infiltrating fibroblasts.2 Tendinitis, the less common condition, is characterized by an acute inflammatory response and inflammatory cell infiltrate.2 Both conditions are common, with Achilles tendinopathy affecting 11% of runners and lateral epicondylitis affecting 1% to 3% of the general population.3,4 Many sports-related overuse injuries, such as tendinopathies, go undiagnosed for extended periods of time because medical attention is avoided in order to prevent time loss from training or competing.5 These delays could be eliminated if a non-surgical option for treating tendon pathology was available.
Tendinopathies are believed to result from tendon overuse that causes micro-damage to collagen, as well as from significant changes in protein and enzyme composition within the tendon.6 The damage accumulates over time and eventually leads to chronic inflammation or fibrotic change within tendons, in both cases weakening the tendon and causing pain. Currently, accepted treatments for tendinopathies include: nonsteroidal anti-inflammatory drugs, physical therapy, ultrasound, laser-therapy, corticosteroids, glyceryl trinitrate patches, extracorporeal shock wave therapy, sclerotherapy, and surgery.7 Recently, platelet-rich plasma (PRP) therapy has emerged as a promising treatment for tendinopathies, as well as a variety of other orthopedic indications.
PRP consists of autologous blood from the patient, centrifuged to increase the amount of platelets in the sample above baseline, and subsequently injected around an affected tendon or joint.8 PRP is used to treat tendinopathy because it can supply injured tendons with blood components that aid in healing, which tendons do not receive due to poor vascularity.9 These components include growth factors, such as platelet derived growth factor (PDGF), transforming growth factor-β (TGF-β), vascular endothelial growth factor (VEGF), endothelial growth factor, and leukocytes that can stimulate an inflammatory response within the injured tissue.10 The inflammatory response from the PRP induces a more robust reconstruction and revascularization of the injured tissue, stimulating proliferation, and remodeling.11,12However, significant variability exists within the platelets, leukocytes, and growth factors that comprise PRP. This is attributed to 3 major causes. First, current commercial preparations of PRP result in differing platelet concentrations, as well as leukocyte-rich and leukocyte-poor compositions.13,14 Variability in platelet concentrations results in unreliable amounts of growth factors, including cytokines, TGF-β, PDGF, VEGF and basic fibroblast growth factor in each preparation, while leukocyte levels affect inflammation, all leading to variable effects for each preparation.15,16Second, despite sex and age of the PRP donor not being significant factors influencing variation in growth factor concentrations, the existence of an unexplained variation in concentrations of growth factors between different donors has been observed.17 Third, the selection of activating agents, bovine thrombin or calcium chloride, and their application, whether to the elbow, shoulder, or knee, produces variability.18
While the effects of platelets and growth factors in PRP have been well studied, less is known about the effects of differing cell types. Recently it was reported that the concentrations of leukocytes directly affect the outcomes of PRP injections. McCarrel and colleagues19,20 found that as the number of leukocytes increased, there was a concomitant increase in the expression of inflammatory cytokines and catabolic activity. This effect may result in inferior healing of injured tissues and is attributed to the release of pro-inflammatory cytokines such as interleukin-1β from the leukocytes.21 There is also evidence that minimizing the catabolic effect of leukocytes may be just as important to tissue healing as the maximizing anabolic effect of platelets and growth factors.22
The use of PRP has been highly disputed in recent years due to conflicting reports of its success in treating orthopedic conditions. Numerous favorable studies have shown benefit for treating chronic and acute orthopedic injuries including; rotator cuff tear repair, chronic refractory patellar tendinopathy, and chronic lateral tendinosis/epicondylitis.23-26 Concurrently, articles demonstrating no significant effects from PRP have also been published. One study claiming that PRP injections did not improve outcomes of chronic Achilles tendinopathy did not differentiate whether patients had tendinosis or tendinitis, and did not consider leukocyte concentration in their PRP preparations27 Another study that determined PRP is not beneficial to the healing of ruptured Achilles tendons after surgical repair also failed to consider the concentration of leukocytes in their PRP preparations.28 One of the difficulties in comparing these studies is their heterogeneous nature. This arises from the use of different conditions in each study that makes the studies incomparable. Variations in PRP preparations lead to different concentrations of growth factors, platelets, and leukocyte concentrations. Additionally, tendinopathy models were not specified as tendinosis and tendonitis, and models or patients were not controlled for age, sex, or comorbidities. Given that leukocyte-rich and leukocyte-poor PRP preparations are currently widely used in clinical practice, the discovery of which type of preparation is indicated in which setting is paramount to evidence-based use of this treatment modality. Due to reports suggesting that leukocytes may be detrimental to tendon healing, determining which types of leukocytes are responsible for these effects is vital. As such, the purpose of this study is to determine the in vivo effects of sub-populations of leukocytes on normal rat tendons. This study design allowed us to isolate the effects of the injections to induce a response and remove confounding effects of normal healing response to a damaged tendon and effects from the injection itself. Our hypothesis was that the injection of leukocytes would cause an inflammatory response in rat tendons, leading to catabolic outcomes.
Continue to: METHODS...
METHODS
This was a prospective, in vivo, placebo controlled, randomized animal study. The University’s Institutional Animal Care and Use Committee approved all procedures prior to initiation. Twenty-four male Sprague-Dawley rats were randomized to 3 treatment groups (n = 8): monocytes; granulocytes, and; plasma, as a negative control.
Allogenic blood from 6 additional rats was collected into K2EDTA tubes via cardiac puncture. Allogenic, as opposed to autogenic, blood is commonly used in rat models because of low immunogenic response to blood from rats of the same strain and litter.29,30 The blood was then pooled and the red cells lysed by incubation with Red Blood Cell Lysis Buffer (Roche). The samples were then sorted into fractions containing monocytes and granulocytes using fluorescence activated cell sorting (FACS) using a FACSAria (BD Biosciences). Cells were sorted using Purified PE Mouse Anti-Rat CD11b/c antibodies (BD Pharmingen) specific to monocytes, granulocytes, macrophages, dendritic cells, and microglia, APC-Cy7 Mouse Anti-Rat CD45 antibodies (BD Pharmingen) specific to all hematopoietic cells except erythrocytes, and FITC Mouse Anti-Rat CD42d antibodies (BD Pharmingen) specific to megakaryocytes and platelets. 20 μL of 0.2 mg/mL CD11b/c, 20 μL of 0.2 mg/mL CD 45, and 10 μL of 0.5 mg/mL CD42d antibodies were added to 1 mL of condensed non-red cells collected from the 6 rats and incubated at room temperature in the dark for 15 minutes. A fraction containing only platelet-poor plasma was also collected. For all treatments the injection volume was 75 μL. Rats in the monocyte group were injected with 200,000 cells in platelet-poor plasma, those in the granulocyte group were injected with 900,000 cells in platelet-poor plasma, and rats in the plasma control group received only platelet-poor plasma. The cell concentrations were based on previous studies that documented these concentrations that are found in typical leukocyte-rich PRP preparations.13
The animals were anesthetized with isoflurane gas and then injected aseptically once into their right Achilles tendon. The left Achilles tendon was used as an un-injected control, giving a total of 48 total Achilles tendons studied. At days 7 and 14 post-injection, 4 rats from each group were euthanized and the Achilles tendons were harvested.
The tendons were fixed in neutral buffered formalin for 24 hours and then embedded in paraffin and sectioned sagittally at 12 μm. The tendons were then stained with hematoxylin and eosin (H&E) using standard histological protocols and examined by 3 individuals trained to assess cellularity and morphology. All samples were assigned unrecognizable numbers and randomized prior to examination by individuals. Cell counts were based on the number of nuclei present in 3 mid-tendon high-power fields (400x) per sample. Morphology was graded on a scale of 1 to 3, with 1 being a normal tendon and 3 having severe pathology with total loss of alignment and crimping on 3 low-power fields (100x) per sample (Figures 1A-1G).
Vascularity was assessed by immunohistochemical staining using Rabbit Polyclonal Anti-CD31 antibodies (Abcam), a marker for vascular endothelial cells, using a Vectastain ABC Kit (Vector Laboratories) system and the ImmPACT AEC Peroxidase (HRP) Substrate (Vector Laboratories). Following staining, automated image analysis was performed (Bioquant). Briefly, all areas that did not contain tendon were masked. CD31 positive areas were then quantified using global thresholding. Vascularity was then calculated as ratio of CD31 positive area to total tendon area. Analyses were performed on 3 mid-tendon medium-power (200x) fields per sample.
For cellularity and morphology, the results for the injected tendons were normalized to those of their contralateral untreated controls and reported as a percentage. Results for vascularity were compared directly between treated tendons. Differences were assessed between groups at each time-point using Independent Samples Median Tests. When significant differences were identified, pairwise comparisons were performed to identify the source of the differences. All analyses were conducted using SPSS (V22, SAS Institute) with significant differences determined for values of P < 0.05.
RESULTS
No significant differences in cellularity between groups were seen at day 7 (P = 0.368) (Figures 1A-1G). However, a significant difference in cellularity between groups was seen at day 14 (P = 0.014). Pairwise tests showed there to be a significant increase in the number of cells in the tendons treated with granulocytes from 221% and 249% in cellularity (P = 0.014) on day 14, as compared to both monocytes and plasma, respectively. Morphologically, no significant differences were seen between groups at either time-point (P = 0.091 for day 7 and P = 1.000 for day 14) (Figures 2A-2G). However, a significant improvement in morphology was observed from day 7 to day 14 in the granulocyte group from 60% to 165% (P = 0.029). Finally, no differences were seen in vascularity between treatment groups at either time-point (P = 0.368 for day 7 and P = 0.535 for day 14) (Figures 3A-3G).
Continue to: DISCUSSION...
DISCUSSION
Our hypothesis that the injection of leukocytes would cause an inflammatory response in rat tendons leading to catabolic outcomes was confirmed in the granulocyte group. It should be noted that prior to the catabolic outcome, there was a transient anabolic effect in the granulocyte group during the second week. Deterioration in morphology was observed in the tendons injected with granulocytes on day 7, which subsequently recovered in the following week. We found that injecting granulocytes into normal tendons resulted in an increase in inflammatory cellularity, when compared to monocytes and plasma injections.
Limitations inherent in this study are those similar to other in vivo studies. To begin with, the results of injections into rat tendons may not be translatable to human tendons. Despite this limitation, the rat is a common model for tendon research.31 Another limitation is that this study injected healthy Achilles tendons, rather than tendons with preexisting tendinopathy. In a naturally occurring tendinopathy, there may be other factors present that interact with PRP, and this model negates the contribution of these factors. Finally, while the immunohistochemistry (IHC) and morphological data are clear, the cellularity data are not clear in identifying the type of cells that were increased by granulocyte injection. However, the cells appeared rounded, resembling inflammatory infiltrate; a common cell type seen in tendons.2 While fibroblasts are also a common infiltrate during chronic tendinopathy, they are generally flat and appear on H&E as long spindle shaped cells. Thus, we believe the increased cellularity of the tendons after granulocyte injections is representative of an increase in inflammation. The increased cellularity could be due to the increased number of cells injected into the tendon; however, our conclusions are consistent with the increased inflammation previously reported linking leukocytes to tendon inflammation.20,22,32
In terms of morphology, we hypothesized that degenerative changes would be seen in the tendons that were injected with granulocytes due to the inflammatory action of these cells. As part of the granulocyte response, neutrophils release proteases and macrophages can stimulate collagen synthesis via fibroblasts, both causing change within the extracellular matrix.33,34 Indeed, we observed a significant change in tissue morphology in the granulocyte group over the course of 14 days. As the degenerative and regenerative effects of granulocytes take time to present, this is likely what we observed to occur between day 7 and 14 after treatment. These observations are also consistent with prior observations that leukocyte-rich PRP injections can be detrimental to tendon healing, but beneficial to tissue degeneration in the setting of chronic tendonitis.20
We hypothesized that the vascularity of the tendons would be similar in all preparations. This was based on previous studies demonstrating that the lack of platelets in the platelet-poor plasma fraction is sufficient to deplete VEGF, the angiogenic agent in PRP.35 In this study, there were no observable differences in vascularity of platelet-poor plasma, monocyte, and granulocyte injections. We attribute this to the lack of VEGF in any of these preparations. The aforementioned study also showed that the lack of platelets in injection was enough to prevent the angiogenic effect of this treatment.35
Continue to: The goal of this study was...
The goal of this study was to assess the morphology, cellularity, and vascularity of normal tendons after injections of different leukocyte populations. This is clinically important because of the potential to tailor future PRP injections on a patient-by-patient basis. In patients requiring an anabolic response, leukocyte-poor PRP may be the best option. In contrast, when patient pathology requires an inflammatory response to improve healing36 or breakdown fibrotic tissue, as seen in tendinosis, leukocyte-rich PRP may be warranted. Further, properly controlled clinical studies are needed to validate these recommendations.
Limitations inherent in this study are those similar to other in vivo studies. First, the results of injections into rat tendons may not be translatable to human tendons. Despite this limitation, the rat is a common model for tendon research.31 A second limitation is that this study injected healthy Achilles tendons, rather than tendons with preexisting tendinopathy. In a naturally occurring tendinopathy, there may be other factors present that interact with PRP, and this model negates the contribution of these factors. Finally, while the IHC and morphological data show clear changes, the cellularity data are not clear in identifying the type of cells that were increased by granulocyte injection. However, the cells appeared rounded, resembling inflammatory infiltrate; a common cell type seen in tendons.2 While fibroblasts are also a common infiltrate during chronic tendinopathy, they are generally flat and appear on H&E as long spindle shaped cells. The last limitation of this study is the lack of functional mechanical testing since, clinically, healing of the tendon is also related to the strength of the tendon. Thus, we believe the increased cellularity of the tendons after granulocyte injections is representative of an increase in inflammation. Moreover, our results are consistent with the increased inflammation previously reported linking leukocytes to tendon inflammation.20,22,32 It is interesting to note that the increase in inflammation does not lead to an increase in vascularity as could be expected.
CONCLUSION
We found that the injection of leukocytes into healthy rat Achilles tendons increases inflammation, as evidenced by increased cellularity and disrupted morphology, which suggests that leukocyte-rich PRP preparations may be contraindicated in settings of acute tendonitis. However, these preparations may be useful for a specific subset of tendinopathies, including chronic tendinosis.
1. Herring SA, Nilson KL. Introduction to overuse injuries. Clin Sports Med. 1987;6(2):225-239.
2. Bass E. Tendinopathy: why the difference between tendinitis and tendinosis matters. Int J Ther Massage Bodywork. 2012;5(1):14-17.
3. James SL, Bates BT, Osternig LR. Injuries to runners. Am J Sports Med. 1978;6(2):40-50.
4. Allander E. Prevalence, incidence, and remission rates of some common rheumatic diseases or syndromes. Scand J Rheumatol. 1974;3(3):145-153.
5. Bahr R. No injuries, but plenty of pain? On the methodology for recording overuse symptoms in sports. Br J Sports Med. 2009;43(13):966-972.
6. Rees JD, Maffulli N, Cook J. Management of tendinopathy. Am J Sports Med. 2009;37(9):1855-1867.
7. Andres BM, Murrell GA. Treatment of tendinopathy: what works, what does not, and what is on the horizon. Clin Orthop Relat Res. 2008;466(7):1539-1554.
8. Hall MP, Band PA, Meislin RJ, Jazrawi LM, Cardone DA. Platelet-rich plasma: current concepts and application in sports medicine. J Am Acad Orthop Surg. 2009;17(10):602-608.
9. Smith JW. Blood Supply of Tendons. Am J Surg. 1965;109:272-276.
10. Wu PI, Diaz R, Borg-Stein J. Platelet-rich plasma. Phys Med Rehabil Clin N Am. 2016;27(4):825-853.
11. Nguyen RT, Borg-Stein J, McInnis K. Applications of platelet-rich plasma in musculoskeletal and sports medicine: an evidence-based approach. PM R. 2011;3(3):226-250.
12. Broughton G 2nd, Janis JE, Attinger CE. Wound healing: an overview. Plast Reconstr Surg. 2006;117(7 Suppl):1e-S-32e-S.
13. Mazzocca AD, McCarthy MB, Chowaniec DM, et al. Platelet-rich plasma differs according to preparation method and human variability. J Bone Joint Surg Am. 2012;94(4):308-316.
14. Mazzocca AD, McCarthy MB, Chowaniec DM, et al. The positive effects of different platelet-rich plasma methods on human muscle, bone, and tendon cells. Am J Sports Med. 2012;40(8):1742-1749.
15. Castillo TN, Pouliot MA, Kim HJ, Dragoo JL. Comparison of growth factor and platelet concentration from commercial platelet-rich plasma separation systems. Am J Sports Med. 2011;39(2):266-271.
16. Cho HS, Song IH, Park SY, Sung MC, Ahn MW, Song KE. Individual variation in growth factor concentrations in platelet-rich plasma and its influence on human mesenchymal stem cells. Korean J Lab Med. 2011;31(3):212-218.
17. Weibrich G, Kleis WK, Hafner G, Hitzler WE. Growth factor levels in platelet-rich plasma and correlations with donor age, sex, and platelet count. J Craniomaxillofac Surg. 2002;30(2):97-102.
18. Taylor DW, Petrera M, Hendry M, Theodoropoulos JS. A systematic review of the use of platelet-rich plasma in sports medicine as a new treatment for tendon and ligament injuries. Clin J Sport Med. 2011;21(4):344-352.
19. McCarrel T, Fortier L. Temporal growth factor release from platelet-rich plasma, trehalose lyophilized platelets, and bone marrow aspirate and their effect on tendon and ligament gene expression. J Orthop Res. 2009;27(8):1033-1042.
20. McCarrel TM, Minas T, Fortier LA. Optimization of leukocyte concentration in platelet-rich plasma for the treatment of tendinopathy. J Bone Joint Surg Am. 2012;94(19):e143(141-148).
21. Pillitteri D, Bassus S, Boller K, et al. Thrombin-induced interleukin 1beta synthesis in platelet suspensions: impact of contaminating leukocytes. Platelets. 2007;18(2):119-127.
22. Boswell SG, Schnabel LV, Mohammed HO, Sundman EA, Minas T, Fortier LA. Increasing platelet concentrations in leukocyte-reduced platelet-rich plasma decrease collagen gene synthesis in tendons. Am J Sports Med. 2014;42(1):42-49.
23. Mishra A, Pavelko T. Treatment of chronic elbow tendinosis with buffered platelet-rich plasma. Am J Sports Med. 2006;34(11):1774-1778.
24. Maniscalco P, Gambera D, Lunati A, et al. The "Cascade" membrane: a new PRP device for tendon ruptures. Description and case report on rotator cuff tendon. Acta Biomed. 2008;79(3):223-226.
25. Filardo G, Kon E, Della Villa S, Vincentelli F, Fornasari PM, Marcacci M. Use of platelet-rich plasma for the treatment of refractory jumper's knee. Int Orthop. 2010;34(6):909-915.
26. Peerbooms JC, Sluimer J, Bruijn DJ, Gosens T. Positive effect of an autologous platelet concentrate in lateral epicondylitis in a double-blind randomized controlled trial: platelet-rich plasma versus corticosteroid injection with a 1-year follow-up. Am J Sports Med. 2010;38(2):255-262.
27. de Vos RJ, Weir A, van Schie HT, et al. Platelet-rich plasma injection for chronic Achilles tendinopathy: a randomized controlled trial. JAMA. 2010;303(2):144-149.
28. Schepull T, Kvist J, Norrman H, Trinks M, Berlin G, Aspenberg P. Autologous platelets have no effect on the healing of human achilles tendon ruptures: a randomized single-blind study. Am J Sports Med. 2011;39(1):38-47.
29. Welsh KI, Burgos H, Batchelor JR. The immune response to allogeneic rat platelets; Ag-B antigens in matrix form lacking Ia. Eur J Immunol. 1977;7(5):267-272.
30. Xue M, Del Bigio MR. Intracortical hemorrhage injury in rats : relationship between blood fractions and brain cell death. Stroke. 2000;31(7):1721-1727.
31. Voleti PB, Buckley MR, Soslowsky LJ. Tendon healing: repair and regeneration. Annu Rev Biomed Eng. 2012;14:47-71.
32. Sundman EA, Cole BJ, Fortier LA. Growth factor and catabolic cytokine concentrations are influenced by the cellular composition of platelet-rich plasma. Am J Sports Med. 2011;39(10):2135-2140.
33. Palmgren MS, deShazo RD, Carter RM, Zimny ML, Shah SV. Mechanisms of neutrophil damage to human alveolar extracellular matrix: the role of serine and metalloproteases. J Allergy Clin Immunol. 1992;89(4):905-915.
34. Khalil N, Bereznay O, Sporn M, Greenberg AH. Macrophage production of transforming growth factor beta and fibroblast collagen synthesis in chronic pulmonary inflammation. J Exp Med. 1989;170(3):727-737.
35. Zhou Y, Zhang J, Wu H, Hogan MV, Wang JH. The differential effects of leukocyte-containing and pure platelet-rich plasma (PRP) on tendon stem/progenitor cells - implications of PRP application for the clinical treatment of tendon injuries. Stem Cell Res Ther. 2015;6:173.
36. Su B, O'Connor JP. NSAID therapy effects on healing of bone, tendon, and the enthesis. J Appl Physiol (1985). 2013;115(6):892-899.
ABSTRACT
In this study, we determine the in vivo effects of injecting sub-populations of leukocytes into normal rat Achilles tendons via a controlled laboratory study. Allogenic monocytes, granulocytes, or plasma were injected into 24 healthy rat Achilles tendons. Treated and contralateral un-treated control tendons then assessed for cellularity, histologic morphology, and vascularity after 7 and 14 days. Significant increases of 221% and 249% in cellularity (P = 0.014) were seen on day 14 within Achilles tendons injected with granulocytes as compared to plasma and monocytes, respectively. Also, significant improvement in morphology (P = 0.029) between days 7 and 14 was seen for the granulocyte injected Achilles tendons. Significant increases in cellularity after an injection of granulocytes, compared to monocytes and plasma, corresponds to a significant increase in inflammation within the tissue, suggesting that leukocyte-rich platelet-rich plasma (PRP) preparations are proinflammatory and potentially catabolic when injected into tendon tissue. The concentration and composition of white blood cells within PRP preparations is variable and needs to be better understood in order to optimize clinical utility of PRP injections.
Continue to: Tendinopathies are debilitating conditions...
Tendinopathies are debilitating conditions affecting patients worldwide every day. They arise most frequently from tendon overuse resulting in pathology.1 There are 2 major subtypes of tendinopathy: tendinosis and tendinitis. Tendinosis, the more common condition, is characterized by long-term, chronic degradation of tendon tissue resulting in fibrosis from infiltrating fibroblasts.2 Tendinitis, the less common condition, is characterized by an acute inflammatory response and inflammatory cell infiltrate.2 Both conditions are common, with Achilles tendinopathy affecting 11% of runners and lateral epicondylitis affecting 1% to 3% of the general population.3,4 Many sports-related overuse injuries, such as tendinopathies, go undiagnosed for extended periods of time because medical attention is avoided in order to prevent time loss from training or competing.5 These delays could be eliminated if a non-surgical option for treating tendon pathology was available.
Tendinopathies are believed to result from tendon overuse that causes micro-damage to collagen, as well as from significant changes in protein and enzyme composition within the tendon.6 The damage accumulates over time and eventually leads to chronic inflammation or fibrotic change within tendons, in both cases weakening the tendon and causing pain. Currently, accepted treatments for tendinopathies include: nonsteroidal anti-inflammatory drugs, physical therapy, ultrasound, laser-therapy, corticosteroids, glyceryl trinitrate patches, extracorporeal shock wave therapy, sclerotherapy, and surgery.7 Recently, platelet-rich plasma (PRP) therapy has emerged as a promising treatment for tendinopathies, as well as a variety of other orthopedic indications.
PRP consists of autologous blood from the patient, centrifuged to increase the amount of platelets in the sample above baseline, and subsequently injected around an affected tendon or joint.8 PRP is used to treat tendinopathy because it can supply injured tendons with blood components that aid in healing, which tendons do not receive due to poor vascularity.9 These components include growth factors, such as platelet derived growth factor (PDGF), transforming growth factor-β (TGF-β), vascular endothelial growth factor (VEGF), endothelial growth factor, and leukocytes that can stimulate an inflammatory response within the injured tissue.10 The inflammatory response from the PRP induces a more robust reconstruction and revascularization of the injured tissue, stimulating proliferation, and remodeling.11,12However, significant variability exists within the platelets, leukocytes, and growth factors that comprise PRP. This is attributed to 3 major causes. First, current commercial preparations of PRP result in differing platelet concentrations, as well as leukocyte-rich and leukocyte-poor compositions.13,14 Variability in platelet concentrations results in unreliable amounts of growth factors, including cytokines, TGF-β, PDGF, VEGF and basic fibroblast growth factor in each preparation, while leukocyte levels affect inflammation, all leading to variable effects for each preparation.15,16Second, despite sex and age of the PRP donor not being significant factors influencing variation in growth factor concentrations, the existence of an unexplained variation in concentrations of growth factors between different donors has been observed.17 Third, the selection of activating agents, bovine thrombin or calcium chloride, and their application, whether to the elbow, shoulder, or knee, produces variability.18
While the effects of platelets and growth factors in PRP have been well studied, less is known about the effects of differing cell types. Recently it was reported that the concentrations of leukocytes directly affect the outcomes of PRP injections. McCarrel and colleagues19,20 found that as the number of leukocytes increased, there was a concomitant increase in the expression of inflammatory cytokines and catabolic activity. This effect may result in inferior healing of injured tissues and is attributed to the release of pro-inflammatory cytokines such as interleukin-1β from the leukocytes.21 There is also evidence that minimizing the catabolic effect of leukocytes may be just as important to tissue healing as the maximizing anabolic effect of platelets and growth factors.22
The use of PRP has been highly disputed in recent years due to conflicting reports of its success in treating orthopedic conditions. Numerous favorable studies have shown benefit for treating chronic and acute orthopedic injuries including; rotator cuff tear repair, chronic refractory patellar tendinopathy, and chronic lateral tendinosis/epicondylitis.23-26 Concurrently, articles demonstrating no significant effects from PRP have also been published. One study claiming that PRP injections did not improve outcomes of chronic Achilles tendinopathy did not differentiate whether patients had tendinosis or tendinitis, and did not consider leukocyte concentration in their PRP preparations27 Another study that determined PRP is not beneficial to the healing of ruptured Achilles tendons after surgical repair also failed to consider the concentration of leukocytes in their PRP preparations.28 One of the difficulties in comparing these studies is their heterogeneous nature. This arises from the use of different conditions in each study that makes the studies incomparable. Variations in PRP preparations lead to different concentrations of growth factors, platelets, and leukocyte concentrations. Additionally, tendinopathy models were not specified as tendinosis and tendonitis, and models or patients were not controlled for age, sex, or comorbidities. Given that leukocyte-rich and leukocyte-poor PRP preparations are currently widely used in clinical practice, the discovery of which type of preparation is indicated in which setting is paramount to evidence-based use of this treatment modality. Due to reports suggesting that leukocytes may be detrimental to tendon healing, determining which types of leukocytes are responsible for these effects is vital. As such, the purpose of this study is to determine the in vivo effects of sub-populations of leukocytes on normal rat tendons. This study design allowed us to isolate the effects of the injections to induce a response and remove confounding effects of normal healing response to a damaged tendon and effects from the injection itself. Our hypothesis was that the injection of leukocytes would cause an inflammatory response in rat tendons, leading to catabolic outcomes.
Continue to: METHODS...
METHODS
This was a prospective, in vivo, placebo controlled, randomized animal study. The University’s Institutional Animal Care and Use Committee approved all procedures prior to initiation. Twenty-four male Sprague-Dawley rats were randomized to 3 treatment groups (n = 8): monocytes; granulocytes, and; plasma, as a negative control.
Allogenic blood from 6 additional rats was collected into K2EDTA tubes via cardiac puncture. Allogenic, as opposed to autogenic, blood is commonly used in rat models because of low immunogenic response to blood from rats of the same strain and litter.29,30 The blood was then pooled and the red cells lysed by incubation with Red Blood Cell Lysis Buffer (Roche). The samples were then sorted into fractions containing monocytes and granulocytes using fluorescence activated cell sorting (FACS) using a FACSAria (BD Biosciences). Cells were sorted using Purified PE Mouse Anti-Rat CD11b/c antibodies (BD Pharmingen) specific to monocytes, granulocytes, macrophages, dendritic cells, and microglia, APC-Cy7 Mouse Anti-Rat CD45 antibodies (BD Pharmingen) specific to all hematopoietic cells except erythrocytes, and FITC Mouse Anti-Rat CD42d antibodies (BD Pharmingen) specific to megakaryocytes and platelets. 20 μL of 0.2 mg/mL CD11b/c, 20 μL of 0.2 mg/mL CD 45, and 10 μL of 0.5 mg/mL CD42d antibodies were added to 1 mL of condensed non-red cells collected from the 6 rats and incubated at room temperature in the dark for 15 minutes. A fraction containing only platelet-poor plasma was also collected. For all treatments the injection volume was 75 μL. Rats in the monocyte group were injected with 200,000 cells in platelet-poor plasma, those in the granulocyte group were injected with 900,000 cells in platelet-poor plasma, and rats in the plasma control group received only platelet-poor plasma. The cell concentrations were based on previous studies that documented these concentrations that are found in typical leukocyte-rich PRP preparations.13
The animals were anesthetized with isoflurane gas and then injected aseptically once into their right Achilles tendon. The left Achilles tendon was used as an un-injected control, giving a total of 48 total Achilles tendons studied. At days 7 and 14 post-injection, 4 rats from each group were euthanized and the Achilles tendons were harvested.
The tendons were fixed in neutral buffered formalin for 24 hours and then embedded in paraffin and sectioned sagittally at 12 μm. The tendons were then stained with hematoxylin and eosin (H&E) using standard histological protocols and examined by 3 individuals trained to assess cellularity and morphology. All samples were assigned unrecognizable numbers and randomized prior to examination by individuals. Cell counts were based on the number of nuclei present in 3 mid-tendon high-power fields (400x) per sample. Morphology was graded on a scale of 1 to 3, with 1 being a normal tendon and 3 having severe pathology with total loss of alignment and crimping on 3 low-power fields (100x) per sample (Figures 1A-1G).
Vascularity was assessed by immunohistochemical staining using Rabbit Polyclonal Anti-CD31 antibodies (Abcam), a marker for vascular endothelial cells, using a Vectastain ABC Kit (Vector Laboratories) system and the ImmPACT AEC Peroxidase (HRP) Substrate (Vector Laboratories). Following staining, automated image analysis was performed (Bioquant). Briefly, all areas that did not contain tendon were masked. CD31 positive areas were then quantified using global thresholding. Vascularity was then calculated as ratio of CD31 positive area to total tendon area. Analyses were performed on 3 mid-tendon medium-power (200x) fields per sample.
For cellularity and morphology, the results for the injected tendons were normalized to those of their contralateral untreated controls and reported as a percentage. Results for vascularity were compared directly between treated tendons. Differences were assessed between groups at each time-point using Independent Samples Median Tests. When significant differences were identified, pairwise comparisons were performed to identify the source of the differences. All analyses were conducted using SPSS (V22, SAS Institute) with significant differences determined for values of P < 0.05.
RESULTS
No significant differences in cellularity between groups were seen at day 7 (P = 0.368) (Figures 1A-1G). However, a significant difference in cellularity between groups was seen at day 14 (P = 0.014). Pairwise tests showed there to be a significant increase in the number of cells in the tendons treated with granulocytes from 221% and 249% in cellularity (P = 0.014) on day 14, as compared to both monocytes and plasma, respectively. Morphologically, no significant differences were seen between groups at either time-point (P = 0.091 for day 7 and P = 1.000 for day 14) (Figures 2A-2G). However, a significant improvement in morphology was observed from day 7 to day 14 in the granulocyte group from 60% to 165% (P = 0.029). Finally, no differences were seen in vascularity between treatment groups at either time-point (P = 0.368 for day 7 and P = 0.535 for day 14) (Figures 3A-3G).
Continue to: DISCUSSION...
DISCUSSION
Our hypothesis that the injection of leukocytes would cause an inflammatory response in rat tendons leading to catabolic outcomes was confirmed in the granulocyte group. It should be noted that prior to the catabolic outcome, there was a transient anabolic effect in the granulocyte group during the second week. Deterioration in morphology was observed in the tendons injected with granulocytes on day 7, which subsequently recovered in the following week. We found that injecting granulocytes into normal tendons resulted in an increase in inflammatory cellularity, when compared to monocytes and plasma injections.
Limitations inherent in this study are those similar to other in vivo studies. To begin with, the results of injections into rat tendons may not be translatable to human tendons. Despite this limitation, the rat is a common model for tendon research.31 Another limitation is that this study injected healthy Achilles tendons, rather than tendons with preexisting tendinopathy. In a naturally occurring tendinopathy, there may be other factors present that interact with PRP, and this model negates the contribution of these factors. Finally, while the immunohistochemistry (IHC) and morphological data are clear, the cellularity data are not clear in identifying the type of cells that were increased by granulocyte injection. However, the cells appeared rounded, resembling inflammatory infiltrate; a common cell type seen in tendons.2 While fibroblasts are also a common infiltrate during chronic tendinopathy, they are generally flat and appear on H&E as long spindle shaped cells. Thus, we believe the increased cellularity of the tendons after granulocyte injections is representative of an increase in inflammation. The increased cellularity could be due to the increased number of cells injected into the tendon; however, our conclusions are consistent with the increased inflammation previously reported linking leukocytes to tendon inflammation.20,22,32
In terms of morphology, we hypothesized that degenerative changes would be seen in the tendons that were injected with granulocytes due to the inflammatory action of these cells. As part of the granulocyte response, neutrophils release proteases and macrophages can stimulate collagen synthesis via fibroblasts, both causing change within the extracellular matrix.33,34 Indeed, we observed a significant change in tissue morphology in the granulocyte group over the course of 14 days. As the degenerative and regenerative effects of granulocytes take time to present, this is likely what we observed to occur between day 7 and 14 after treatment. These observations are also consistent with prior observations that leukocyte-rich PRP injections can be detrimental to tendon healing, but beneficial to tissue degeneration in the setting of chronic tendonitis.20
We hypothesized that the vascularity of the tendons would be similar in all preparations. This was based on previous studies demonstrating that the lack of platelets in the platelet-poor plasma fraction is sufficient to deplete VEGF, the angiogenic agent in PRP.35 In this study, there were no observable differences in vascularity of platelet-poor plasma, monocyte, and granulocyte injections. We attribute this to the lack of VEGF in any of these preparations. The aforementioned study also showed that the lack of platelets in injection was enough to prevent the angiogenic effect of this treatment.35
Continue to: The goal of this study was...
The goal of this study was to assess the morphology, cellularity, and vascularity of normal tendons after injections of different leukocyte populations. This is clinically important because of the potential to tailor future PRP injections on a patient-by-patient basis. In patients requiring an anabolic response, leukocyte-poor PRP may be the best option. In contrast, when patient pathology requires an inflammatory response to improve healing36 or breakdown fibrotic tissue, as seen in tendinosis, leukocyte-rich PRP may be warranted. Further, properly controlled clinical studies are needed to validate these recommendations.
Limitations inherent in this study are those similar to other in vivo studies. First, the results of injections into rat tendons may not be translatable to human tendons. Despite this limitation, the rat is a common model for tendon research.31 A second limitation is that this study injected healthy Achilles tendons, rather than tendons with preexisting tendinopathy. In a naturally occurring tendinopathy, there may be other factors present that interact with PRP, and this model negates the contribution of these factors. Finally, while the IHC and morphological data show clear changes, the cellularity data are not clear in identifying the type of cells that were increased by granulocyte injection. However, the cells appeared rounded, resembling inflammatory infiltrate; a common cell type seen in tendons.2 While fibroblasts are also a common infiltrate during chronic tendinopathy, they are generally flat and appear on H&E as long spindle shaped cells. The last limitation of this study is the lack of functional mechanical testing since, clinically, healing of the tendon is also related to the strength of the tendon. Thus, we believe the increased cellularity of the tendons after granulocyte injections is representative of an increase in inflammation. Moreover, our results are consistent with the increased inflammation previously reported linking leukocytes to tendon inflammation.20,22,32 It is interesting to note that the increase in inflammation does not lead to an increase in vascularity as could be expected.
CONCLUSION
We found that the injection of leukocytes into healthy rat Achilles tendons increases inflammation, as evidenced by increased cellularity and disrupted morphology, which suggests that leukocyte-rich PRP preparations may be contraindicated in settings of acute tendonitis. However, these preparations may be useful for a specific subset of tendinopathies, including chronic tendinosis.
ABSTRACT
In this study, we determine the in vivo effects of injecting sub-populations of leukocytes into normal rat Achilles tendons via a controlled laboratory study. Allogenic monocytes, granulocytes, or plasma were injected into 24 healthy rat Achilles tendons. Treated and contralateral un-treated control tendons then assessed for cellularity, histologic morphology, and vascularity after 7 and 14 days. Significant increases of 221% and 249% in cellularity (P = 0.014) were seen on day 14 within Achilles tendons injected with granulocytes as compared to plasma and monocytes, respectively. Also, significant improvement in morphology (P = 0.029) between days 7 and 14 was seen for the granulocyte injected Achilles tendons. Significant increases in cellularity after an injection of granulocytes, compared to monocytes and plasma, corresponds to a significant increase in inflammation within the tissue, suggesting that leukocyte-rich platelet-rich plasma (PRP) preparations are proinflammatory and potentially catabolic when injected into tendon tissue. The concentration and composition of white blood cells within PRP preparations is variable and needs to be better understood in order to optimize clinical utility of PRP injections.
Continue to: Tendinopathies are debilitating conditions...
Tendinopathies are debilitating conditions affecting patients worldwide every day. They arise most frequently from tendon overuse resulting in pathology.1 There are 2 major subtypes of tendinopathy: tendinosis and tendinitis. Tendinosis, the more common condition, is characterized by long-term, chronic degradation of tendon tissue resulting in fibrosis from infiltrating fibroblasts.2 Tendinitis, the less common condition, is characterized by an acute inflammatory response and inflammatory cell infiltrate.2 Both conditions are common, with Achilles tendinopathy affecting 11% of runners and lateral epicondylitis affecting 1% to 3% of the general population.3,4 Many sports-related overuse injuries, such as tendinopathies, go undiagnosed for extended periods of time because medical attention is avoided in order to prevent time loss from training or competing.5 These delays could be eliminated if a non-surgical option for treating tendon pathology was available.
Tendinopathies are believed to result from tendon overuse that causes micro-damage to collagen, as well as from significant changes in protein and enzyme composition within the tendon.6 The damage accumulates over time and eventually leads to chronic inflammation or fibrotic change within tendons, in both cases weakening the tendon and causing pain. Currently, accepted treatments for tendinopathies include: nonsteroidal anti-inflammatory drugs, physical therapy, ultrasound, laser-therapy, corticosteroids, glyceryl trinitrate patches, extracorporeal shock wave therapy, sclerotherapy, and surgery.7 Recently, platelet-rich plasma (PRP) therapy has emerged as a promising treatment for tendinopathies, as well as a variety of other orthopedic indications.
PRP consists of autologous blood from the patient, centrifuged to increase the amount of platelets in the sample above baseline, and subsequently injected around an affected tendon or joint.8 PRP is used to treat tendinopathy because it can supply injured tendons with blood components that aid in healing, which tendons do not receive due to poor vascularity.9 These components include growth factors, such as platelet derived growth factor (PDGF), transforming growth factor-β (TGF-β), vascular endothelial growth factor (VEGF), endothelial growth factor, and leukocytes that can stimulate an inflammatory response within the injured tissue.10 The inflammatory response from the PRP induces a more robust reconstruction and revascularization of the injured tissue, stimulating proliferation, and remodeling.11,12However, significant variability exists within the platelets, leukocytes, and growth factors that comprise PRP. This is attributed to 3 major causes. First, current commercial preparations of PRP result in differing platelet concentrations, as well as leukocyte-rich and leukocyte-poor compositions.13,14 Variability in platelet concentrations results in unreliable amounts of growth factors, including cytokines, TGF-β, PDGF, VEGF and basic fibroblast growth factor in each preparation, while leukocyte levels affect inflammation, all leading to variable effects for each preparation.15,16Second, despite sex and age of the PRP donor not being significant factors influencing variation in growth factor concentrations, the existence of an unexplained variation in concentrations of growth factors between different donors has been observed.17 Third, the selection of activating agents, bovine thrombin or calcium chloride, and their application, whether to the elbow, shoulder, or knee, produces variability.18
While the effects of platelets and growth factors in PRP have been well studied, less is known about the effects of differing cell types. Recently it was reported that the concentrations of leukocytes directly affect the outcomes of PRP injections. McCarrel and colleagues19,20 found that as the number of leukocytes increased, there was a concomitant increase in the expression of inflammatory cytokines and catabolic activity. This effect may result in inferior healing of injured tissues and is attributed to the release of pro-inflammatory cytokines such as interleukin-1β from the leukocytes.21 There is also evidence that minimizing the catabolic effect of leukocytes may be just as important to tissue healing as the maximizing anabolic effect of platelets and growth factors.22
The use of PRP has been highly disputed in recent years due to conflicting reports of its success in treating orthopedic conditions. Numerous favorable studies have shown benefit for treating chronic and acute orthopedic injuries including; rotator cuff tear repair, chronic refractory patellar tendinopathy, and chronic lateral tendinosis/epicondylitis.23-26 Concurrently, articles demonstrating no significant effects from PRP have also been published. One study claiming that PRP injections did not improve outcomes of chronic Achilles tendinopathy did not differentiate whether patients had tendinosis or tendinitis, and did not consider leukocyte concentration in their PRP preparations27 Another study that determined PRP is not beneficial to the healing of ruptured Achilles tendons after surgical repair also failed to consider the concentration of leukocytes in their PRP preparations.28 One of the difficulties in comparing these studies is their heterogeneous nature. This arises from the use of different conditions in each study that makes the studies incomparable. Variations in PRP preparations lead to different concentrations of growth factors, platelets, and leukocyte concentrations. Additionally, tendinopathy models were not specified as tendinosis and tendonitis, and models or patients were not controlled for age, sex, or comorbidities. Given that leukocyte-rich and leukocyte-poor PRP preparations are currently widely used in clinical practice, the discovery of which type of preparation is indicated in which setting is paramount to evidence-based use of this treatment modality. Due to reports suggesting that leukocytes may be detrimental to tendon healing, determining which types of leukocytes are responsible for these effects is vital. As such, the purpose of this study is to determine the in vivo effects of sub-populations of leukocytes on normal rat tendons. This study design allowed us to isolate the effects of the injections to induce a response and remove confounding effects of normal healing response to a damaged tendon and effects from the injection itself. Our hypothesis was that the injection of leukocytes would cause an inflammatory response in rat tendons, leading to catabolic outcomes.
Continue to: METHODS...
METHODS
This was a prospective, in vivo, placebo controlled, randomized animal study. The University’s Institutional Animal Care and Use Committee approved all procedures prior to initiation. Twenty-four male Sprague-Dawley rats were randomized to 3 treatment groups (n = 8): monocytes; granulocytes, and; plasma, as a negative control.
Allogenic blood from 6 additional rats was collected into K2EDTA tubes via cardiac puncture. Allogenic, as opposed to autogenic, blood is commonly used in rat models because of low immunogenic response to blood from rats of the same strain and litter.29,30 The blood was then pooled and the red cells lysed by incubation with Red Blood Cell Lysis Buffer (Roche). The samples were then sorted into fractions containing monocytes and granulocytes using fluorescence activated cell sorting (FACS) using a FACSAria (BD Biosciences). Cells were sorted using Purified PE Mouse Anti-Rat CD11b/c antibodies (BD Pharmingen) specific to monocytes, granulocytes, macrophages, dendritic cells, and microglia, APC-Cy7 Mouse Anti-Rat CD45 antibodies (BD Pharmingen) specific to all hematopoietic cells except erythrocytes, and FITC Mouse Anti-Rat CD42d antibodies (BD Pharmingen) specific to megakaryocytes and platelets. 20 μL of 0.2 mg/mL CD11b/c, 20 μL of 0.2 mg/mL CD 45, and 10 μL of 0.5 mg/mL CD42d antibodies were added to 1 mL of condensed non-red cells collected from the 6 rats and incubated at room temperature in the dark for 15 minutes. A fraction containing only platelet-poor plasma was also collected. For all treatments the injection volume was 75 μL. Rats in the monocyte group were injected with 200,000 cells in platelet-poor plasma, those in the granulocyte group were injected with 900,000 cells in platelet-poor plasma, and rats in the plasma control group received only platelet-poor plasma. The cell concentrations were based on previous studies that documented these concentrations that are found in typical leukocyte-rich PRP preparations.13
The animals were anesthetized with isoflurane gas and then injected aseptically once into their right Achilles tendon. The left Achilles tendon was used as an un-injected control, giving a total of 48 total Achilles tendons studied. At days 7 and 14 post-injection, 4 rats from each group were euthanized and the Achilles tendons were harvested.
The tendons were fixed in neutral buffered formalin for 24 hours and then embedded in paraffin and sectioned sagittally at 12 μm. The tendons were then stained with hematoxylin and eosin (H&E) using standard histological protocols and examined by 3 individuals trained to assess cellularity and morphology. All samples were assigned unrecognizable numbers and randomized prior to examination by individuals. Cell counts were based on the number of nuclei present in 3 mid-tendon high-power fields (400x) per sample. Morphology was graded on a scale of 1 to 3, with 1 being a normal tendon and 3 having severe pathology with total loss of alignment and crimping on 3 low-power fields (100x) per sample (Figures 1A-1G).
Vascularity was assessed by immunohistochemical staining using Rabbit Polyclonal Anti-CD31 antibodies (Abcam), a marker for vascular endothelial cells, using a Vectastain ABC Kit (Vector Laboratories) system and the ImmPACT AEC Peroxidase (HRP) Substrate (Vector Laboratories). Following staining, automated image analysis was performed (Bioquant). Briefly, all areas that did not contain tendon were masked. CD31 positive areas were then quantified using global thresholding. Vascularity was then calculated as ratio of CD31 positive area to total tendon area. Analyses were performed on 3 mid-tendon medium-power (200x) fields per sample.
For cellularity and morphology, the results for the injected tendons were normalized to those of their contralateral untreated controls and reported as a percentage. Results for vascularity were compared directly between treated tendons. Differences were assessed between groups at each time-point using Independent Samples Median Tests. When significant differences were identified, pairwise comparisons were performed to identify the source of the differences. All analyses were conducted using SPSS (V22, SAS Institute) with significant differences determined for values of P < 0.05.
RESULTS
No significant differences in cellularity between groups were seen at day 7 (P = 0.368) (Figures 1A-1G). However, a significant difference in cellularity between groups was seen at day 14 (P = 0.014). Pairwise tests showed there to be a significant increase in the number of cells in the tendons treated with granulocytes from 221% and 249% in cellularity (P = 0.014) on day 14, as compared to both monocytes and plasma, respectively. Morphologically, no significant differences were seen between groups at either time-point (P = 0.091 for day 7 and P = 1.000 for day 14) (Figures 2A-2G). However, a significant improvement in morphology was observed from day 7 to day 14 in the granulocyte group from 60% to 165% (P = 0.029). Finally, no differences were seen in vascularity between treatment groups at either time-point (P = 0.368 for day 7 and P = 0.535 for day 14) (Figures 3A-3G).
Continue to: DISCUSSION...
DISCUSSION
Our hypothesis that the injection of leukocytes would cause an inflammatory response in rat tendons leading to catabolic outcomes was confirmed in the granulocyte group. It should be noted that prior to the catabolic outcome, there was a transient anabolic effect in the granulocyte group during the second week. Deterioration in morphology was observed in the tendons injected with granulocytes on day 7, which subsequently recovered in the following week. We found that injecting granulocytes into normal tendons resulted in an increase in inflammatory cellularity, when compared to monocytes and plasma injections.
Limitations inherent in this study are those similar to other in vivo studies. To begin with, the results of injections into rat tendons may not be translatable to human tendons. Despite this limitation, the rat is a common model for tendon research.31 Another limitation is that this study injected healthy Achilles tendons, rather than tendons with preexisting tendinopathy. In a naturally occurring tendinopathy, there may be other factors present that interact with PRP, and this model negates the contribution of these factors. Finally, while the immunohistochemistry (IHC) and morphological data are clear, the cellularity data are not clear in identifying the type of cells that were increased by granulocyte injection. However, the cells appeared rounded, resembling inflammatory infiltrate; a common cell type seen in tendons.2 While fibroblasts are also a common infiltrate during chronic tendinopathy, they are generally flat and appear on H&E as long spindle shaped cells. Thus, we believe the increased cellularity of the tendons after granulocyte injections is representative of an increase in inflammation. The increased cellularity could be due to the increased number of cells injected into the tendon; however, our conclusions are consistent with the increased inflammation previously reported linking leukocytes to tendon inflammation.20,22,32
In terms of morphology, we hypothesized that degenerative changes would be seen in the tendons that were injected with granulocytes due to the inflammatory action of these cells. As part of the granulocyte response, neutrophils release proteases and macrophages can stimulate collagen synthesis via fibroblasts, both causing change within the extracellular matrix.33,34 Indeed, we observed a significant change in tissue morphology in the granulocyte group over the course of 14 days. As the degenerative and regenerative effects of granulocytes take time to present, this is likely what we observed to occur between day 7 and 14 after treatment. These observations are also consistent with prior observations that leukocyte-rich PRP injections can be detrimental to tendon healing, but beneficial to tissue degeneration in the setting of chronic tendonitis.20
We hypothesized that the vascularity of the tendons would be similar in all preparations. This was based on previous studies demonstrating that the lack of platelets in the platelet-poor plasma fraction is sufficient to deplete VEGF, the angiogenic agent in PRP.35 In this study, there were no observable differences in vascularity of platelet-poor plasma, monocyte, and granulocyte injections. We attribute this to the lack of VEGF in any of these preparations. The aforementioned study also showed that the lack of platelets in injection was enough to prevent the angiogenic effect of this treatment.35
Continue to: The goal of this study was...
The goal of this study was to assess the morphology, cellularity, and vascularity of normal tendons after injections of different leukocyte populations. This is clinically important because of the potential to tailor future PRP injections on a patient-by-patient basis. In patients requiring an anabolic response, leukocyte-poor PRP may be the best option. In contrast, when patient pathology requires an inflammatory response to improve healing36 or breakdown fibrotic tissue, as seen in tendinosis, leukocyte-rich PRP may be warranted. Further, properly controlled clinical studies are needed to validate these recommendations.
Limitations inherent in this study are those similar to other in vivo studies. First, the results of injections into rat tendons may not be translatable to human tendons. Despite this limitation, the rat is a common model for tendon research.31 A second limitation is that this study injected healthy Achilles tendons, rather than tendons with preexisting tendinopathy. In a naturally occurring tendinopathy, there may be other factors present that interact with PRP, and this model negates the contribution of these factors. Finally, while the IHC and morphological data show clear changes, the cellularity data are not clear in identifying the type of cells that were increased by granulocyte injection. However, the cells appeared rounded, resembling inflammatory infiltrate; a common cell type seen in tendons.2 While fibroblasts are also a common infiltrate during chronic tendinopathy, they are generally flat and appear on H&E as long spindle shaped cells. The last limitation of this study is the lack of functional mechanical testing since, clinically, healing of the tendon is also related to the strength of the tendon. Thus, we believe the increased cellularity of the tendons after granulocyte injections is representative of an increase in inflammation. Moreover, our results are consistent with the increased inflammation previously reported linking leukocytes to tendon inflammation.20,22,32 It is interesting to note that the increase in inflammation does not lead to an increase in vascularity as could be expected.
CONCLUSION
We found that the injection of leukocytes into healthy rat Achilles tendons increases inflammation, as evidenced by increased cellularity and disrupted morphology, which suggests that leukocyte-rich PRP preparations may be contraindicated in settings of acute tendonitis. However, these preparations may be useful for a specific subset of tendinopathies, including chronic tendinosis.
1. Herring SA, Nilson KL. Introduction to overuse injuries. Clin Sports Med. 1987;6(2):225-239.
2. Bass E. Tendinopathy: why the difference between tendinitis and tendinosis matters. Int J Ther Massage Bodywork. 2012;5(1):14-17.
3. James SL, Bates BT, Osternig LR. Injuries to runners. Am J Sports Med. 1978;6(2):40-50.
4. Allander E. Prevalence, incidence, and remission rates of some common rheumatic diseases or syndromes. Scand J Rheumatol. 1974;3(3):145-153.
5. Bahr R. No injuries, but plenty of pain? On the methodology for recording overuse symptoms in sports. Br J Sports Med. 2009;43(13):966-972.
6. Rees JD, Maffulli N, Cook J. Management of tendinopathy. Am J Sports Med. 2009;37(9):1855-1867.
7. Andres BM, Murrell GA. Treatment of tendinopathy: what works, what does not, and what is on the horizon. Clin Orthop Relat Res. 2008;466(7):1539-1554.
8. Hall MP, Band PA, Meislin RJ, Jazrawi LM, Cardone DA. Platelet-rich plasma: current concepts and application in sports medicine. J Am Acad Orthop Surg. 2009;17(10):602-608.
9. Smith JW. Blood Supply of Tendons. Am J Surg. 1965;109:272-276.
10. Wu PI, Diaz R, Borg-Stein J. Platelet-rich plasma. Phys Med Rehabil Clin N Am. 2016;27(4):825-853.
11. Nguyen RT, Borg-Stein J, McInnis K. Applications of platelet-rich plasma in musculoskeletal and sports medicine: an evidence-based approach. PM R. 2011;3(3):226-250.
12. Broughton G 2nd, Janis JE, Attinger CE. Wound healing: an overview. Plast Reconstr Surg. 2006;117(7 Suppl):1e-S-32e-S.
13. Mazzocca AD, McCarthy MB, Chowaniec DM, et al. Platelet-rich plasma differs according to preparation method and human variability. J Bone Joint Surg Am. 2012;94(4):308-316.
14. Mazzocca AD, McCarthy MB, Chowaniec DM, et al. The positive effects of different platelet-rich plasma methods on human muscle, bone, and tendon cells. Am J Sports Med. 2012;40(8):1742-1749.
15. Castillo TN, Pouliot MA, Kim HJ, Dragoo JL. Comparison of growth factor and platelet concentration from commercial platelet-rich plasma separation systems. Am J Sports Med. 2011;39(2):266-271.
16. Cho HS, Song IH, Park SY, Sung MC, Ahn MW, Song KE. Individual variation in growth factor concentrations in platelet-rich plasma and its influence on human mesenchymal stem cells. Korean J Lab Med. 2011;31(3):212-218.
17. Weibrich G, Kleis WK, Hafner G, Hitzler WE. Growth factor levels in platelet-rich plasma and correlations with donor age, sex, and platelet count. J Craniomaxillofac Surg. 2002;30(2):97-102.
18. Taylor DW, Petrera M, Hendry M, Theodoropoulos JS. A systematic review of the use of platelet-rich plasma in sports medicine as a new treatment for tendon and ligament injuries. Clin J Sport Med. 2011;21(4):344-352.
19. McCarrel T, Fortier L. Temporal growth factor release from platelet-rich plasma, trehalose lyophilized platelets, and bone marrow aspirate and their effect on tendon and ligament gene expression. J Orthop Res. 2009;27(8):1033-1042.
20. McCarrel TM, Minas T, Fortier LA. Optimization of leukocyte concentration in platelet-rich plasma for the treatment of tendinopathy. J Bone Joint Surg Am. 2012;94(19):e143(141-148).
21. Pillitteri D, Bassus S, Boller K, et al. Thrombin-induced interleukin 1beta synthesis in platelet suspensions: impact of contaminating leukocytes. Platelets. 2007;18(2):119-127.
22. Boswell SG, Schnabel LV, Mohammed HO, Sundman EA, Minas T, Fortier LA. Increasing platelet concentrations in leukocyte-reduced platelet-rich plasma decrease collagen gene synthesis in tendons. Am J Sports Med. 2014;42(1):42-49.
23. Mishra A, Pavelko T. Treatment of chronic elbow tendinosis with buffered platelet-rich plasma. Am J Sports Med. 2006;34(11):1774-1778.
24. Maniscalco P, Gambera D, Lunati A, et al. The "Cascade" membrane: a new PRP device for tendon ruptures. Description and case report on rotator cuff tendon. Acta Biomed. 2008;79(3):223-226.
25. Filardo G, Kon E, Della Villa S, Vincentelli F, Fornasari PM, Marcacci M. Use of platelet-rich plasma for the treatment of refractory jumper's knee. Int Orthop. 2010;34(6):909-915.
26. Peerbooms JC, Sluimer J, Bruijn DJ, Gosens T. Positive effect of an autologous platelet concentrate in lateral epicondylitis in a double-blind randomized controlled trial: platelet-rich plasma versus corticosteroid injection with a 1-year follow-up. Am J Sports Med. 2010;38(2):255-262.
27. de Vos RJ, Weir A, van Schie HT, et al. Platelet-rich plasma injection for chronic Achilles tendinopathy: a randomized controlled trial. JAMA. 2010;303(2):144-149.
28. Schepull T, Kvist J, Norrman H, Trinks M, Berlin G, Aspenberg P. Autologous platelets have no effect on the healing of human achilles tendon ruptures: a randomized single-blind study. Am J Sports Med. 2011;39(1):38-47.
29. Welsh KI, Burgos H, Batchelor JR. The immune response to allogeneic rat platelets; Ag-B antigens in matrix form lacking Ia. Eur J Immunol. 1977;7(5):267-272.
30. Xue M, Del Bigio MR. Intracortical hemorrhage injury in rats : relationship between blood fractions and brain cell death. Stroke. 2000;31(7):1721-1727.
31. Voleti PB, Buckley MR, Soslowsky LJ. Tendon healing: repair and regeneration. Annu Rev Biomed Eng. 2012;14:47-71.
32. Sundman EA, Cole BJ, Fortier LA. Growth factor and catabolic cytokine concentrations are influenced by the cellular composition of platelet-rich plasma. Am J Sports Med. 2011;39(10):2135-2140.
33. Palmgren MS, deShazo RD, Carter RM, Zimny ML, Shah SV. Mechanisms of neutrophil damage to human alveolar extracellular matrix: the role of serine and metalloproteases. J Allergy Clin Immunol. 1992;89(4):905-915.
34. Khalil N, Bereznay O, Sporn M, Greenberg AH. Macrophage production of transforming growth factor beta and fibroblast collagen synthesis in chronic pulmonary inflammation. J Exp Med. 1989;170(3):727-737.
35. Zhou Y, Zhang J, Wu H, Hogan MV, Wang JH. The differential effects of leukocyte-containing and pure platelet-rich plasma (PRP) on tendon stem/progenitor cells - implications of PRP application for the clinical treatment of tendon injuries. Stem Cell Res Ther. 2015;6:173.
36. Su B, O'Connor JP. NSAID therapy effects on healing of bone, tendon, and the enthesis. J Appl Physiol (1985). 2013;115(6):892-899.
1. Herring SA, Nilson KL. Introduction to overuse injuries. Clin Sports Med. 1987;6(2):225-239.
2. Bass E. Tendinopathy: why the difference between tendinitis and tendinosis matters. Int J Ther Massage Bodywork. 2012;5(1):14-17.
3. James SL, Bates BT, Osternig LR. Injuries to runners. Am J Sports Med. 1978;6(2):40-50.
4. Allander E. Prevalence, incidence, and remission rates of some common rheumatic diseases or syndromes. Scand J Rheumatol. 1974;3(3):145-153.
5. Bahr R. No injuries, but plenty of pain? On the methodology for recording overuse symptoms in sports. Br J Sports Med. 2009;43(13):966-972.
6. Rees JD, Maffulli N, Cook J. Management of tendinopathy. Am J Sports Med. 2009;37(9):1855-1867.
7. Andres BM, Murrell GA. Treatment of tendinopathy: what works, what does not, and what is on the horizon. Clin Orthop Relat Res. 2008;466(7):1539-1554.
8. Hall MP, Band PA, Meislin RJ, Jazrawi LM, Cardone DA. Platelet-rich plasma: current concepts and application in sports medicine. J Am Acad Orthop Surg. 2009;17(10):602-608.
9. Smith JW. Blood Supply of Tendons. Am J Surg. 1965;109:272-276.
10. Wu PI, Diaz R, Borg-Stein J. Platelet-rich plasma. Phys Med Rehabil Clin N Am. 2016;27(4):825-853.
11. Nguyen RT, Borg-Stein J, McInnis K. Applications of platelet-rich plasma in musculoskeletal and sports medicine: an evidence-based approach. PM R. 2011;3(3):226-250.
12. Broughton G 2nd, Janis JE, Attinger CE. Wound healing: an overview. Plast Reconstr Surg. 2006;117(7 Suppl):1e-S-32e-S.
13. Mazzocca AD, McCarthy MB, Chowaniec DM, et al. Platelet-rich plasma differs according to preparation method and human variability. J Bone Joint Surg Am. 2012;94(4):308-316.
14. Mazzocca AD, McCarthy MB, Chowaniec DM, et al. The positive effects of different platelet-rich plasma methods on human muscle, bone, and tendon cells. Am J Sports Med. 2012;40(8):1742-1749.
15. Castillo TN, Pouliot MA, Kim HJ, Dragoo JL. Comparison of growth factor and platelet concentration from commercial platelet-rich plasma separation systems. Am J Sports Med. 2011;39(2):266-271.
16. Cho HS, Song IH, Park SY, Sung MC, Ahn MW, Song KE. Individual variation in growth factor concentrations in platelet-rich plasma and its influence on human mesenchymal stem cells. Korean J Lab Med. 2011;31(3):212-218.
17. Weibrich G, Kleis WK, Hafner G, Hitzler WE. Growth factor levels in platelet-rich plasma and correlations with donor age, sex, and platelet count. J Craniomaxillofac Surg. 2002;30(2):97-102.
18. Taylor DW, Petrera M, Hendry M, Theodoropoulos JS. A systematic review of the use of platelet-rich plasma in sports medicine as a new treatment for tendon and ligament injuries. Clin J Sport Med. 2011;21(4):344-352.
19. McCarrel T, Fortier L. Temporal growth factor release from platelet-rich plasma, trehalose lyophilized platelets, and bone marrow aspirate and their effect on tendon and ligament gene expression. J Orthop Res. 2009;27(8):1033-1042.
20. McCarrel TM, Minas T, Fortier LA. Optimization of leukocyte concentration in platelet-rich plasma for the treatment of tendinopathy. J Bone Joint Surg Am. 2012;94(19):e143(141-148).
21. Pillitteri D, Bassus S, Boller K, et al. Thrombin-induced interleukin 1beta synthesis in platelet suspensions: impact of contaminating leukocytes. Platelets. 2007;18(2):119-127.
22. Boswell SG, Schnabel LV, Mohammed HO, Sundman EA, Minas T, Fortier LA. Increasing platelet concentrations in leukocyte-reduced platelet-rich plasma decrease collagen gene synthesis in tendons. Am J Sports Med. 2014;42(1):42-49.
23. Mishra A, Pavelko T. Treatment of chronic elbow tendinosis with buffered platelet-rich plasma. Am J Sports Med. 2006;34(11):1774-1778.
24. Maniscalco P, Gambera D, Lunati A, et al. The "Cascade" membrane: a new PRP device for tendon ruptures. Description and case report on rotator cuff tendon. Acta Biomed. 2008;79(3):223-226.
25. Filardo G, Kon E, Della Villa S, Vincentelli F, Fornasari PM, Marcacci M. Use of platelet-rich plasma for the treatment of refractory jumper's knee. Int Orthop. 2010;34(6):909-915.
26. Peerbooms JC, Sluimer J, Bruijn DJ, Gosens T. Positive effect of an autologous platelet concentrate in lateral epicondylitis in a double-blind randomized controlled trial: platelet-rich plasma versus corticosteroid injection with a 1-year follow-up. Am J Sports Med. 2010;38(2):255-262.
27. de Vos RJ, Weir A, van Schie HT, et al. Platelet-rich plasma injection for chronic Achilles tendinopathy: a randomized controlled trial. JAMA. 2010;303(2):144-149.
28. Schepull T, Kvist J, Norrman H, Trinks M, Berlin G, Aspenberg P. Autologous platelets have no effect on the healing of human achilles tendon ruptures: a randomized single-blind study. Am J Sports Med. 2011;39(1):38-47.
29. Welsh KI, Burgos H, Batchelor JR. The immune response to allogeneic rat platelets; Ag-B antigens in matrix form lacking Ia. Eur J Immunol. 1977;7(5):267-272.
30. Xue M, Del Bigio MR. Intracortical hemorrhage injury in rats : relationship between blood fractions and brain cell death. Stroke. 2000;31(7):1721-1727.
31. Voleti PB, Buckley MR, Soslowsky LJ. Tendon healing: repair and regeneration. Annu Rev Biomed Eng. 2012;14:47-71.
32. Sundman EA, Cole BJ, Fortier LA. Growth factor and catabolic cytokine concentrations are influenced by the cellular composition of platelet-rich plasma. Am J Sports Med. 2011;39(10):2135-2140.
33. Palmgren MS, deShazo RD, Carter RM, Zimny ML, Shah SV. Mechanisms of neutrophil damage to human alveolar extracellular matrix: the role of serine and metalloproteases. J Allergy Clin Immunol. 1992;89(4):905-915.
34. Khalil N, Bereznay O, Sporn M, Greenberg AH. Macrophage production of transforming growth factor beta and fibroblast collagen synthesis in chronic pulmonary inflammation. J Exp Med. 1989;170(3):727-737.
35. Zhou Y, Zhang J, Wu H, Hogan MV, Wang JH. The differential effects of leukocyte-containing and pure platelet-rich plasma (PRP) on tendon stem/progenitor cells - implications of PRP application for the clinical treatment of tendon injuries. Stem Cell Res Ther. 2015;6:173.
36. Su B, O'Connor JP. NSAID therapy effects on healing of bone, tendon, and the enthesis. J Appl Physiol (1985). 2013;115(6):892-899.
TAKE-HOME POINTS
- Injection of leukocytes into healthy rat Achilles tendons increases inflammation.
- Injection of leukocytes into healthy rat Achilles tendons does not affect vascularity.
- Leukocyte-rich PRP preparations may be contraindicated in settings of acute tendonitis.
- Leukocyte-rich PRP preparations may be useful for chronic tendinosis.
- The concentration and composition of white blood cells within PRP preparations is variable and needs to be better understood in order to optimize clinical utility of PRP injections.
Real-World Evidence for Safety and Effectiveness of Repeated Courses of Hyaluronic Acid Injections on the Time to Knee Replacement Surgery
ABSTRACT
Osteoarthritis (OA) of the knee is a top cause of disability among the elderly. Total knee replacement (TKR) has been available as an effective and definite surgical method to treat severe OA of the knee. However, TKR is a significant procedure with potential risk for serious complications and high costs. Alternative lower risk therapies that can delay or obviate TKR are valuable to those who are poor candidates for surgery or wish to avoid TKR as long as possible. Given the chondroprotective effects of hyaluronic acid (HA) injections, they are a safe and effective treatment to improve pain, function, and longevity of the knee. Thus, HA features the potential to delay or obviate TKR.
We aim to study the safety and effectiveness of repeated courses of HA on the time to TKR over a 3-year period using data from a large US health plan administrative claims database.
Retrospective analyses were conducted by identifying knee OA patients during the selection period (2007-2010). The follow-up period was 36 months, post-index date of initial HA injection. Procedural outcomes and adverse events of interest were tabulated and analyzed. A Cox proportional hazards model was used to model the risk of TKR.
A total of 50,389 patients who received HA for treatment of knee OA and met the study inclusion criteria were analyzed. Successive courses of HA showed a good safety profile and led to high proportions of patients without TKR 3 years after treatment initiation. Multivariate statistical modeling showed that multiple courses of HA injections significantly decreased the rates of TKR (95.0% without TKR for ≥5 courses vs 71.6% without TKR for 1 course; hazard ratio, 0.138; P < .0001).
Repeated courses of treatment with HA are safe and are associated with the delay of TKR for up to 3 years. Additional research is needed to evaluate the effect of repeated HA courses on delaying TKR beyond a 3-year time horizon.
Continue to: Osteoarthritis (OA) of the knee...
Osteoarthritis (OA) of the knee has emerged as one of the main causes of disability in the United States. Although no currently known cure of OA can reverse the progression of the disease, total knee replacement (TKR) is an effective and definitive treatment. However, TKR is an invasive procedure with potential risk for serious complications, and it has imposed high costs on the US healthcare system, with expenses accounting for hospital expenditures of TKR estimated at $28.5 billion in 2009.1Alternative low-risk therapies that can delay or obviate TKR are valuable to a number of patients, especially the poor candidates for surgery or those who wish to avoid TKR.
Intra-articular (IA) hyaluronic acid (HA) injections have been available as a safe and effective treatment option to alleviate pain and to improve joint functions.2 Results of randomized double-blind controlled clinical trials have demonstrated the pain-relieving effect of IA HA injections.3-5 Furthermore, a recent network meta-analysis comparing various pharmacologic interventions for knee OA has confirmed the efficacy of IA HA injections, which outperformed other interventions when compared with oral placebos.6,7 IA therapies are more effective than oral therapies for knee OA pain, with IA HA injections demonstrating the most pain reduction, potentially due to the benefit associated with needle injection and aspiration. Recent experimental studies have also suggested that IA HA may provide cartilage protection, reduce inflammation, and boost the viscosity of synovial fluid;8 IA HA may also exert therapeutic effects by inhibiting bone formation in OA patients.9,10 HA possesses the potential to delay or obviate TKR. Previous research with a case series review of patients in an orthopedic specialty practice reported that the use of IA HA injections in patients with grade IV OA delayed TKR substantially.11 One study analyzed retrospective medical claims data from a single private insurer and discovered potential evidence for the modest benefit of IA HA injections in delaying TKR.12
More detailed research work on a large sample of patients with knee OA and the requirement of TKR as a condition for inclusion using US administrative claims data has demonstrated the TKR-delaying effects of IA HA injections in comparison with a control group without claims for IA HA injections.13,14 This study also uses real-world US administrative data but utilizes a different approach by starting with a sample of patients with knee OA and evidence of IA HA injections and then assessing the effect of repeated courses of HA treatment on the delay of TKR, without TKR as a mandatory condition for inclusion. All patients with knee OA within the time window were included, regardless of the need for TKR compared with previous studies which only considered patients who ultimately received TKR. Safety information and effectiveness information were examined to achieve a balanced risk-benefit assessment. We also analyzed how multiple courses of HA treatment and other potentially relevant covariates at baseline affected the risk of receiving TKR in a multivariate survival model. We aimed to achieve a realistic assessment of the clinical utility of HA injections in delaying TKR in a real-world setting using both safety and effectiveness data.
METHODS
DATA SOURCE
A retrospective cohort observational study using IMS Health’s PharMetrics Plus Health Plan Claims Database was conducted by identifying knee OA patients with claims indicating initiation of HA injection at an index date during the selection period (July 1, 2007 to June 30, 2010). All common HA agents in the US market during this period (Euflexxa, Hyalgan, Orthovisc, Supartz, and Synvisc) were selected via the corresponding J-codes and pooled for investigation of HA class effects. The follow-up period was 36 months, post-index date of the initial HA injection. Outcomes were measured, and adverse events were identified during this period. The time window for identification of adverse events was within 2 weeks from any injection during the course of therapy (evidence of an emergency room visit and/or physician office visit with requisite code). The data during the 12-month pre-index baseline period from the claims database was used to obtain information about baseline patient characteristics, such as age, gender, type of coverage, physician specialty, Charlson Comorbidity Index (CCI), major comorbidities, and major medications of interest commonly used among patients with knee OA.
STUDY SAMPLE SELECTION
The eligible patients required an outpatient claim indicating the initiation of HA injection. The date of the first claim for the patient within the selection window was defined as their index date. Patients had to be ≥18 years of age in the year of their index date. They had to present at least 1 clinical knee OA diagnosis at any point in the 12-month pre-index period (including the index date), and only patients who were continuously enrolled from 12 months pre-index to 36 months post-index date were evaluated. Among these patients (approximately 1.4 million), the following were excluded to minimize complications in data analysis and interpretation: patients with evidence of any HA use in the pre-index period; patients with evidence of a different kind of HA index medication in the post-index period; patients with evidence of TKR within 30 days of the index event during the post-index period; patients with evidence of 2 different kinds of HA index medications on the index date; and patients with evidence of diagnosis of hip OA, fibromyalgia, rheumatoid arthritis, lupus, or gout during the pre-index period.
Five patient cohorts were defined according to the number of courses of IA HA injections over the entire post-index period.
Continue to: Statistical analysis...
STATISTICAL ANALYSIS
All statistical analyses were performed using SAS version 9.2 (SAS Institute Inc.). Descriptive statistics such as means, standard deviations, medians, and 25% and 75% percentiles (Q1 and Q3, respectively) were provided for the continuous variables. Numbers and percentages were provided for the categorical variables. For statistical testing, Student’s t-tests were applied for the continuous variables and chi-square tests for the categorical variables. All the statistical tests were two-tailed. The sample sizes in this database study are remarkably large, such that differences that are not clinically important could still be statistically significant at the conventional alpha level of 0.05. Thus, we applied a more stringent requirement of the alpha level of 0.0001 to identify highly statistically significant results. The number and percentage of patients within each cohort with at least 1 instance of an adverse event of interest (those adverse events commonly expected for patients who receive IA injections for knee OA) were assessed. Times to TKR during the 36-month post-index period were analyzed and compared among different cohorts. Any patients who had not undergone TKR by the end of the post-index period were considered censored at 36 months. The Kaplan-Meier method was employed to model survival curves with time to TKR data, and log-rank tests were used to compare survival curves among different cohorts. A Cox proportional hazards model (PHM) was used to model the risk of TKR with a pre-specified set of covariates adjusted for baseline attributes, such as age, gender, comorbidities, and pre-index healthcare costs. Hazard ratios with 95% confidence intervals were used to examine the measures of event risk.
RESULTS
PATIENT CHARACTERISTICS
Applying study selection criteria to the claims database yielded 50,389 patients (Figure 1), providing an ample sample size for the statistical analysis. Only patients with evidence of knee OA and use of HA injections (the index medication of interest) were selected, regardless of whether they received TKR during the post-index period. The requirement for a knee OA diagnosis during the 12-month pre-index period resulted in the significant attrition of patients, with 584,956 patients being excluded. Among the 50,389 patients who received HA for treatment of knee OA, 36,260 (72.0%) received a single course of treatment, 8709 (17.3%) received 2 courses, 3179 (6.3%) received 3 courses, 1354 (2.7%) received 4 courses, and 887 (1.8%) received ≥5 courses of treatment.
Comparison of baseline characteristics among the 5 IA HA cohorts showed the fairly similar baseline characteristics of all cohorts (Table 1). Geographic region, physician specialty, and opioid use showed differences among the cohorts. Cohorts with ≥5 HA courses presented lower proportions of patients from Southern US states, patients seeing orthopedic surgeons, and patients using opioids than cohorts with fewer HA courses.
PROCEDURES OF INTEREST
An analysis of the procedures patients received after HA treatment initiation showed that higher numbers of HA treatment courses resulted in lower proportions of patients receiving TKR within 3 years after HA treatment initiation (Table 2). With an increasing number of HA treatment courses, the proportion of patients with TKR within 3 years post-index consistently decreased from 28.4% (for 1 HA course) to 5.0% (for ≥5 HA courses), with all differences being highly statistically significant (P < .0001). Similarly, partial knee replacement exhibited a similar trend, with the proportion of patients decreasing from 3.3% (for 1 HA course) to 0.8% (for ≥5 HA courses; P < .0001). Among the patients with TKR within 3 years post-index, increasing numbers of treatment courses correlated with increasing time to TKR, with a mean of 375.6 days (for 1 HA course) rising to a mean of 971.5 days (for ≥5 HA courses; P < .0001). On the other hand, patients with multiple courses of HA treatment were more likely to undergo radiologic examinations of the knee, arthrocenteses, and image-guided injections than patients with only a single course of HA treatment (P < .0001).
ADVERSE EVENTS
Arthralgia and joint pain in the knee were the most commonly recorded adverse events (Table 3). More courses of HA treatment were associated with higher rates of adverse events. Overall, the reported adverse events profile of repeated courses of HA treatment consisted of mostly common and mild adverse events and displayed no safety concern for patients with knee OA that was followed-up for 3 years. The causality of these adverse events directly related to HA injections vs a specific disease state cannot be determined from an administrative claims data set.
TIME TO TKR
Successive courses of HA led to high proportions of patients without TKR 3 years after HA treatment initiation. This result is evident in the Kaplan-Meier survival curves of time to TKR for different HA cohorts (Figure 2), with log-rank tests of multiple courses vs a single course of HA (P < .0001) showing highly statistically significance. Tabulation of proportions of patients without TKR by various time points showed that increasing numbers of HA treatment courses correlated with higher proportions of patients without TKR at almost all time points (Table 4); within 3 years post-index, 71.6% of patients in the 1 HA course cohort exhibited no TKR, whereas 95.0% of patients in ≥5 HA courses cohort presented no TKR. We also performed a multivariate Cox PHM (Table 5) to account for baseline characteristics of different HA cohorts with covariates when estimating the risks of receiving TKR. The results of the Cox PHM showed that multiple courses of HA treatment significantly decreased the risk of TKR (hazard ratio, 0.138 for ≥5 HA courses vs 1 HA course; P < .0001). Inspection of other highly significant covariates showed that being older, living in the Midwest region of the US (vs the Northeast), receiving pre-index corticosteroids, having an orthopedic surgeon as a treating physician (vs a general practitioner, a rheumatologist, or a physical medicine and rehabilitation specialist), experiencing hypertension or hyperlipidemia, and higher pre-index total healthcare costs were associated with an increased risk of TKR (all P < .0001). Vascular disease and high CCI scores were associated with a decreased risk of TKR (P < .0001).
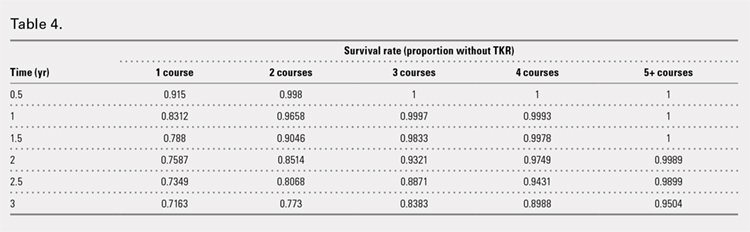
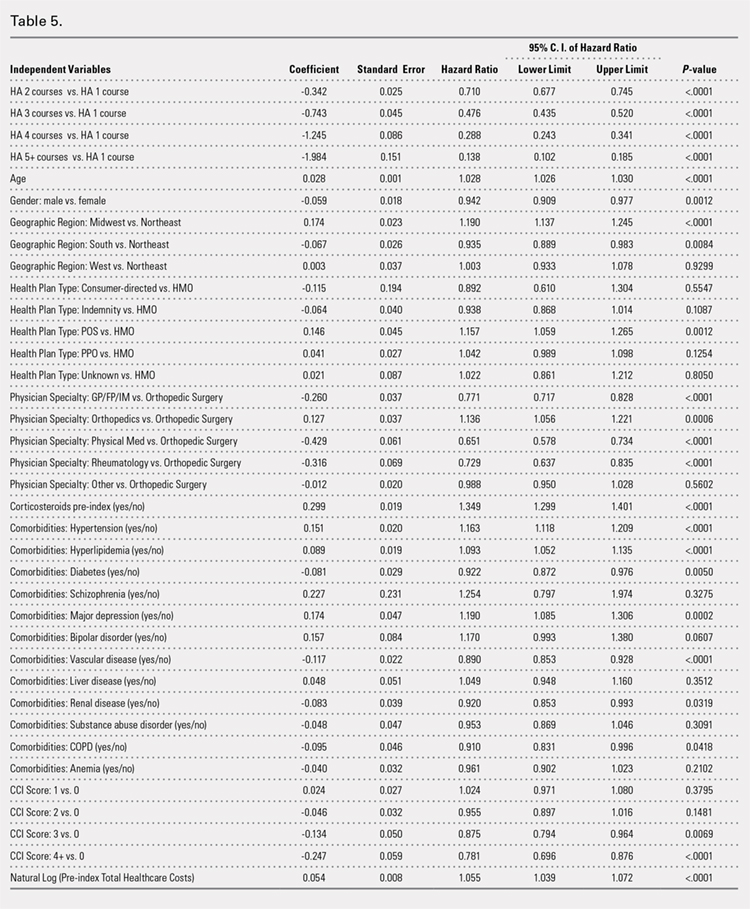
Continue to: Discussion...
DISCUSSION
This study demonstrated that multiple courses of HA treatment can delay the need for surgery for up to 3 years, with risk for both TKR and partial knee replacement decreasing in a dose-dependent manner. The potentially confounding effect of differences in baseline characteristics that could influence patients’ propensity to receive TKR in a database study was controlled by performing a multivariate analysis with covariate adjustment. The TKR-delaying effect of HA injection was more prominent in cohorts with a high number of HA treatment courses: 19 out of 20 patients in the cohort of ≥5 HA courses were free of TKR at the end of the 3-year post-index period. Such a high proportion of patients avoiding TKR with repeated courses of HA suggests that some patients may be able to successfully delay TKR well beyond the 3-year time span. This finding is counter-evidence to the frequently made assumption15 that all patients with knee OA will eventually progress to a state of disability, making TKR inevitable. The patients with end-stage radiographic knee OA can also benefit from IA HA injections for an extended period of time;16 the latest evidence indicates that nonoperative management can improve symptoms irrespective of radiographic disease severity, implying that TKR needs not to be the only therapeutic option for patients with end-stage radiographic knee OA.17 This finding suggests that HA treatment should be considered an important clinical treatment option for patients with knee OA.
Although the incidence rates of certain adverse events, such as arthralgia/joint pain, are sizable, these temporary adverse events commonly occur among patients who receive IA injections for knee OA; most of these events may simply include symptoms of the remaining underlying knee OA. These results are consistent with those of previous literature reporting the safety of repeated treatment with IA HA injections in a prospective clinical trial18 and demonstrating that repeated courses of HA treatment pose no greater safety risk than a single course of HA treatment.
Multivariate modeling outcomes of factors influencing risk of receiving TKR are broadly consistent with the generally accepted notions that different levels of disease severity and patients’ willingness to consider TKR at baseline influence the likelihood and timing of receiving TKR.19,20 Age and obesity are common risk factors for progression of OA. Orthopedic surgeons are more likely to recommend surgery than non-surgeons. The pre-index use of corticosteroids and high pre-index healthcare costs could be associated with more severe symptoms at baseline. Patients with vascular disease or severe comorbidities, as evidenced by high CCI scores, make poor candidates for major elective surgeries such as TKR. These results are intuitive and validate the clinical insights of this study. Moreover, inclusion of these covariates in the analysis model allows for indirect adjustment of the most important prognostic factors for TKR at baseline, permitting proper statistical comparison of the results for different cohort groups.
Recently, the efficacy of HA injections for OA patients has become the subject of debate when the American Academy of Orthopaedic Surgeons (AAOS) revised its clinical practice guideline, recommending against the use of HA.21 The AAOS’ findings differ from those of other clinical societies, such as the American College of Rheumatology22 and the European League Against Rheumatism,23 which provide no strong recommendation against the use of HA injections. The announcement of the new guideline by AAOS caused concern among clinicians and payers who had valued IA HA injections as a means to control knee OA pain before patients progress to TKR;24 on the other hand, the demand for nonoperative treatment of knee OA remains high. Utilization rates of TKR have increased dramatically, and surgeries are now performed on younger patients with increasing burden on the healthcare system,25,26 in spite of the fact that as high as a third of TKR surgeries may have been performed in inappropriate patients.27 Part of the confusion surrounding clinical utility of HA stems from the fact that up until recently, relatively little research looked into the practical benefits of HA in actual clinical practice. Analyses of databases such as registries are now gaining attention to overcome that problem. Examination of large administrative databases maintained by commercial payers offers the benefit of probing realistically the safety and efficacy of treatments in actual clinical environments in a very large number of patients with heterogeneous backgrounds. Recently, the Agency for Healthcare Research and Quality’s Technology Assessment Program in the US called for such studies to determine whether HA injections can delay progression to TKR.28 The results of this study and several others11,13,14,16 suggest that use of HA to treat OA of the knee is associated with the delay of TKR, supporting the utility of HA in clinical practice and the healthcare system. Potential clinical benefits of delaying TKR may include the reduced risk of aseptic loosening if younger patients can wait for TKR or more time to allow the modification of risk factors in patients who will ultimately undergo TKR.
LIMITATIONS
Follow-up period was limited to 3 years post-index date because longer follow-up data were not available at the time of the study design. If an incorrect adverse event or OA diagnosis was listed in the medical record, or if the medical record was incomplete, then patients might have been misclassified, resulting in selection bias. The claims dataset includes no uninsured and Medicare patients, as the population in the database consisted primarily of commercially-insured patients in the US. Therefore, the results are most generalizable to other commercially-insured patients in the US. Generalizability to other populations may not be assured if they differ in their accessibility to physician services or prescriptions from the patients in this study. Other treatments such as the nonsteroidal anti-inflammatory drugs used by patients were not included within the pre-specified statistical model because their potential effects were assumed to be short-lived and much less than those of corticosteroid. Including these treatments would overload the statistical model with too many covariates, leading to potential computational instability. The database used provides no information on systemic factors, including plan limits on medication use, that could affect care. Given the large and diverse nature of the healthcare plans in the database. However, these factors should not have materially affected our study results. The claims database also lacks direct indicators of OA disease severity, such as Kellgren-Lawrence scores or patient-reported outcomes, including pain and function questionnaire scores. Our multivariate analysis indirectly makes up for this deficiency by considering other baseline characteristics or clinical indicators that may be correlated with information unavailable in a claims database. Patients who opt to undergo repeated courses of HA treatment may be more inclined to avoid surgery or may naturally experience OA disease progression more slowly, making them potentially different from patients who select to undergo surgery earlier without repeated courses of HA treatment. This condition may introduce a bias that causes difficulty in proving the causality between repeated HA use and delay of TKR.
CONCLUSION
Analysis of the knee OA patient data from a real-world database showed that repeated courses of treatment with HA are safe and are associated with the delay of TKR for up to 3 years. Additional research is needed to evaluate the effects of repeated HA courses on delaying TKR beyond a 3-year period.
- Murphy L, Helmick CG. The impact of osteoarthritis in the United States: a population-health perspective. Am J Nurs. 2012;112(3 Suppl 1):S13-S19.
- Arnold W, Fullerton DS, Holder S, May CS. Viscosupplementation: managed care issues for osteoarthritis of the knee. J Manag Care Pharm. 2007;13(4 Suppl):S3-S19.
- Strand V, Conaghan PG, Lohmander LS, et al. An integrated analysis of five double-blind, randomized controlled trials evaluating the safety and efficacy of a hyaluronan product for intra-articular injection in osteoarthritis of the knee. Osteoarthritis Cartilage. 2006;14(9):859-866.
- Strand V, Baraf HS, Lavin PT, Lim S, Hosokawa H. A multicenter, randomized controlled trial comparing a single intra-articular injection of Gel-200, a new cross-linked formulation of hyaluronic acid, to phosphate buffered saline for treatment of osteoarthritis of the knee. Osteoarthritis Cartilage. 2012;20(5):350-356.
- Strand V, McIntyre LF, Beach WR, Miller LE, Block JE. Safety and efficacy of US-approved viscosupplements for knee osteoarthritis: a systematic review and meta-analysis of randomized, saline-controlled trials. J Pain Res. 2015;8:217-228.
- Bannuru RR, Schmid CH, Kent DM, Vaysbrott EE, Wong JB, McAlindon TE. Comparative effectiveness of pharmacologic interventions for knee osteoarthritis: a systematic review and network meta-analysis. Ann Intern Med. 2015;162(1):46-54.
- Mandl LA, Losina E. Relative efficacy of knee osteoarthritis treatments: are all placebos created equal? Ann Intern Med. 2015;162(1):71-72.
- Kusayama Y, Akamatsu Y, Kumagai K, Kobayashi H, Aratake M, Saito T. Changes in synovial fluid biomarkers and clinical efficacy of intra-articular injections of hyaluronic acid for patients with knee osteoarthritis. J Exp Orthop. 2014;1(1):16. doi:10.1186/s40634-014-0016-7.
- Kaneko K, Higuchi C, Kunugiza Y, et al. Hyaluronan inhibits BMP-induced osteoblast differentiation. FEBS Lett. 2015;589(4):447-454. doi:10.1016/j.febslet.2014.
- Altman RD, Manjoo A, Fierlinger A, Niazi F, Nicholls M. The mechanism of action for hyaluronic acid treatment in the osteoarthritic knee: a systematic review. BMC Musculoskelet Disord. 2015;16:321. doi:10.1186/s12891-015-0775-z.
- Waddell DD, Bricker DC. Total knee replacement delayed with hylan G-F 20 use in patients with grade IV osteoarthritis. J Manag Care Pharm. 2007;13(2):113-121.
- Khan T, Nanchanatt G, Farber K, Jan S. Analysis of the effectiveness of hyaluronic acid in prevention of total knee replacement in osteoarthritis patients. J Manag Care Pharm. 2014;20:S49.
- Abbott T, Altman RD, Dimeff R, et al. Do hyaluronic acid injections delay total knee replacement surgery? Arthritis Rheum. 2013;65(Suppl 10):2139.
- Altman R, Lim S, Steen R, Dasa V. Intra-articular hyaluronic acid delays total knee replacement in patients with knee osteoarthritis: evidence from a large U.S. health claims database. Osteoarthritis Cartilage. 2015;23(Suppl 2):A403-A404.
- Mather RC 3rd, Hug KT, Orlando LA, et al. Economic evaluation of access to musculoskeletal care: the case of waiting for total knee arthroplasty. BMC Musculoskelet Disord. 2014;15:22. doi:10.1186/1471-2474-15-22.
- Waddell DD, Joseph B. Delayed total knee replacement with Hylan G-F 20. J Knee Surg. 2016;29(2):159-168. doi:10.1055/s-0034-1395281.
- Atukorala I, Makovey J, Williams M, Ochoa Albiztegui E, Eyles JP, Hunter DJ. If you have end-stage radiographic knee osteoarthritis can you respond to non-surgical management? Osteoarthritis Cartilage. 2015;23(Suppl 2):A329.
- Strand V, Baraf HS, Lavin PT, Lim S, Hosokawa H. Effectiveness and safety of a multicenter extension and retreatment trial of Gel-200 in patients with knee osteoarthritis. Cartilage. 2012;3(4):297-304. doi:10.1177/1947603512451024.
- Riddle DL, Kong X, Jiranek WA. Two-year incidence and predictors of future knee arthroplasty in persons with symptomatic knee osteoarthritis: preliminary analysis of longitudinal data from the osteoarthritis initiative. Knee. 2009;16(6):494-500.
- Hawker GA, Guan J, Croxford R, et al. A prospective population-based study of the predictors of undergoing total joint arthroplasty. Arthritis Rheum. 2006;54(10):3212-3220.
- Jevsevar DS. Treatment of osteoarthritis of the knee: evidence-based guideline, 2nd edition. J Am Acad Orthop Surg. 2013;21(9):571-576. doi:10.5435/JAAOS-21-09-571.
- Hochberg MC, Altman RD, April KT, et al. American College of Rheumatology 2012 recommendations for the use of nonpharmacologic and pharmacologic therapies in osteoarthritis of the hand, hip, and knee. Arthritis Care Res (Hoboken). 2012;64(4):465-474.
- Jordan KM, Arden NK, Doherty M, et al. EULAR Recommendations 2003: an evidence based approach to the management of knee osteoarthritis: report of a task force of the standing committee for international clinical studies including therapeutic trials (ESCISIT). Ann Rheum Dis. 2003;62(12):1145-1155.
- Bannuru RR, Vaysbrot EE, McIntyre LF. Did the American Academy of Orthopaedic Surgeons osteoarthritis guidelines miss the mark? Arthroscopy. 2014;30(1):86-89. doi:10.1016/j.arthro.2013.10.007.
- Losina E, Thornhill TS, Rome BN, Wright J, Katz JN. The dramatic increase in total knee replacement utilization rates in the United States cannot be fully explained by growth in population size and the obesity epidemic. J Bone Joint Surg Am. 2012;94(3):201-207. doi:10.2106/JBJS.J.01958.
- Weinstein AM, Rome BN, Reichmann WM, et al. Estimating the burden of total knee replacement in the United States. J Bone Joint Surg Am. 2013;95(5):385-392. doi:10.2106/JBJS.L.00206.
- Riddle DL, Jiranek WA, Hayes CW. Use of a validated algorithm to judge the appropriateness of total knee arthroplasty in the United States: a multicenter longitudinal cohort study. Arthritis Rheumatol. 2014;66(8):2134-2143. doi:10.1002/art.38685.
- NewBerry SJ, Fitzgerald JD, Maglione MA, et al. Agency for Healthcare Research and Quality Web site. Systematic Review for Effectiveness of Hyaluronic Acid in the Treatment of Severe Degenerative Joint Disease (DJD) of the Knee: Technology Assessment Report. http://www.ahrq.gov/research/findings/ta/call-for-public-review.html. Published July 23, 2015. Accessed December 22, 2014.
ABSTRACT
Osteoarthritis (OA) of the knee is a top cause of disability among the elderly. Total knee replacement (TKR) has been available as an effective and definite surgical method to treat severe OA of the knee. However, TKR is a significant procedure with potential risk for serious complications and high costs. Alternative lower risk therapies that can delay or obviate TKR are valuable to those who are poor candidates for surgery or wish to avoid TKR as long as possible. Given the chondroprotective effects of hyaluronic acid (HA) injections, they are a safe and effective treatment to improve pain, function, and longevity of the knee. Thus, HA features the potential to delay or obviate TKR.
We aim to study the safety and effectiveness of repeated courses of HA on the time to TKR over a 3-year period using data from a large US health plan administrative claims database.
Retrospective analyses were conducted by identifying knee OA patients during the selection period (2007-2010). The follow-up period was 36 months, post-index date of initial HA injection. Procedural outcomes and adverse events of interest were tabulated and analyzed. A Cox proportional hazards model was used to model the risk of TKR.
A total of 50,389 patients who received HA for treatment of knee OA and met the study inclusion criteria were analyzed. Successive courses of HA showed a good safety profile and led to high proportions of patients without TKR 3 years after treatment initiation. Multivariate statistical modeling showed that multiple courses of HA injections significantly decreased the rates of TKR (95.0% without TKR for ≥5 courses vs 71.6% without TKR for 1 course; hazard ratio, 0.138; P < .0001).
Repeated courses of treatment with HA are safe and are associated with the delay of TKR for up to 3 years. Additional research is needed to evaluate the effect of repeated HA courses on delaying TKR beyond a 3-year time horizon.
Continue to: Osteoarthritis (OA) of the knee...
Osteoarthritis (OA) of the knee has emerged as one of the main causes of disability in the United States. Although no currently known cure of OA can reverse the progression of the disease, total knee replacement (TKR) is an effective and definitive treatment. However, TKR is an invasive procedure with potential risk for serious complications, and it has imposed high costs on the US healthcare system, with expenses accounting for hospital expenditures of TKR estimated at $28.5 billion in 2009.1Alternative low-risk therapies that can delay or obviate TKR are valuable to a number of patients, especially the poor candidates for surgery or those who wish to avoid TKR.
Intra-articular (IA) hyaluronic acid (HA) injections have been available as a safe and effective treatment option to alleviate pain and to improve joint functions.2 Results of randomized double-blind controlled clinical trials have demonstrated the pain-relieving effect of IA HA injections.3-5 Furthermore, a recent network meta-analysis comparing various pharmacologic interventions for knee OA has confirmed the efficacy of IA HA injections, which outperformed other interventions when compared with oral placebos.6,7 IA therapies are more effective than oral therapies for knee OA pain, with IA HA injections demonstrating the most pain reduction, potentially due to the benefit associated with needle injection and aspiration. Recent experimental studies have also suggested that IA HA may provide cartilage protection, reduce inflammation, and boost the viscosity of synovial fluid;8 IA HA may also exert therapeutic effects by inhibiting bone formation in OA patients.9,10 HA possesses the potential to delay or obviate TKR. Previous research with a case series review of patients in an orthopedic specialty practice reported that the use of IA HA injections in patients with grade IV OA delayed TKR substantially.11 One study analyzed retrospective medical claims data from a single private insurer and discovered potential evidence for the modest benefit of IA HA injections in delaying TKR.12
More detailed research work on a large sample of patients with knee OA and the requirement of TKR as a condition for inclusion using US administrative claims data has demonstrated the TKR-delaying effects of IA HA injections in comparison with a control group without claims for IA HA injections.13,14 This study also uses real-world US administrative data but utilizes a different approach by starting with a sample of patients with knee OA and evidence of IA HA injections and then assessing the effect of repeated courses of HA treatment on the delay of TKR, without TKR as a mandatory condition for inclusion. All patients with knee OA within the time window were included, regardless of the need for TKR compared with previous studies which only considered patients who ultimately received TKR. Safety information and effectiveness information were examined to achieve a balanced risk-benefit assessment. We also analyzed how multiple courses of HA treatment and other potentially relevant covariates at baseline affected the risk of receiving TKR in a multivariate survival model. We aimed to achieve a realistic assessment of the clinical utility of HA injections in delaying TKR in a real-world setting using both safety and effectiveness data.
METHODS
DATA SOURCE
A retrospective cohort observational study using IMS Health’s PharMetrics Plus Health Plan Claims Database was conducted by identifying knee OA patients with claims indicating initiation of HA injection at an index date during the selection period (July 1, 2007 to June 30, 2010). All common HA agents in the US market during this period (Euflexxa, Hyalgan, Orthovisc, Supartz, and Synvisc) were selected via the corresponding J-codes and pooled for investigation of HA class effects. The follow-up period was 36 months, post-index date of the initial HA injection. Outcomes were measured, and adverse events were identified during this period. The time window for identification of adverse events was within 2 weeks from any injection during the course of therapy (evidence of an emergency room visit and/or physician office visit with requisite code). The data during the 12-month pre-index baseline period from the claims database was used to obtain information about baseline patient characteristics, such as age, gender, type of coverage, physician specialty, Charlson Comorbidity Index (CCI), major comorbidities, and major medications of interest commonly used among patients with knee OA.
STUDY SAMPLE SELECTION
The eligible patients required an outpatient claim indicating the initiation of HA injection. The date of the first claim for the patient within the selection window was defined as their index date. Patients had to be ≥18 years of age in the year of their index date. They had to present at least 1 clinical knee OA diagnosis at any point in the 12-month pre-index period (including the index date), and only patients who were continuously enrolled from 12 months pre-index to 36 months post-index date were evaluated. Among these patients (approximately 1.4 million), the following were excluded to minimize complications in data analysis and interpretation: patients with evidence of any HA use in the pre-index period; patients with evidence of a different kind of HA index medication in the post-index period; patients with evidence of TKR within 30 days of the index event during the post-index period; patients with evidence of 2 different kinds of HA index medications on the index date; and patients with evidence of diagnosis of hip OA, fibromyalgia, rheumatoid arthritis, lupus, or gout during the pre-index period.
Five patient cohorts were defined according to the number of courses of IA HA injections over the entire post-index period.
Continue to: Statistical analysis...
STATISTICAL ANALYSIS
All statistical analyses were performed using SAS version 9.2 (SAS Institute Inc.). Descriptive statistics such as means, standard deviations, medians, and 25% and 75% percentiles (Q1 and Q3, respectively) were provided for the continuous variables. Numbers and percentages were provided for the categorical variables. For statistical testing, Student’s t-tests were applied for the continuous variables and chi-square tests for the categorical variables. All the statistical tests were two-tailed. The sample sizes in this database study are remarkably large, such that differences that are not clinically important could still be statistically significant at the conventional alpha level of 0.05. Thus, we applied a more stringent requirement of the alpha level of 0.0001 to identify highly statistically significant results. The number and percentage of patients within each cohort with at least 1 instance of an adverse event of interest (those adverse events commonly expected for patients who receive IA injections for knee OA) were assessed. Times to TKR during the 36-month post-index period were analyzed and compared among different cohorts. Any patients who had not undergone TKR by the end of the post-index period were considered censored at 36 months. The Kaplan-Meier method was employed to model survival curves with time to TKR data, and log-rank tests were used to compare survival curves among different cohorts. A Cox proportional hazards model (PHM) was used to model the risk of TKR with a pre-specified set of covariates adjusted for baseline attributes, such as age, gender, comorbidities, and pre-index healthcare costs. Hazard ratios with 95% confidence intervals were used to examine the measures of event risk.
RESULTS
PATIENT CHARACTERISTICS
Applying study selection criteria to the claims database yielded 50,389 patients (Figure 1), providing an ample sample size for the statistical analysis. Only patients with evidence of knee OA and use of HA injections (the index medication of interest) were selected, regardless of whether they received TKR during the post-index period. The requirement for a knee OA diagnosis during the 12-month pre-index period resulted in the significant attrition of patients, with 584,956 patients being excluded. Among the 50,389 patients who received HA for treatment of knee OA, 36,260 (72.0%) received a single course of treatment, 8709 (17.3%) received 2 courses, 3179 (6.3%) received 3 courses, 1354 (2.7%) received 4 courses, and 887 (1.8%) received ≥5 courses of treatment.
Comparison of baseline characteristics among the 5 IA HA cohorts showed the fairly similar baseline characteristics of all cohorts (Table 1). Geographic region, physician specialty, and opioid use showed differences among the cohorts. Cohorts with ≥5 HA courses presented lower proportions of patients from Southern US states, patients seeing orthopedic surgeons, and patients using opioids than cohorts with fewer HA courses.
PROCEDURES OF INTEREST
An analysis of the procedures patients received after HA treatment initiation showed that higher numbers of HA treatment courses resulted in lower proportions of patients receiving TKR within 3 years after HA treatment initiation (Table 2). With an increasing number of HA treatment courses, the proportion of patients with TKR within 3 years post-index consistently decreased from 28.4% (for 1 HA course) to 5.0% (for ≥5 HA courses), with all differences being highly statistically significant (P < .0001). Similarly, partial knee replacement exhibited a similar trend, with the proportion of patients decreasing from 3.3% (for 1 HA course) to 0.8% (for ≥5 HA courses; P < .0001). Among the patients with TKR within 3 years post-index, increasing numbers of treatment courses correlated with increasing time to TKR, with a mean of 375.6 days (for 1 HA course) rising to a mean of 971.5 days (for ≥5 HA courses; P < .0001). On the other hand, patients with multiple courses of HA treatment were more likely to undergo radiologic examinations of the knee, arthrocenteses, and image-guided injections than patients with only a single course of HA treatment (P < .0001).
ADVERSE EVENTS
Arthralgia and joint pain in the knee were the most commonly recorded adverse events (Table 3). More courses of HA treatment were associated with higher rates of adverse events. Overall, the reported adverse events profile of repeated courses of HA treatment consisted of mostly common and mild adverse events and displayed no safety concern for patients with knee OA that was followed-up for 3 years. The causality of these adverse events directly related to HA injections vs a specific disease state cannot be determined from an administrative claims data set.
TIME TO TKR
Successive courses of HA led to high proportions of patients without TKR 3 years after HA treatment initiation. This result is evident in the Kaplan-Meier survival curves of time to TKR for different HA cohorts (Figure 2), with log-rank tests of multiple courses vs a single course of HA (P < .0001) showing highly statistically significance. Tabulation of proportions of patients without TKR by various time points showed that increasing numbers of HA treatment courses correlated with higher proportions of patients without TKR at almost all time points (Table 4); within 3 years post-index, 71.6% of patients in the 1 HA course cohort exhibited no TKR, whereas 95.0% of patients in ≥5 HA courses cohort presented no TKR. We also performed a multivariate Cox PHM (Table 5) to account for baseline characteristics of different HA cohorts with covariates when estimating the risks of receiving TKR. The results of the Cox PHM showed that multiple courses of HA treatment significantly decreased the risk of TKR (hazard ratio, 0.138 for ≥5 HA courses vs 1 HA course; P < .0001). Inspection of other highly significant covariates showed that being older, living in the Midwest region of the US (vs the Northeast), receiving pre-index corticosteroids, having an orthopedic surgeon as a treating physician (vs a general practitioner, a rheumatologist, or a physical medicine and rehabilitation specialist), experiencing hypertension or hyperlipidemia, and higher pre-index total healthcare costs were associated with an increased risk of TKR (all P < .0001). Vascular disease and high CCI scores were associated with a decreased risk of TKR (P < .0001).
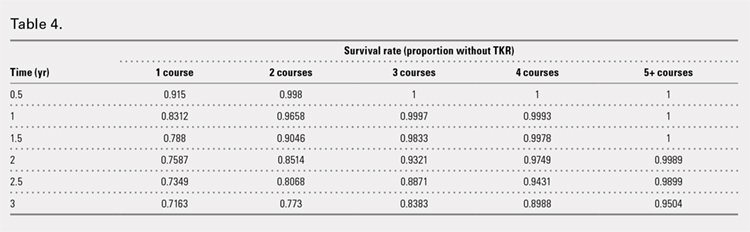
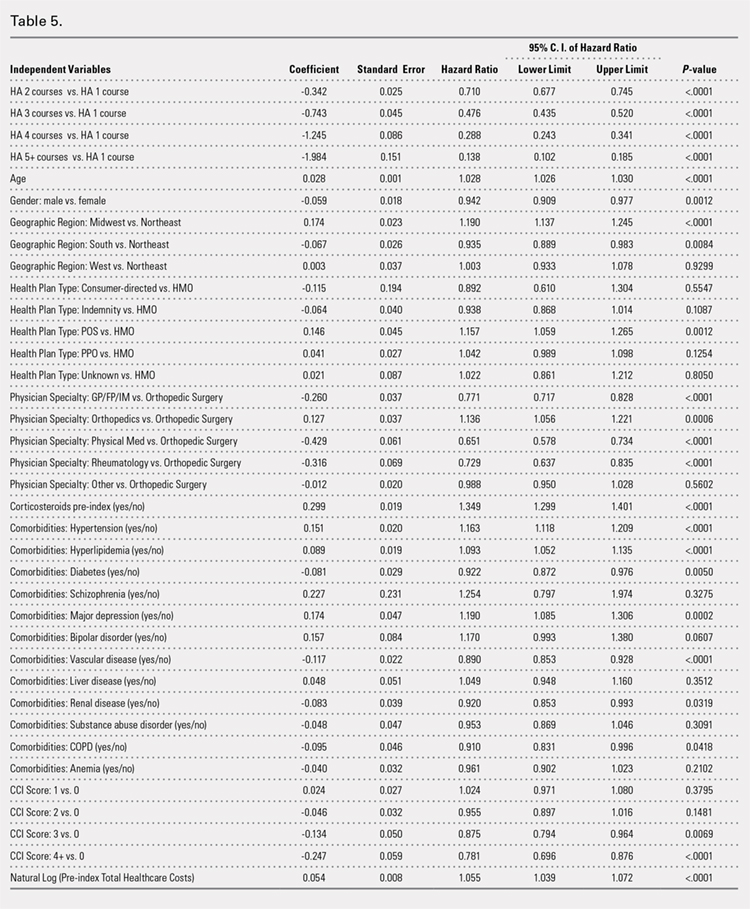
Continue to: Discussion...
DISCUSSION
This study demonstrated that multiple courses of HA treatment can delay the need for surgery for up to 3 years, with risk for both TKR and partial knee replacement decreasing in a dose-dependent manner. The potentially confounding effect of differences in baseline characteristics that could influence patients’ propensity to receive TKR in a database study was controlled by performing a multivariate analysis with covariate adjustment. The TKR-delaying effect of HA injection was more prominent in cohorts with a high number of HA treatment courses: 19 out of 20 patients in the cohort of ≥5 HA courses were free of TKR at the end of the 3-year post-index period. Such a high proportion of patients avoiding TKR with repeated courses of HA suggests that some patients may be able to successfully delay TKR well beyond the 3-year time span. This finding is counter-evidence to the frequently made assumption15 that all patients with knee OA will eventually progress to a state of disability, making TKR inevitable. The patients with end-stage radiographic knee OA can also benefit from IA HA injections for an extended period of time;16 the latest evidence indicates that nonoperative management can improve symptoms irrespective of radiographic disease severity, implying that TKR needs not to be the only therapeutic option for patients with end-stage radiographic knee OA.17 This finding suggests that HA treatment should be considered an important clinical treatment option for patients with knee OA.
Although the incidence rates of certain adverse events, such as arthralgia/joint pain, are sizable, these temporary adverse events commonly occur among patients who receive IA injections for knee OA; most of these events may simply include symptoms of the remaining underlying knee OA. These results are consistent with those of previous literature reporting the safety of repeated treatment with IA HA injections in a prospective clinical trial18 and demonstrating that repeated courses of HA treatment pose no greater safety risk than a single course of HA treatment.
Multivariate modeling outcomes of factors influencing risk of receiving TKR are broadly consistent with the generally accepted notions that different levels of disease severity and patients’ willingness to consider TKR at baseline influence the likelihood and timing of receiving TKR.19,20 Age and obesity are common risk factors for progression of OA. Orthopedic surgeons are more likely to recommend surgery than non-surgeons. The pre-index use of corticosteroids and high pre-index healthcare costs could be associated with more severe symptoms at baseline. Patients with vascular disease or severe comorbidities, as evidenced by high CCI scores, make poor candidates for major elective surgeries such as TKR. These results are intuitive and validate the clinical insights of this study. Moreover, inclusion of these covariates in the analysis model allows for indirect adjustment of the most important prognostic factors for TKR at baseline, permitting proper statistical comparison of the results for different cohort groups.
Recently, the efficacy of HA injections for OA patients has become the subject of debate when the American Academy of Orthopaedic Surgeons (AAOS) revised its clinical practice guideline, recommending against the use of HA.21 The AAOS’ findings differ from those of other clinical societies, such as the American College of Rheumatology22 and the European League Against Rheumatism,23 which provide no strong recommendation against the use of HA injections. The announcement of the new guideline by AAOS caused concern among clinicians and payers who had valued IA HA injections as a means to control knee OA pain before patients progress to TKR;24 on the other hand, the demand for nonoperative treatment of knee OA remains high. Utilization rates of TKR have increased dramatically, and surgeries are now performed on younger patients with increasing burden on the healthcare system,25,26 in spite of the fact that as high as a third of TKR surgeries may have been performed in inappropriate patients.27 Part of the confusion surrounding clinical utility of HA stems from the fact that up until recently, relatively little research looked into the practical benefits of HA in actual clinical practice. Analyses of databases such as registries are now gaining attention to overcome that problem. Examination of large administrative databases maintained by commercial payers offers the benefit of probing realistically the safety and efficacy of treatments in actual clinical environments in a very large number of patients with heterogeneous backgrounds. Recently, the Agency for Healthcare Research and Quality’s Technology Assessment Program in the US called for such studies to determine whether HA injections can delay progression to TKR.28 The results of this study and several others11,13,14,16 suggest that use of HA to treat OA of the knee is associated with the delay of TKR, supporting the utility of HA in clinical practice and the healthcare system. Potential clinical benefits of delaying TKR may include the reduced risk of aseptic loosening if younger patients can wait for TKR or more time to allow the modification of risk factors in patients who will ultimately undergo TKR.
LIMITATIONS
Follow-up period was limited to 3 years post-index date because longer follow-up data were not available at the time of the study design. If an incorrect adverse event or OA diagnosis was listed in the medical record, or if the medical record was incomplete, then patients might have been misclassified, resulting in selection bias. The claims dataset includes no uninsured and Medicare patients, as the population in the database consisted primarily of commercially-insured patients in the US. Therefore, the results are most generalizable to other commercially-insured patients in the US. Generalizability to other populations may not be assured if they differ in their accessibility to physician services or prescriptions from the patients in this study. Other treatments such as the nonsteroidal anti-inflammatory drugs used by patients were not included within the pre-specified statistical model because their potential effects were assumed to be short-lived and much less than those of corticosteroid. Including these treatments would overload the statistical model with too many covariates, leading to potential computational instability. The database used provides no information on systemic factors, including plan limits on medication use, that could affect care. Given the large and diverse nature of the healthcare plans in the database. However, these factors should not have materially affected our study results. The claims database also lacks direct indicators of OA disease severity, such as Kellgren-Lawrence scores or patient-reported outcomes, including pain and function questionnaire scores. Our multivariate analysis indirectly makes up for this deficiency by considering other baseline characteristics or clinical indicators that may be correlated with information unavailable in a claims database. Patients who opt to undergo repeated courses of HA treatment may be more inclined to avoid surgery or may naturally experience OA disease progression more slowly, making them potentially different from patients who select to undergo surgery earlier without repeated courses of HA treatment. This condition may introduce a bias that causes difficulty in proving the causality between repeated HA use and delay of TKR.
CONCLUSION
Analysis of the knee OA patient data from a real-world database showed that repeated courses of treatment with HA are safe and are associated with the delay of TKR for up to 3 years. Additional research is needed to evaluate the effects of repeated HA courses on delaying TKR beyond a 3-year period.
ABSTRACT
Osteoarthritis (OA) of the knee is a top cause of disability among the elderly. Total knee replacement (TKR) has been available as an effective and definite surgical method to treat severe OA of the knee. However, TKR is a significant procedure with potential risk for serious complications and high costs. Alternative lower risk therapies that can delay or obviate TKR are valuable to those who are poor candidates for surgery or wish to avoid TKR as long as possible. Given the chondroprotective effects of hyaluronic acid (HA) injections, they are a safe and effective treatment to improve pain, function, and longevity of the knee. Thus, HA features the potential to delay or obviate TKR.
We aim to study the safety and effectiveness of repeated courses of HA on the time to TKR over a 3-year period using data from a large US health plan administrative claims database.
Retrospective analyses were conducted by identifying knee OA patients during the selection period (2007-2010). The follow-up period was 36 months, post-index date of initial HA injection. Procedural outcomes and adverse events of interest were tabulated and analyzed. A Cox proportional hazards model was used to model the risk of TKR.
A total of 50,389 patients who received HA for treatment of knee OA and met the study inclusion criteria were analyzed. Successive courses of HA showed a good safety profile and led to high proportions of patients without TKR 3 years after treatment initiation. Multivariate statistical modeling showed that multiple courses of HA injections significantly decreased the rates of TKR (95.0% without TKR for ≥5 courses vs 71.6% without TKR for 1 course; hazard ratio, 0.138; P < .0001).
Repeated courses of treatment with HA are safe and are associated with the delay of TKR for up to 3 years. Additional research is needed to evaluate the effect of repeated HA courses on delaying TKR beyond a 3-year time horizon.
Continue to: Osteoarthritis (OA) of the knee...
Osteoarthritis (OA) of the knee has emerged as one of the main causes of disability in the United States. Although no currently known cure of OA can reverse the progression of the disease, total knee replacement (TKR) is an effective and definitive treatment. However, TKR is an invasive procedure with potential risk for serious complications, and it has imposed high costs on the US healthcare system, with expenses accounting for hospital expenditures of TKR estimated at $28.5 billion in 2009.1Alternative low-risk therapies that can delay or obviate TKR are valuable to a number of patients, especially the poor candidates for surgery or those who wish to avoid TKR.
Intra-articular (IA) hyaluronic acid (HA) injections have been available as a safe and effective treatment option to alleviate pain and to improve joint functions.2 Results of randomized double-blind controlled clinical trials have demonstrated the pain-relieving effect of IA HA injections.3-5 Furthermore, a recent network meta-analysis comparing various pharmacologic interventions for knee OA has confirmed the efficacy of IA HA injections, which outperformed other interventions when compared with oral placebos.6,7 IA therapies are more effective than oral therapies for knee OA pain, with IA HA injections demonstrating the most pain reduction, potentially due to the benefit associated with needle injection and aspiration. Recent experimental studies have also suggested that IA HA may provide cartilage protection, reduce inflammation, and boost the viscosity of synovial fluid;8 IA HA may also exert therapeutic effects by inhibiting bone formation in OA patients.9,10 HA possesses the potential to delay or obviate TKR. Previous research with a case series review of patients in an orthopedic specialty practice reported that the use of IA HA injections in patients with grade IV OA delayed TKR substantially.11 One study analyzed retrospective medical claims data from a single private insurer and discovered potential evidence for the modest benefit of IA HA injections in delaying TKR.12
More detailed research work on a large sample of patients with knee OA and the requirement of TKR as a condition for inclusion using US administrative claims data has demonstrated the TKR-delaying effects of IA HA injections in comparison with a control group without claims for IA HA injections.13,14 This study also uses real-world US administrative data but utilizes a different approach by starting with a sample of patients with knee OA and evidence of IA HA injections and then assessing the effect of repeated courses of HA treatment on the delay of TKR, without TKR as a mandatory condition for inclusion. All patients with knee OA within the time window were included, regardless of the need for TKR compared with previous studies which only considered patients who ultimately received TKR. Safety information and effectiveness information were examined to achieve a balanced risk-benefit assessment. We also analyzed how multiple courses of HA treatment and other potentially relevant covariates at baseline affected the risk of receiving TKR in a multivariate survival model. We aimed to achieve a realistic assessment of the clinical utility of HA injections in delaying TKR in a real-world setting using both safety and effectiveness data.
METHODS
DATA SOURCE
A retrospective cohort observational study using IMS Health’s PharMetrics Plus Health Plan Claims Database was conducted by identifying knee OA patients with claims indicating initiation of HA injection at an index date during the selection period (July 1, 2007 to June 30, 2010). All common HA agents in the US market during this period (Euflexxa, Hyalgan, Orthovisc, Supartz, and Synvisc) were selected via the corresponding J-codes and pooled for investigation of HA class effects. The follow-up period was 36 months, post-index date of the initial HA injection. Outcomes were measured, and adverse events were identified during this period. The time window for identification of adverse events was within 2 weeks from any injection during the course of therapy (evidence of an emergency room visit and/or physician office visit with requisite code). The data during the 12-month pre-index baseline period from the claims database was used to obtain information about baseline patient characteristics, such as age, gender, type of coverage, physician specialty, Charlson Comorbidity Index (CCI), major comorbidities, and major medications of interest commonly used among patients with knee OA.
STUDY SAMPLE SELECTION
The eligible patients required an outpatient claim indicating the initiation of HA injection. The date of the first claim for the patient within the selection window was defined as their index date. Patients had to be ≥18 years of age in the year of their index date. They had to present at least 1 clinical knee OA diagnosis at any point in the 12-month pre-index period (including the index date), and only patients who were continuously enrolled from 12 months pre-index to 36 months post-index date were evaluated. Among these patients (approximately 1.4 million), the following were excluded to minimize complications in data analysis and interpretation: patients with evidence of any HA use in the pre-index period; patients with evidence of a different kind of HA index medication in the post-index period; patients with evidence of TKR within 30 days of the index event during the post-index period; patients with evidence of 2 different kinds of HA index medications on the index date; and patients with evidence of diagnosis of hip OA, fibromyalgia, rheumatoid arthritis, lupus, or gout during the pre-index period.
Five patient cohorts were defined according to the number of courses of IA HA injections over the entire post-index period.
Continue to: Statistical analysis...
STATISTICAL ANALYSIS
All statistical analyses were performed using SAS version 9.2 (SAS Institute Inc.). Descriptive statistics such as means, standard deviations, medians, and 25% and 75% percentiles (Q1 and Q3, respectively) were provided for the continuous variables. Numbers and percentages were provided for the categorical variables. For statistical testing, Student’s t-tests were applied for the continuous variables and chi-square tests for the categorical variables. All the statistical tests were two-tailed. The sample sizes in this database study are remarkably large, such that differences that are not clinically important could still be statistically significant at the conventional alpha level of 0.05. Thus, we applied a more stringent requirement of the alpha level of 0.0001 to identify highly statistically significant results. The number and percentage of patients within each cohort with at least 1 instance of an adverse event of interest (those adverse events commonly expected for patients who receive IA injections for knee OA) were assessed. Times to TKR during the 36-month post-index period were analyzed and compared among different cohorts. Any patients who had not undergone TKR by the end of the post-index period were considered censored at 36 months. The Kaplan-Meier method was employed to model survival curves with time to TKR data, and log-rank tests were used to compare survival curves among different cohorts. A Cox proportional hazards model (PHM) was used to model the risk of TKR with a pre-specified set of covariates adjusted for baseline attributes, such as age, gender, comorbidities, and pre-index healthcare costs. Hazard ratios with 95% confidence intervals were used to examine the measures of event risk.
RESULTS
PATIENT CHARACTERISTICS
Applying study selection criteria to the claims database yielded 50,389 patients (Figure 1), providing an ample sample size for the statistical analysis. Only patients with evidence of knee OA and use of HA injections (the index medication of interest) were selected, regardless of whether they received TKR during the post-index period. The requirement for a knee OA diagnosis during the 12-month pre-index period resulted in the significant attrition of patients, with 584,956 patients being excluded. Among the 50,389 patients who received HA for treatment of knee OA, 36,260 (72.0%) received a single course of treatment, 8709 (17.3%) received 2 courses, 3179 (6.3%) received 3 courses, 1354 (2.7%) received 4 courses, and 887 (1.8%) received ≥5 courses of treatment.
Comparison of baseline characteristics among the 5 IA HA cohorts showed the fairly similar baseline characteristics of all cohorts (Table 1). Geographic region, physician specialty, and opioid use showed differences among the cohorts. Cohorts with ≥5 HA courses presented lower proportions of patients from Southern US states, patients seeing orthopedic surgeons, and patients using opioids than cohorts with fewer HA courses.
PROCEDURES OF INTEREST
An analysis of the procedures patients received after HA treatment initiation showed that higher numbers of HA treatment courses resulted in lower proportions of patients receiving TKR within 3 years after HA treatment initiation (Table 2). With an increasing number of HA treatment courses, the proportion of patients with TKR within 3 years post-index consistently decreased from 28.4% (for 1 HA course) to 5.0% (for ≥5 HA courses), with all differences being highly statistically significant (P < .0001). Similarly, partial knee replacement exhibited a similar trend, with the proportion of patients decreasing from 3.3% (for 1 HA course) to 0.8% (for ≥5 HA courses; P < .0001). Among the patients with TKR within 3 years post-index, increasing numbers of treatment courses correlated with increasing time to TKR, with a mean of 375.6 days (for 1 HA course) rising to a mean of 971.5 days (for ≥5 HA courses; P < .0001). On the other hand, patients with multiple courses of HA treatment were more likely to undergo radiologic examinations of the knee, arthrocenteses, and image-guided injections than patients with only a single course of HA treatment (P < .0001).
ADVERSE EVENTS
Arthralgia and joint pain in the knee were the most commonly recorded adverse events (Table 3). More courses of HA treatment were associated with higher rates of adverse events. Overall, the reported adverse events profile of repeated courses of HA treatment consisted of mostly common and mild adverse events and displayed no safety concern for patients with knee OA that was followed-up for 3 years. The causality of these adverse events directly related to HA injections vs a specific disease state cannot be determined from an administrative claims data set.
TIME TO TKR
Successive courses of HA led to high proportions of patients without TKR 3 years after HA treatment initiation. This result is evident in the Kaplan-Meier survival curves of time to TKR for different HA cohorts (Figure 2), with log-rank tests of multiple courses vs a single course of HA (P < .0001) showing highly statistically significance. Tabulation of proportions of patients without TKR by various time points showed that increasing numbers of HA treatment courses correlated with higher proportions of patients without TKR at almost all time points (Table 4); within 3 years post-index, 71.6% of patients in the 1 HA course cohort exhibited no TKR, whereas 95.0% of patients in ≥5 HA courses cohort presented no TKR. We also performed a multivariate Cox PHM (Table 5) to account for baseline characteristics of different HA cohorts with covariates when estimating the risks of receiving TKR. The results of the Cox PHM showed that multiple courses of HA treatment significantly decreased the risk of TKR (hazard ratio, 0.138 for ≥5 HA courses vs 1 HA course; P < .0001). Inspection of other highly significant covariates showed that being older, living in the Midwest region of the US (vs the Northeast), receiving pre-index corticosteroids, having an orthopedic surgeon as a treating physician (vs a general practitioner, a rheumatologist, or a physical medicine and rehabilitation specialist), experiencing hypertension or hyperlipidemia, and higher pre-index total healthcare costs were associated with an increased risk of TKR (all P < .0001). Vascular disease and high CCI scores were associated with a decreased risk of TKR (P < .0001).
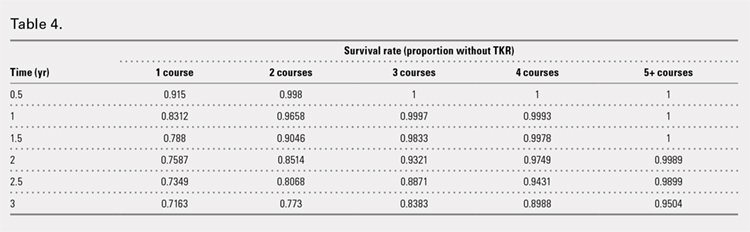
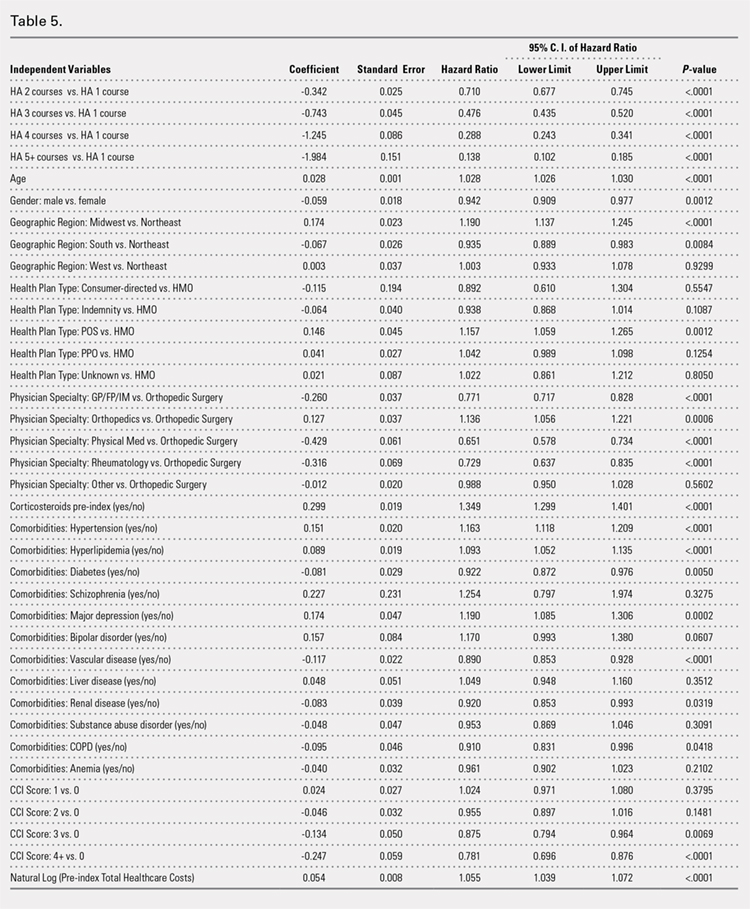
Continue to: Discussion...
DISCUSSION
This study demonstrated that multiple courses of HA treatment can delay the need for surgery for up to 3 years, with risk for both TKR and partial knee replacement decreasing in a dose-dependent manner. The potentially confounding effect of differences in baseline characteristics that could influence patients’ propensity to receive TKR in a database study was controlled by performing a multivariate analysis with covariate adjustment. The TKR-delaying effect of HA injection was more prominent in cohorts with a high number of HA treatment courses: 19 out of 20 patients in the cohort of ≥5 HA courses were free of TKR at the end of the 3-year post-index period. Such a high proportion of patients avoiding TKR with repeated courses of HA suggests that some patients may be able to successfully delay TKR well beyond the 3-year time span. This finding is counter-evidence to the frequently made assumption15 that all patients with knee OA will eventually progress to a state of disability, making TKR inevitable. The patients with end-stage radiographic knee OA can also benefit from IA HA injections for an extended period of time;16 the latest evidence indicates that nonoperative management can improve symptoms irrespective of radiographic disease severity, implying that TKR needs not to be the only therapeutic option for patients with end-stage radiographic knee OA.17 This finding suggests that HA treatment should be considered an important clinical treatment option for patients with knee OA.
Although the incidence rates of certain adverse events, such as arthralgia/joint pain, are sizable, these temporary adverse events commonly occur among patients who receive IA injections for knee OA; most of these events may simply include symptoms of the remaining underlying knee OA. These results are consistent with those of previous literature reporting the safety of repeated treatment with IA HA injections in a prospective clinical trial18 and demonstrating that repeated courses of HA treatment pose no greater safety risk than a single course of HA treatment.
Multivariate modeling outcomes of factors influencing risk of receiving TKR are broadly consistent with the generally accepted notions that different levels of disease severity and patients’ willingness to consider TKR at baseline influence the likelihood and timing of receiving TKR.19,20 Age and obesity are common risk factors for progression of OA. Orthopedic surgeons are more likely to recommend surgery than non-surgeons. The pre-index use of corticosteroids and high pre-index healthcare costs could be associated with more severe symptoms at baseline. Patients with vascular disease or severe comorbidities, as evidenced by high CCI scores, make poor candidates for major elective surgeries such as TKR. These results are intuitive and validate the clinical insights of this study. Moreover, inclusion of these covariates in the analysis model allows for indirect adjustment of the most important prognostic factors for TKR at baseline, permitting proper statistical comparison of the results for different cohort groups.
Recently, the efficacy of HA injections for OA patients has become the subject of debate when the American Academy of Orthopaedic Surgeons (AAOS) revised its clinical practice guideline, recommending against the use of HA.21 The AAOS’ findings differ from those of other clinical societies, such as the American College of Rheumatology22 and the European League Against Rheumatism,23 which provide no strong recommendation against the use of HA injections. The announcement of the new guideline by AAOS caused concern among clinicians and payers who had valued IA HA injections as a means to control knee OA pain before patients progress to TKR;24 on the other hand, the demand for nonoperative treatment of knee OA remains high. Utilization rates of TKR have increased dramatically, and surgeries are now performed on younger patients with increasing burden on the healthcare system,25,26 in spite of the fact that as high as a third of TKR surgeries may have been performed in inappropriate patients.27 Part of the confusion surrounding clinical utility of HA stems from the fact that up until recently, relatively little research looked into the practical benefits of HA in actual clinical practice. Analyses of databases such as registries are now gaining attention to overcome that problem. Examination of large administrative databases maintained by commercial payers offers the benefit of probing realistically the safety and efficacy of treatments in actual clinical environments in a very large number of patients with heterogeneous backgrounds. Recently, the Agency for Healthcare Research and Quality’s Technology Assessment Program in the US called for such studies to determine whether HA injections can delay progression to TKR.28 The results of this study and several others11,13,14,16 suggest that use of HA to treat OA of the knee is associated with the delay of TKR, supporting the utility of HA in clinical practice and the healthcare system. Potential clinical benefits of delaying TKR may include the reduced risk of aseptic loosening if younger patients can wait for TKR or more time to allow the modification of risk factors in patients who will ultimately undergo TKR.
LIMITATIONS
Follow-up period was limited to 3 years post-index date because longer follow-up data were not available at the time of the study design. If an incorrect adverse event or OA diagnosis was listed in the medical record, or if the medical record was incomplete, then patients might have been misclassified, resulting in selection bias. The claims dataset includes no uninsured and Medicare patients, as the population in the database consisted primarily of commercially-insured patients in the US. Therefore, the results are most generalizable to other commercially-insured patients in the US. Generalizability to other populations may not be assured if they differ in their accessibility to physician services or prescriptions from the patients in this study. Other treatments such as the nonsteroidal anti-inflammatory drugs used by patients were not included within the pre-specified statistical model because their potential effects were assumed to be short-lived and much less than those of corticosteroid. Including these treatments would overload the statistical model with too many covariates, leading to potential computational instability. The database used provides no information on systemic factors, including plan limits on medication use, that could affect care. Given the large and diverse nature of the healthcare plans in the database. However, these factors should not have materially affected our study results. The claims database also lacks direct indicators of OA disease severity, such as Kellgren-Lawrence scores or patient-reported outcomes, including pain and function questionnaire scores. Our multivariate analysis indirectly makes up for this deficiency by considering other baseline characteristics or clinical indicators that may be correlated with information unavailable in a claims database. Patients who opt to undergo repeated courses of HA treatment may be more inclined to avoid surgery or may naturally experience OA disease progression more slowly, making them potentially different from patients who select to undergo surgery earlier without repeated courses of HA treatment. This condition may introduce a bias that causes difficulty in proving the causality between repeated HA use and delay of TKR.
CONCLUSION
Analysis of the knee OA patient data from a real-world database showed that repeated courses of treatment with HA are safe and are associated with the delay of TKR for up to 3 years. Additional research is needed to evaluate the effects of repeated HA courses on delaying TKR beyond a 3-year period.
- Murphy L, Helmick CG. The impact of osteoarthritis in the United States: a population-health perspective. Am J Nurs. 2012;112(3 Suppl 1):S13-S19.
- Arnold W, Fullerton DS, Holder S, May CS. Viscosupplementation: managed care issues for osteoarthritis of the knee. J Manag Care Pharm. 2007;13(4 Suppl):S3-S19.
- Strand V, Conaghan PG, Lohmander LS, et al. An integrated analysis of five double-blind, randomized controlled trials evaluating the safety and efficacy of a hyaluronan product for intra-articular injection in osteoarthritis of the knee. Osteoarthritis Cartilage. 2006;14(9):859-866.
- Strand V, Baraf HS, Lavin PT, Lim S, Hosokawa H. A multicenter, randomized controlled trial comparing a single intra-articular injection of Gel-200, a new cross-linked formulation of hyaluronic acid, to phosphate buffered saline for treatment of osteoarthritis of the knee. Osteoarthritis Cartilage. 2012;20(5):350-356.
- Strand V, McIntyre LF, Beach WR, Miller LE, Block JE. Safety and efficacy of US-approved viscosupplements for knee osteoarthritis: a systematic review and meta-analysis of randomized, saline-controlled trials. J Pain Res. 2015;8:217-228.
- Bannuru RR, Schmid CH, Kent DM, Vaysbrott EE, Wong JB, McAlindon TE. Comparative effectiveness of pharmacologic interventions for knee osteoarthritis: a systematic review and network meta-analysis. Ann Intern Med. 2015;162(1):46-54.
- Mandl LA, Losina E. Relative efficacy of knee osteoarthritis treatments: are all placebos created equal? Ann Intern Med. 2015;162(1):71-72.
- Kusayama Y, Akamatsu Y, Kumagai K, Kobayashi H, Aratake M, Saito T. Changes in synovial fluid biomarkers and clinical efficacy of intra-articular injections of hyaluronic acid for patients with knee osteoarthritis. J Exp Orthop. 2014;1(1):16. doi:10.1186/s40634-014-0016-7.
- Kaneko K, Higuchi C, Kunugiza Y, et al. Hyaluronan inhibits BMP-induced osteoblast differentiation. FEBS Lett. 2015;589(4):447-454. doi:10.1016/j.febslet.2014.
- Altman RD, Manjoo A, Fierlinger A, Niazi F, Nicholls M. The mechanism of action for hyaluronic acid treatment in the osteoarthritic knee: a systematic review. BMC Musculoskelet Disord. 2015;16:321. doi:10.1186/s12891-015-0775-z.
- Waddell DD, Bricker DC. Total knee replacement delayed with hylan G-F 20 use in patients with grade IV osteoarthritis. J Manag Care Pharm. 2007;13(2):113-121.
- Khan T, Nanchanatt G, Farber K, Jan S. Analysis of the effectiveness of hyaluronic acid in prevention of total knee replacement in osteoarthritis patients. J Manag Care Pharm. 2014;20:S49.
- Abbott T, Altman RD, Dimeff R, et al. Do hyaluronic acid injections delay total knee replacement surgery? Arthritis Rheum. 2013;65(Suppl 10):2139.
- Altman R, Lim S, Steen R, Dasa V. Intra-articular hyaluronic acid delays total knee replacement in patients with knee osteoarthritis: evidence from a large U.S. health claims database. Osteoarthritis Cartilage. 2015;23(Suppl 2):A403-A404.
- Mather RC 3rd, Hug KT, Orlando LA, et al. Economic evaluation of access to musculoskeletal care: the case of waiting for total knee arthroplasty. BMC Musculoskelet Disord. 2014;15:22. doi:10.1186/1471-2474-15-22.
- Waddell DD, Joseph B. Delayed total knee replacement with Hylan G-F 20. J Knee Surg. 2016;29(2):159-168. doi:10.1055/s-0034-1395281.
- Atukorala I, Makovey J, Williams M, Ochoa Albiztegui E, Eyles JP, Hunter DJ. If you have end-stage radiographic knee osteoarthritis can you respond to non-surgical management? Osteoarthritis Cartilage. 2015;23(Suppl 2):A329.
- Strand V, Baraf HS, Lavin PT, Lim S, Hosokawa H. Effectiveness and safety of a multicenter extension and retreatment trial of Gel-200 in patients with knee osteoarthritis. Cartilage. 2012;3(4):297-304. doi:10.1177/1947603512451024.
- Riddle DL, Kong X, Jiranek WA. Two-year incidence and predictors of future knee arthroplasty in persons with symptomatic knee osteoarthritis: preliminary analysis of longitudinal data from the osteoarthritis initiative. Knee. 2009;16(6):494-500.
- Hawker GA, Guan J, Croxford R, et al. A prospective population-based study of the predictors of undergoing total joint arthroplasty. Arthritis Rheum. 2006;54(10):3212-3220.
- Jevsevar DS. Treatment of osteoarthritis of the knee: evidence-based guideline, 2nd edition. J Am Acad Orthop Surg. 2013;21(9):571-576. doi:10.5435/JAAOS-21-09-571.
- Hochberg MC, Altman RD, April KT, et al. American College of Rheumatology 2012 recommendations for the use of nonpharmacologic and pharmacologic therapies in osteoarthritis of the hand, hip, and knee. Arthritis Care Res (Hoboken). 2012;64(4):465-474.
- Jordan KM, Arden NK, Doherty M, et al. EULAR Recommendations 2003: an evidence based approach to the management of knee osteoarthritis: report of a task force of the standing committee for international clinical studies including therapeutic trials (ESCISIT). Ann Rheum Dis. 2003;62(12):1145-1155.
- Bannuru RR, Vaysbrot EE, McIntyre LF. Did the American Academy of Orthopaedic Surgeons osteoarthritis guidelines miss the mark? Arthroscopy. 2014;30(1):86-89. doi:10.1016/j.arthro.2013.10.007.
- Losina E, Thornhill TS, Rome BN, Wright J, Katz JN. The dramatic increase in total knee replacement utilization rates in the United States cannot be fully explained by growth in population size and the obesity epidemic. J Bone Joint Surg Am. 2012;94(3):201-207. doi:10.2106/JBJS.J.01958.
- Weinstein AM, Rome BN, Reichmann WM, et al. Estimating the burden of total knee replacement in the United States. J Bone Joint Surg Am. 2013;95(5):385-392. doi:10.2106/JBJS.L.00206.
- Riddle DL, Jiranek WA, Hayes CW. Use of a validated algorithm to judge the appropriateness of total knee arthroplasty in the United States: a multicenter longitudinal cohort study. Arthritis Rheumatol. 2014;66(8):2134-2143. doi:10.1002/art.38685.
- NewBerry SJ, Fitzgerald JD, Maglione MA, et al. Agency for Healthcare Research and Quality Web site. Systematic Review for Effectiveness of Hyaluronic Acid in the Treatment of Severe Degenerative Joint Disease (DJD) of the Knee: Technology Assessment Report. http://www.ahrq.gov/research/findings/ta/call-for-public-review.html. Published July 23, 2015. Accessed December 22, 2014.
- Murphy L, Helmick CG. The impact of osteoarthritis in the United States: a population-health perspective. Am J Nurs. 2012;112(3 Suppl 1):S13-S19.
- Arnold W, Fullerton DS, Holder S, May CS. Viscosupplementation: managed care issues for osteoarthritis of the knee. J Manag Care Pharm. 2007;13(4 Suppl):S3-S19.
- Strand V, Conaghan PG, Lohmander LS, et al. An integrated analysis of five double-blind, randomized controlled trials evaluating the safety and efficacy of a hyaluronan product for intra-articular injection in osteoarthritis of the knee. Osteoarthritis Cartilage. 2006;14(9):859-866.
- Strand V, Baraf HS, Lavin PT, Lim S, Hosokawa H. A multicenter, randomized controlled trial comparing a single intra-articular injection of Gel-200, a new cross-linked formulation of hyaluronic acid, to phosphate buffered saline for treatment of osteoarthritis of the knee. Osteoarthritis Cartilage. 2012;20(5):350-356.
- Strand V, McIntyre LF, Beach WR, Miller LE, Block JE. Safety and efficacy of US-approved viscosupplements for knee osteoarthritis: a systematic review and meta-analysis of randomized, saline-controlled trials. J Pain Res. 2015;8:217-228.
- Bannuru RR, Schmid CH, Kent DM, Vaysbrott EE, Wong JB, McAlindon TE. Comparative effectiveness of pharmacologic interventions for knee osteoarthritis: a systematic review and network meta-analysis. Ann Intern Med. 2015;162(1):46-54.
- Mandl LA, Losina E. Relative efficacy of knee osteoarthritis treatments: are all placebos created equal? Ann Intern Med. 2015;162(1):71-72.
- Kusayama Y, Akamatsu Y, Kumagai K, Kobayashi H, Aratake M, Saito T. Changes in synovial fluid biomarkers and clinical efficacy of intra-articular injections of hyaluronic acid for patients with knee osteoarthritis. J Exp Orthop. 2014;1(1):16. doi:10.1186/s40634-014-0016-7.
- Kaneko K, Higuchi C, Kunugiza Y, et al. Hyaluronan inhibits BMP-induced osteoblast differentiation. FEBS Lett. 2015;589(4):447-454. doi:10.1016/j.febslet.2014.
- Altman RD, Manjoo A, Fierlinger A, Niazi F, Nicholls M. The mechanism of action for hyaluronic acid treatment in the osteoarthritic knee: a systematic review. BMC Musculoskelet Disord. 2015;16:321. doi:10.1186/s12891-015-0775-z.
- Waddell DD, Bricker DC. Total knee replacement delayed with hylan G-F 20 use in patients with grade IV osteoarthritis. J Manag Care Pharm. 2007;13(2):113-121.
- Khan T, Nanchanatt G, Farber K, Jan S. Analysis of the effectiveness of hyaluronic acid in prevention of total knee replacement in osteoarthritis patients. J Manag Care Pharm. 2014;20:S49.
- Abbott T, Altman RD, Dimeff R, et al. Do hyaluronic acid injections delay total knee replacement surgery? Arthritis Rheum. 2013;65(Suppl 10):2139.
- Altman R, Lim S, Steen R, Dasa V. Intra-articular hyaluronic acid delays total knee replacement in patients with knee osteoarthritis: evidence from a large U.S. health claims database. Osteoarthritis Cartilage. 2015;23(Suppl 2):A403-A404.
- Mather RC 3rd, Hug KT, Orlando LA, et al. Economic evaluation of access to musculoskeletal care: the case of waiting for total knee arthroplasty. BMC Musculoskelet Disord. 2014;15:22. doi:10.1186/1471-2474-15-22.
- Waddell DD, Joseph B. Delayed total knee replacement with Hylan G-F 20. J Knee Surg. 2016;29(2):159-168. doi:10.1055/s-0034-1395281.
- Atukorala I, Makovey J, Williams M, Ochoa Albiztegui E, Eyles JP, Hunter DJ. If you have end-stage radiographic knee osteoarthritis can you respond to non-surgical management? Osteoarthritis Cartilage. 2015;23(Suppl 2):A329.
- Strand V, Baraf HS, Lavin PT, Lim S, Hosokawa H. Effectiveness and safety of a multicenter extension and retreatment trial of Gel-200 in patients with knee osteoarthritis. Cartilage. 2012;3(4):297-304. doi:10.1177/1947603512451024.
- Riddle DL, Kong X, Jiranek WA. Two-year incidence and predictors of future knee arthroplasty in persons with symptomatic knee osteoarthritis: preliminary analysis of longitudinal data from the osteoarthritis initiative. Knee. 2009;16(6):494-500.
- Hawker GA, Guan J, Croxford R, et al. A prospective population-based study of the predictors of undergoing total joint arthroplasty. Arthritis Rheum. 2006;54(10):3212-3220.
- Jevsevar DS. Treatment of osteoarthritis of the knee: evidence-based guideline, 2nd edition. J Am Acad Orthop Surg. 2013;21(9):571-576. doi:10.5435/JAAOS-21-09-571.
- Hochberg MC, Altman RD, April KT, et al. American College of Rheumatology 2012 recommendations for the use of nonpharmacologic and pharmacologic therapies in osteoarthritis of the hand, hip, and knee. Arthritis Care Res (Hoboken). 2012;64(4):465-474.
- Jordan KM, Arden NK, Doherty M, et al. EULAR Recommendations 2003: an evidence based approach to the management of knee osteoarthritis: report of a task force of the standing committee for international clinical studies including therapeutic trials (ESCISIT). Ann Rheum Dis. 2003;62(12):1145-1155.
- Bannuru RR, Vaysbrot EE, McIntyre LF. Did the American Academy of Orthopaedic Surgeons osteoarthritis guidelines miss the mark? Arthroscopy. 2014;30(1):86-89. doi:10.1016/j.arthro.2013.10.007.
- Losina E, Thornhill TS, Rome BN, Wright J, Katz JN. The dramatic increase in total knee replacement utilization rates in the United States cannot be fully explained by growth in population size and the obesity epidemic. J Bone Joint Surg Am. 2012;94(3):201-207. doi:10.2106/JBJS.J.01958.
- Weinstein AM, Rome BN, Reichmann WM, et al. Estimating the burden of total knee replacement in the United States. J Bone Joint Surg Am. 2013;95(5):385-392. doi:10.2106/JBJS.L.00206.
- Riddle DL, Jiranek WA, Hayes CW. Use of a validated algorithm to judge the appropriateness of total knee arthroplasty in the United States: a multicenter longitudinal cohort study. Arthritis Rheumatol. 2014;66(8):2134-2143. doi:10.1002/art.38685.
- NewBerry SJ, Fitzgerald JD, Maglione MA, et al. Agency for Healthcare Research and Quality Web site. Systematic Review for Effectiveness of Hyaluronic Acid in the Treatment of Severe Degenerative Joint Disease (DJD) of the Knee: Technology Assessment Report. http://www.ahrq.gov/research/findings/ta/call-for-public-review.html. Published July 23, 2015. Accessed December 22, 2014.
TAKE-HOME POINTS
- Repeated courses of treatment with HA are safe and are associated with the delay of TKR for up to 3 years.
- HA treatment should be considered an important clinical treatment option for patients with knee OA.
- Repeated courses of treatment with HA are safe.
- Repeated courses of HA treatment pose no greater safety risk than a single course of HA treatment.
- Additional research is needed to evaluate the effects of repeated HA courses on delaying TKR beyond a 3-year period.
Treatment of Grade III Acromioclavicular Separations in Professional Baseball Pitchers: A Survey of Major League Baseball Team Physicians
ABSTRACT
Despite advancements in surgical technique and understanding of throwing mechanics, controversy persists regarding the treatment of grade III acromioclavicular (AC) joint separations, particularly in throwing athletes. Twenty-eight major league baseball (MLB) orthopedic team physicians were surveyed to determine their definitive management of a grade III AC separation in the dominant arm of a professional baseball pitcher and their experience treating AC joint separations in starting pitchers and position players. Return-to-play outcomes were also evaluated. Twenty (71.4%) team physicians recommended nonoperative intervention compared to 8 (28.6%) who would have operated acutely. Eighteen (64.3%) team physicians had treated at least 1 professional pitcher with a grade III AC separation; 51 (77.3%) pitchers had been treated nonoperatively compared to 15 (22.7%) operatively. No difference was observed in the proportion of pitchers who returned to the same level of play (P = .54), had full, unrestricted range of motion (P = .23), or had full pain relief (P = .19) between the operatively and nonoperatively treated MLB pitchers. The majority (53.6%) of physicians would not include an injection if the injury was treated nonoperatively. Open coracoclavicular reconstruction (65.2%) was preferred for operative cases; 66.7% of surgeons would also include distal clavicle excision as an adjunct procedure. About 90% of physicians would return pitchers to throwing >12 weeks after surgery compared to after 4 to 6 weeks in nonoperatively treated cases. In conclusion, MLB team physicians preferred nonoperative management for an acute grade III AC joint separation in professional pitchers. If operative intervention is required, ligament reconstruction with adjunct distal clavicle excision were the most commonly performed procedures.
Continue to: Despite advancements in surgucal technique...
Despite advancements in surgical technique and improved understanding of the physiology of throwing mechanics, controversy persists regarding the preferred treatment for grade III acromioclavicular (AC) joint separations.1-6 Nonsurgical management has demonstrated return to prior function with fewer complications.7 However, there is a growing body of evidence demonstrating that surgical intervention is associated with more favorable outcomes8 and should be considered in patients who place high functional demands on their shoulders.9
The reported results on professional athletes in the literature remain ambivalent. Multiple small case reports/series have reported successful nonoperative treatment of elite athletes.10-12 Not surprisingly, McFarland and colleagues13 reported in 1997 that 69% of major league baseball (MLB) team physicians preferred nonoperative treatment for a theoretical starting pitcher sustaining a grade III AC separation 1 week prior to the start of the season. In contrast, reports of an inability to throw at a pre-injury level are equally commonplace.14,15 Nevertheless, all of these studies were limited to small cohorts, as the incidence of grade III AC separations in elite throwing athletes is relatively uncommon.13,16
In this study, we re-evaluated the study performed by McFarland and colleagues13 in 1997 by surveying all active MLB team orthopedic surgeons. We asked them how they would treat a grade III AC separation in a starting professional baseball pitcher. The physicians were also asked about their personal experience evaluating outcomes in these elite athletes. Given our improved understanding of the anatomy, pathophysiology, and surgical techniques for treating grade III AC separations, we hypothesize that more MLB team physicians would favor operative intervention treatment in professional baseball pitchers, as their vocation places higher demands on their shoulders.
MATERIALS AND METHODS
A questionnaire (Appendix A) was distributed to the team physicians of all 30 MLB teams. In addition to surgeon demographics, including age, years in practice, and years of taking care of an MLB team, the initial section of the questionnaire asked orthopedic surgeons how they would treat a theoretical starting pitcher who sustained a grade III AC joint separation of the dominant throwing arm 1 week prior to the start of the season. Physicians who preferred nonoperative treatment were asked whether they would use an injection (and what type), as well as when they would allow the pitcher to start a progressive interval throwing program. Physicians who preferred operative treatment were asked to rank their indications for operating, what procedure they would use (eg, open vs arthroscopic or coracoclavicular ligament repair vs reconstruction), and whether the surgical intervention would include distal clavicle excision. Both groups of physicians were also asked if their preferred treatment would change if the injury were to occur at the end of the season.
The second portion of the questionnaire asked surgeons about their experience treating AC joint separations in both starting pitchers and position players, as well as to describe the long-term outcomes of their preferred treatment, including time to return to full clearance for pitching, whether their patients returned to their prior level of play, and whether these patients had full pain relief. Finally, physicians were asked if any of the nonoperatively treated players ultimately crossed over and required operative intervention.
Continue to: Statistics...
STATISTICS
Descriptive statistics were used for continuous variables, and frequencies were used for categorical variables. Linear regression was performed to determine the correlation between the physician’s training or experience in treating AC joint separations and their recommended treatment. Fischer’s exact test/chi-square analysis was used to compare categorical variables. All tests were conducted using 2-sided hypothesis testing with statistical significance set at P < .05. All statistical analyses were conducted with SPSS 21.0 software (IBM Corporation).
RESULTS
A total of 28 MLB team physicians completed the questionnaires from 18 of the 30 MLB teams. The average age of the responders was 50.5 years (range, 34-60 years), with an average of 18.2 years in practice (range, 2-30 years) and 10.8 years (range, 1-24 years) taking care of their current professional baseball team. About 82% of the team physicians completed a sports medicine fellowship. On average, physicians saw 16.6 (range, 5-50) grade III or higher AC joint separations per year, and operated on 4.6 (range, 0-10) per year.
Nonoperative treatment was the preferred treatment for a grade III AC joint separation in a starting professional baseball pitcher for the majority of team physicians (20/28). No correlation was observed between the physician’s age (P = .881), years in practice (P = .915), years taking care of their professional team (P = .989), percentage of practice focused on shoulders (P = .986), number of AC joint injuries seen (P = .325), or number of surgeries performed per year (P = .807) with the team physician’s preferred treatment. Compared to the proportion reported originally by McFarland and colleagues13 in 1997 (69%), there was no difference in the proportion of team physicians that recommended nonoperative treatment (P = 1).
If treating this injury nonoperatively, 46.4% of physicians would also use an injection, with orthobiologics (eg, platelet-rich plasma) as the most popular choice (Table 1). No consensus was provided on the timeframe to return pitchers back to a progressive interval throwing program; however, 46.67% of physicians would return pitchers 4 to 6 weeks after a nonoperatively treated injury, while 35.7% would return pitchers 7 to 12 weeks after the initial injury.
Table 1. Treatment Preferences of Grade III AC Separation by MLB Team Physicians
Nonoperativea | |
Yes injection | 13 (46.4%) |
Cortisone | 3 (23.1%) |
Orthobiologic | 10 (76.9%) |
Local anesthetic (eg, lidocaine) | 1 (7.7%) |
Intramuscular toradol | 3 (23.1%) |
No injection | 15 (53.6%) |
Operativea | |
Open coracoclavicular ligament repair | 3 (13.0%) |
Open coracoclavicular ligament reconstruction | 15 (65.2%) |
Arthroscopic reconstruction with graft | 6 (26.1%) |
Arthroscopic repair with implant (ie, tight-rope) | 2 (8.7%) |
Distal clavicle excisionb | 16 (66.7%) |
Would not intervene operatively | 5 (17.9%) |
|
|
aRespondents were allowed to choose more than 1 treatment in each category. bChosen as an adjunct treatment.
Abbreviations: AC, acromioclavicular; MLB, major league baseball.
Most physicians (64.3%) cited functional limitations as the most important reason for indicating operative treatment, followed by pain (21.4%), and a deformity (14.3%). About 65% preferred open coracoclavicular ligament reconstruction. No physician recommended the Weaver-Dunn procedure or use of hardware (eg, hook plate). Of those who preferred an operative intervention, 66.7% would also include a distal clavicle excision, which is significantly higher than the proportion reported by McFarland and colleagues13 (23%, P = .0170). About 90% of physicians would return pitchers to play >12 weeks after operative treatment.
Continue to: If the injury occurred at the end ...
If the injury occurred at the end of the season, 7 of the 20 orthopedists (35%) who recommended nonoperative treatment said they would change to an operative intervention. Eighteen of 28 responders would have the same algorithm for MLB position players. Team physicians were less likely to recommend operative intervention in position players due to less demand on the arm and increased ability to accommodate the injury by altering their throwing mechanics.
Eighteen (64%) of the team physicians had treated at least 1 professional pitcher with a grade III AC separation in his dominant arm, and 11 (39.3%) had treated >1. Collectively, team physicians had treated 15 professional pitchers operatively, and 51 nonoperatively; only 3 patients converted to operative intervention after a failed nonoperative treatment.
Of the pitchers treated operatively, 93.3% (14) of pitchers returned to their prior level of pitching. The 1 patient who failed to return to the same level of pitching retired instead of returning to play. About 80% (12) of the pitchers had full pain relief, and 93.3% (14) had full range of motion (ROM). The pitcher who failed to regain full ROM also had a concomitant rotator cuff repair. The only complication reported from an operative intervention was a pitcher who sustained a coracoid fracture 10 months postoperatively while throwing 100 mph. Of the pitchers treated nonoperatively, 96% returned to their prior level of pitching, 92.2% (47) had full complete pain relief when throwing, and 100% had full ROM. No differences were observed between the proportion of pitchers who returned to their prior level of pitching, regained full ROM, or had full pain relief in the operative and nonoperative groups (Table 2).
Table 2. Outcomes of Treatment of Grade III AC Separation in 58 Professional Baseball Players
| Operative | Nonoperative | P-value |
Return to same level of play | 14/15 (93.3%) | 49/51 (96%) | 0.54 |
Full pain relief | 12/15 (80%) | 47/51 (92.2%) | 0.19 |
Full ROM | 14/15 (93.3%) | 51/51 (100%) | 0.23 |
Abbreviations: AC, acromioclavicular; ROM, range of motion.
DISCUSSION
Controversy persists regarding the optimal management of acute grade III AC separations, with the current available evidence potentially suggesting better cosmetic and radiological results but no definite differences in clinical results.1-6,17,18 In the absence of formal clinical practice guidelines, surgeons rely on their own experience or defer to the anecdotal experience of experts in the field. Our initial hypothesis was false in this survey of MLB team physicians taking care of overhead throwing athletes at the highest level. Our results demonstrate that despite improved techniques and an increased understanding of the pathophysiology of AC joint separations, conservative management is still the preferred treatment for acute grade III AC joint separations in professional baseball pitchers. The proportion of team physicians recommending nonoperative treatment in our series was essentially equivalent to the results reported by McFarland and colleagues13 in 1997, suggesting that the pendulum continues to favor conservative management initially. This status quo likely reflects both the dearth of literature suggesting a substantial benefit of acute operative repair, as well as the ability to accommodate with conservative measures after most grade III AC injuries, even at the highest level of athletic competition.
These results are also consistent with trends from the last few decades. In the 1970s, the overwhelming preference for treating an acute complete AC joint separation was surgical repair, with Powers and Bach10 reporting in a 1974 survey of 163 chairmen of orthopedic programs around the country that 91.5% advocated surgical treatment. However, surgical preference had reversed by the 1990s. Of the 187 chairmen and 59 team physicians surveyed by Cox19 in 1992, 72% and 86% respectively preferred nonoperative treatment in a theoretical 21-year-old athlete with a grade III AC separation. Nissen and Chatterjee20 reported in 2007 on a survey of all American Orthopaedic Society for Sports Medicine surgeons (N = 577) and Accreditation Council for Graduate Medical Education orthopedic program residency directors (N = 87) that >80% of responders preferred conservative measures for this acute injury. The reversal of trends has also been corroborated by recent multicenter trials demonstrating no difference in clinical outcomes between operative and nonoperative treatment of high grade AC joint dislocations, albeit these patients were not all high level overhead throwing athletes.17,18
Continue to: The trends in surgical interventions are notable...
The trends in surgical interventions are notable within the smaller subset of patients who are indicated for operative repair. Use of hardware and primary ligament repair, while popular in the surveys conducted in the 1970s10 and 1990s13 and even present in Nissen and Chatterjee’s20 2007 survey, were noticeably absent from our survey results, with the majority of respondents preferring open coracoclavicular ligament reconstruction. The role of distal clavicle excision has also expanded, from 23% of team physicians recommending it in 199713 to 57% to 59% in Nissen and Chatterjee’s20 2007 survey, to 66.7% in our series. This trend is not surprising as several recent cadaveric biomechanical studies have demonstrated that not only do peak graft forces not increase significantly,21 the anterior-posterior and superior-inferior motion at the AC joint following ligament reconstruction is maintained despite resection of the lateral clavicle.22 Additionally, primary distal clavicle excision may prevent the development of post-traumatic arthritis at the AC joint and osteolysis of the distal clavicle as a possible pain generator in the future.23 However, some respondents cautioned against performing a concomitant distal clavicle excision, as some biomechanical data demonstrate that resecting the distal clavicle may lead to increased horizontal translation at the AC joint despite intact superior and posterior AC capsules.24 Professional baseball pitchers may also be more lax and thus prone to more instability. Primary repair or reconstruction may not always lead to complete pre-injury stability in these individuals. This subtle unrecognized instability is hard to diagnosis and may be a persistent source of pain; thus, adding a distal clavicle excision may actually exacerbate the instability.
The nuanced indications for operative intervention, such as the presence of associated lesions were not captured by our survey.25 While most team physicians cited functional limitations as their most common reason for offering surgery, several MLB orthopedic surgeons also commented on evaluating the stability of the AC joint after a grade III injury, akin to the consensus statement from the International Society of Arthroscopy, Knee Surgery and Orthopaedic Sports Medicine (ISAKOS) Upper Extremity Committee26 in 2014 that diversified the Rockwood Grade III AC joint separation into its IIIA and IIIB classifications. The ISAKOS recommendations include initial conservative management and a second evaluation (both clinical and radiographic) for grade III lesions 3 to 6 weeks after the injury. However, as professional baseball is an incredibly profitable sport with an annual revenue approaching $9.5 billion27 and pitching salaries up to $32.5 million in 2015, serious financial considerations must be given to players who wish to avoid undergoing delayed surgery.
This study has shortcomings typical of expert opinion papers. The retrospective nature of this study places the data at risk of recall bias. Objective data (eg, terminal ROM, pain relief, and return to play) were obtained from a retrospective chart review; however, no standard documentation or collection method was used given the number of surgeons involved and, thus, conclusions based on treatment outcomes are imperfect. Another major weakness of this survey is the relatively small number of patients and respondents. An a priori power analysis was not available, as this was a retrospective review. A comparative trial will be necessary to definitively support one treatment over another. Assuming a 95% return to play in the nonoperatively treated group, approximately 300 patients would be needed in a prospective 2-armed study with 80% power to detect a 10% reduction in the incidence of return to play using an alpha level of 0.05 and assuming no loss to follow-up. This sample size would be difficult to achieve in this patient population.
However, compared to past series,13 the number of professional baseball players treated by the collective experience of these MLB team physicians is the largest reported to date. As suggested above, the rarity of this condition in elite athletes precludes the ability to have matched controls to definitively determine the optimal treatment, which may explain the lack of difference in the return to play, ROM, and pain relief outcomes. Instead, we can only extrapolate based on the collective anecdotal experience of the MLB team physicians.
CONCLUSION
Despite advances in surgical technique and understanding of throwing mechanics, the majority of MLB team physicians preferred nonoperative management for an acute grade III AC joint separation in a professional baseball pitcher. Open coracoclavicular ligament reconstruction was preferred for those who preferred operative intervention. An increasing number of orthopedic surgeons now consider a distal clavicle excision as an adjunct procedure.
This paper will be judged for the Resident Writer’s Award.
- Spencer EE Jr. Treatment of grade III acromioclavicular joint injuries: a systematic review. Clin Orthop Relat Res. 2007;455:38-44. doi:10.1097/BLO.0b013e318030df83.
- Ceccarelli E, Bondì R, Alviti F, Garofalo R, Miulli F, Padua R. Treatment of acute grade III acromioclavicular dislocation: A lack of evidence. J Orthop Traumatol. 2008;9(2):105-108. doi:10.1007/s10195-008-0013-7.
- Smith TO, Chester R, Pearse EO, Hing CB. Operative versus non-operative management following rockwood grade III acromioclavicular separation: a meta-analysis of the current evidence base. J Orthop Traumatol. 2011;12(1):19-27. doi:10.1007/s10195-011-0127-1.
- Beitzel K, Cote MP, Apostolakos J, et al. Current concepts in the treatment of acromioclavicular joint dislocations. Arthroscopy. 2013;29(2):387-397. doi:10.1016/j.arthro.2012.11.023.
- Korsten K, Gunning AC, Leenen LP. Operative or conservative treatment in patients with rockwood type III acromioclavicular dislocation: a systematic review and update of current literature. Int Orthop. 2014;38(4):831-838. doi:10.1007/s00264-013-2143-7.
- Modi CS, Beazley J, Zywiel MG, Lawrence TM, Veillette CJ. Controversies relating to the management of acromioclavicular joint dislocations. Bone Joint J. 2013;95-B(12):1595-1602. doi:10.1302/0301-620X.95B12.31802.
- Reid D, Polson K, Johnson L. Acromioclavicular joint separations grades I-III: a review of the literature and development of best practice guidelines. Sports Med. 2012;42(8):681-696. doi:10.2165/11633460-000000000-00000.
- Farber AJ, Cascio BM, Wilckens JH. Type III acromioclavicular separation: rationale for anatomical reconstruction. Am J Orthop. 2008;37(7):349-355.
- Li X, Ma R, Bedi A, Dines DM, Altchek DW, Dines JS. Management of acromioclavicular joint injuries. J Bone Joint Surg Am. 2014;96(1):73-84. doi:10.2106/JBJS.L.00734.
- Powers JA, Bach PJ. Acromioclavicular separations. Closed or open treatment? Clin Orthop Relat Res. 1974;104(104):213-223. doi:10.1097/00003086-197410000-00024.
- Glick JM, Milburn LJ, Haggerty JF, Nishimoto D. Dislocated acromioclavicular joint: follow-up study of 35 unreduced acromioclavicular dislocations. Am J Sports Med. 1977;5(6):264-270. doi:10.1177/036354657700500614.
- Watson ST, Wyland DJ. Return to play after nonoperative management for a severe type III acromioclavicular separation in the throwing shoulder of a collegiate pitcher. Phys Sportsmed. 2015;43(1):99-103. doi:10.1080/00913847.2015.1001937.
- McFarland EG, Blivin SJ, Doehring CB, Curl LA, Silberstein C. Treatment of grade III acromioclavicular separations in professional throwing athletes: results of a survey. Am J Orthop. 1997;26(11):771-774.
- Wojtys EM, Nelson G. Conservative treatment of grade III acromioclavicular dislocations. Clin Orthop Relat Res. 1991;268(268):112-119.
- Galpin RD, Hawkins RJ, Grainger RW. A comparative analysis of operative versus nonoperative treatment of grade III acromioclavicular separations. Clin Orthop Relat Res. 1985;193(193):150-155. doi:10.1097/00003086-198503000-00020.
- Pallis M, Cameron KL, Svoboda SJ, Owens BD. Epidemiology of acromioclavicular joint injury in young athletes. Am J Sports Med. 2012;40(9):2072-2077. doi:10.1177/0363546512450162.
- Canadian Orthopaedic Trauma Society. Multicenter randomized clinical trial of nonoperative versus operative treatment of acute acromio-clavicular joint dislocation. J Orthop Trauma. 2015;29(11):479-487. doi:10.1097/BOT.0000000000000437.
- Joukainen A, Kröger H, Niemitukia L, Mäkelä EA, Väätäinen U. Results of operative and nonoperative treatment of rockwood types III and V acromioclavicular joint dislocation: a prospective, randomized trial with an 18- to 20-year follow-up. Orthop J Sports Med. 2014;2(12):2325967114560130. doi:10.1177/2325967114560130.
- Cox JS. Current method of treatment of acromioclavicular joint dislocations. Orthopedics. 1992;15(9):1041-1044.
- Nissen CW, Chatterjee A. Type III acromioclavicular separation: results of a recent survey on its management. Am J Orthop. 2007;36(2):89-93.
- Kowalsky MS, Kremenic IJ, Orishimo KF, McHugh MP, Nicholas SJ, Lee SJ. The effect of distal clavicle excision on in situ graft forces in coracoclavicular ligament reconstruction. Am J Sports Med. 2010;38(11):2313-2319. doi:10.1177/0363546510374447.
- Beaver AB, Parks BG, Hinton RY. Biomechanical analysis of distal clavicle excision with acromioclavicular joint reconstruction. Am J Sports Med. 2013;41(7):1684-1688. doi:10.1177/0363546513488750.
- Mumford EB. Acromioclavicular dislocation. J Bone Joint Surg Am. 1941;23:799-802.
- Beitzel K, Sablan N, Chowaniec DM, et al. Sequential resection of the distal clavicle and its effects on horizontal acromioclavicular joint translation. Am J Sports Med. 2012;40(3):681-685. doi:10.1177/0363546511428880.
- Arrigoni P, Brady PC, Zottarelli L, et al. Associated lesions requiring additional surgical treatment in grade 3 acromioclavicular joint dislocations. Arthroscopy. 2014;30(1):6-10. doi:10.1016/j.arthro.2013.10.006.
- Beitzel K, Mazzocca AD, Bak K, et al. ISAKOS upper extremity committee consensus statement on the need for diversification of the rockwood classification for acromioclavicular joint injuries. Arthroscopy. 2014;30(2):271-278. doi:10.1016/j.arthro.2013.11.005.
- Brown M. MLB sees record revenues for 2015, up $500 million and approaching $9.5 billion. Forbes Web site. http://www.forbes.com/sites/maurybrown/2015/12/04/mlb-sees-record-revenu.... Published December 4, 2015. Accessed February 4, 2016.
ABSTRACT
Despite advancements in surgical technique and understanding of throwing mechanics, controversy persists regarding the treatment of grade III acromioclavicular (AC) joint separations, particularly in throwing athletes. Twenty-eight major league baseball (MLB) orthopedic team physicians were surveyed to determine their definitive management of a grade III AC separation in the dominant arm of a professional baseball pitcher and their experience treating AC joint separations in starting pitchers and position players. Return-to-play outcomes were also evaluated. Twenty (71.4%) team physicians recommended nonoperative intervention compared to 8 (28.6%) who would have operated acutely. Eighteen (64.3%) team physicians had treated at least 1 professional pitcher with a grade III AC separation; 51 (77.3%) pitchers had been treated nonoperatively compared to 15 (22.7%) operatively. No difference was observed in the proportion of pitchers who returned to the same level of play (P = .54), had full, unrestricted range of motion (P = .23), or had full pain relief (P = .19) between the operatively and nonoperatively treated MLB pitchers. The majority (53.6%) of physicians would not include an injection if the injury was treated nonoperatively. Open coracoclavicular reconstruction (65.2%) was preferred for operative cases; 66.7% of surgeons would also include distal clavicle excision as an adjunct procedure. About 90% of physicians would return pitchers to throwing >12 weeks after surgery compared to after 4 to 6 weeks in nonoperatively treated cases. In conclusion, MLB team physicians preferred nonoperative management for an acute grade III AC joint separation in professional pitchers. If operative intervention is required, ligament reconstruction with adjunct distal clavicle excision were the most commonly performed procedures.
Continue to: Despite advancements in surgucal technique...
Despite advancements in surgical technique and improved understanding of the physiology of throwing mechanics, controversy persists regarding the preferred treatment for grade III acromioclavicular (AC) joint separations.1-6 Nonsurgical management has demonstrated return to prior function with fewer complications.7 However, there is a growing body of evidence demonstrating that surgical intervention is associated with more favorable outcomes8 and should be considered in patients who place high functional demands on their shoulders.9
The reported results on professional athletes in the literature remain ambivalent. Multiple small case reports/series have reported successful nonoperative treatment of elite athletes.10-12 Not surprisingly, McFarland and colleagues13 reported in 1997 that 69% of major league baseball (MLB) team physicians preferred nonoperative treatment for a theoretical starting pitcher sustaining a grade III AC separation 1 week prior to the start of the season. In contrast, reports of an inability to throw at a pre-injury level are equally commonplace.14,15 Nevertheless, all of these studies were limited to small cohorts, as the incidence of grade III AC separations in elite throwing athletes is relatively uncommon.13,16
In this study, we re-evaluated the study performed by McFarland and colleagues13 in 1997 by surveying all active MLB team orthopedic surgeons. We asked them how they would treat a grade III AC separation in a starting professional baseball pitcher. The physicians were also asked about their personal experience evaluating outcomes in these elite athletes. Given our improved understanding of the anatomy, pathophysiology, and surgical techniques for treating grade III AC separations, we hypothesize that more MLB team physicians would favor operative intervention treatment in professional baseball pitchers, as their vocation places higher demands on their shoulders.
MATERIALS AND METHODS
A questionnaire (Appendix A) was distributed to the team physicians of all 30 MLB teams. In addition to surgeon demographics, including age, years in practice, and years of taking care of an MLB team, the initial section of the questionnaire asked orthopedic surgeons how they would treat a theoretical starting pitcher who sustained a grade III AC joint separation of the dominant throwing arm 1 week prior to the start of the season. Physicians who preferred nonoperative treatment were asked whether they would use an injection (and what type), as well as when they would allow the pitcher to start a progressive interval throwing program. Physicians who preferred operative treatment were asked to rank their indications for operating, what procedure they would use (eg, open vs arthroscopic or coracoclavicular ligament repair vs reconstruction), and whether the surgical intervention would include distal clavicle excision. Both groups of physicians were also asked if their preferred treatment would change if the injury were to occur at the end of the season.
The second portion of the questionnaire asked surgeons about their experience treating AC joint separations in both starting pitchers and position players, as well as to describe the long-term outcomes of their preferred treatment, including time to return to full clearance for pitching, whether their patients returned to their prior level of play, and whether these patients had full pain relief. Finally, physicians were asked if any of the nonoperatively treated players ultimately crossed over and required operative intervention.
Continue to: Statistics...
STATISTICS
Descriptive statistics were used for continuous variables, and frequencies were used for categorical variables. Linear regression was performed to determine the correlation between the physician’s training or experience in treating AC joint separations and their recommended treatment. Fischer’s exact test/chi-square analysis was used to compare categorical variables. All tests were conducted using 2-sided hypothesis testing with statistical significance set at P < .05. All statistical analyses were conducted with SPSS 21.0 software (IBM Corporation).
RESULTS
A total of 28 MLB team physicians completed the questionnaires from 18 of the 30 MLB teams. The average age of the responders was 50.5 years (range, 34-60 years), with an average of 18.2 years in practice (range, 2-30 years) and 10.8 years (range, 1-24 years) taking care of their current professional baseball team. About 82% of the team physicians completed a sports medicine fellowship. On average, physicians saw 16.6 (range, 5-50) grade III or higher AC joint separations per year, and operated on 4.6 (range, 0-10) per year.
Nonoperative treatment was the preferred treatment for a grade III AC joint separation in a starting professional baseball pitcher for the majority of team physicians (20/28). No correlation was observed between the physician’s age (P = .881), years in practice (P = .915), years taking care of their professional team (P = .989), percentage of practice focused on shoulders (P = .986), number of AC joint injuries seen (P = .325), or number of surgeries performed per year (P = .807) with the team physician’s preferred treatment. Compared to the proportion reported originally by McFarland and colleagues13 in 1997 (69%), there was no difference in the proportion of team physicians that recommended nonoperative treatment (P = 1).
If treating this injury nonoperatively, 46.4% of physicians would also use an injection, with orthobiologics (eg, platelet-rich plasma) as the most popular choice (Table 1). No consensus was provided on the timeframe to return pitchers back to a progressive interval throwing program; however, 46.67% of physicians would return pitchers 4 to 6 weeks after a nonoperatively treated injury, while 35.7% would return pitchers 7 to 12 weeks after the initial injury.
Table 1. Treatment Preferences of Grade III AC Separation by MLB Team Physicians
Nonoperativea | |
Yes injection | 13 (46.4%) |
Cortisone | 3 (23.1%) |
Orthobiologic | 10 (76.9%) |
Local anesthetic (eg, lidocaine) | 1 (7.7%) |
Intramuscular toradol | 3 (23.1%) |
No injection | 15 (53.6%) |
Operativea | |
Open coracoclavicular ligament repair | 3 (13.0%) |
Open coracoclavicular ligament reconstruction | 15 (65.2%) |
Arthroscopic reconstruction with graft | 6 (26.1%) |
Arthroscopic repair with implant (ie, tight-rope) | 2 (8.7%) |
Distal clavicle excisionb | 16 (66.7%) |
Would not intervene operatively | 5 (17.9%) |
|
|
aRespondents were allowed to choose more than 1 treatment in each category. bChosen as an adjunct treatment.
Abbreviations: AC, acromioclavicular; MLB, major league baseball.
Most physicians (64.3%) cited functional limitations as the most important reason for indicating operative treatment, followed by pain (21.4%), and a deformity (14.3%). About 65% preferred open coracoclavicular ligament reconstruction. No physician recommended the Weaver-Dunn procedure or use of hardware (eg, hook plate). Of those who preferred an operative intervention, 66.7% would also include a distal clavicle excision, which is significantly higher than the proportion reported by McFarland and colleagues13 (23%, P = .0170). About 90% of physicians would return pitchers to play >12 weeks after operative treatment.
Continue to: If the injury occurred at the end ...
If the injury occurred at the end of the season, 7 of the 20 orthopedists (35%) who recommended nonoperative treatment said they would change to an operative intervention. Eighteen of 28 responders would have the same algorithm for MLB position players. Team physicians were less likely to recommend operative intervention in position players due to less demand on the arm and increased ability to accommodate the injury by altering their throwing mechanics.
Eighteen (64%) of the team physicians had treated at least 1 professional pitcher with a grade III AC separation in his dominant arm, and 11 (39.3%) had treated >1. Collectively, team physicians had treated 15 professional pitchers operatively, and 51 nonoperatively; only 3 patients converted to operative intervention after a failed nonoperative treatment.
Of the pitchers treated operatively, 93.3% (14) of pitchers returned to their prior level of pitching. The 1 patient who failed to return to the same level of pitching retired instead of returning to play. About 80% (12) of the pitchers had full pain relief, and 93.3% (14) had full range of motion (ROM). The pitcher who failed to regain full ROM also had a concomitant rotator cuff repair. The only complication reported from an operative intervention was a pitcher who sustained a coracoid fracture 10 months postoperatively while throwing 100 mph. Of the pitchers treated nonoperatively, 96% returned to their prior level of pitching, 92.2% (47) had full complete pain relief when throwing, and 100% had full ROM. No differences were observed between the proportion of pitchers who returned to their prior level of pitching, regained full ROM, or had full pain relief in the operative and nonoperative groups (Table 2).
Table 2. Outcomes of Treatment of Grade III AC Separation in 58 Professional Baseball Players
| Operative | Nonoperative | P-value |
Return to same level of play | 14/15 (93.3%) | 49/51 (96%) | 0.54 |
Full pain relief | 12/15 (80%) | 47/51 (92.2%) | 0.19 |
Full ROM | 14/15 (93.3%) | 51/51 (100%) | 0.23 |
Abbreviations: AC, acromioclavicular; ROM, range of motion.
DISCUSSION
Controversy persists regarding the optimal management of acute grade III AC separations, with the current available evidence potentially suggesting better cosmetic and radiological results but no definite differences in clinical results.1-6,17,18 In the absence of formal clinical practice guidelines, surgeons rely on their own experience or defer to the anecdotal experience of experts in the field. Our initial hypothesis was false in this survey of MLB team physicians taking care of overhead throwing athletes at the highest level. Our results demonstrate that despite improved techniques and an increased understanding of the pathophysiology of AC joint separations, conservative management is still the preferred treatment for acute grade III AC joint separations in professional baseball pitchers. The proportion of team physicians recommending nonoperative treatment in our series was essentially equivalent to the results reported by McFarland and colleagues13 in 1997, suggesting that the pendulum continues to favor conservative management initially. This status quo likely reflects both the dearth of literature suggesting a substantial benefit of acute operative repair, as well as the ability to accommodate with conservative measures after most grade III AC injuries, even at the highest level of athletic competition.
These results are also consistent with trends from the last few decades. In the 1970s, the overwhelming preference for treating an acute complete AC joint separation was surgical repair, with Powers and Bach10 reporting in a 1974 survey of 163 chairmen of orthopedic programs around the country that 91.5% advocated surgical treatment. However, surgical preference had reversed by the 1990s. Of the 187 chairmen and 59 team physicians surveyed by Cox19 in 1992, 72% and 86% respectively preferred nonoperative treatment in a theoretical 21-year-old athlete with a grade III AC separation. Nissen and Chatterjee20 reported in 2007 on a survey of all American Orthopaedic Society for Sports Medicine surgeons (N = 577) and Accreditation Council for Graduate Medical Education orthopedic program residency directors (N = 87) that >80% of responders preferred conservative measures for this acute injury. The reversal of trends has also been corroborated by recent multicenter trials demonstrating no difference in clinical outcomes between operative and nonoperative treatment of high grade AC joint dislocations, albeit these patients were not all high level overhead throwing athletes.17,18
Continue to: The trends in surgical interventions are notable...
The trends in surgical interventions are notable within the smaller subset of patients who are indicated for operative repair. Use of hardware and primary ligament repair, while popular in the surveys conducted in the 1970s10 and 1990s13 and even present in Nissen and Chatterjee’s20 2007 survey, were noticeably absent from our survey results, with the majority of respondents preferring open coracoclavicular ligament reconstruction. The role of distal clavicle excision has also expanded, from 23% of team physicians recommending it in 199713 to 57% to 59% in Nissen and Chatterjee’s20 2007 survey, to 66.7% in our series. This trend is not surprising as several recent cadaveric biomechanical studies have demonstrated that not only do peak graft forces not increase significantly,21 the anterior-posterior and superior-inferior motion at the AC joint following ligament reconstruction is maintained despite resection of the lateral clavicle.22 Additionally, primary distal clavicle excision may prevent the development of post-traumatic arthritis at the AC joint and osteolysis of the distal clavicle as a possible pain generator in the future.23 However, some respondents cautioned against performing a concomitant distal clavicle excision, as some biomechanical data demonstrate that resecting the distal clavicle may lead to increased horizontal translation at the AC joint despite intact superior and posterior AC capsules.24 Professional baseball pitchers may also be more lax and thus prone to more instability. Primary repair or reconstruction may not always lead to complete pre-injury stability in these individuals. This subtle unrecognized instability is hard to diagnosis and may be a persistent source of pain; thus, adding a distal clavicle excision may actually exacerbate the instability.
The nuanced indications for operative intervention, such as the presence of associated lesions were not captured by our survey.25 While most team physicians cited functional limitations as their most common reason for offering surgery, several MLB orthopedic surgeons also commented on evaluating the stability of the AC joint after a grade III injury, akin to the consensus statement from the International Society of Arthroscopy, Knee Surgery and Orthopaedic Sports Medicine (ISAKOS) Upper Extremity Committee26 in 2014 that diversified the Rockwood Grade III AC joint separation into its IIIA and IIIB classifications. The ISAKOS recommendations include initial conservative management and a second evaluation (both clinical and radiographic) for grade III lesions 3 to 6 weeks after the injury. However, as professional baseball is an incredibly profitable sport with an annual revenue approaching $9.5 billion27 and pitching salaries up to $32.5 million in 2015, serious financial considerations must be given to players who wish to avoid undergoing delayed surgery.
This study has shortcomings typical of expert opinion papers. The retrospective nature of this study places the data at risk of recall bias. Objective data (eg, terminal ROM, pain relief, and return to play) were obtained from a retrospective chart review; however, no standard documentation or collection method was used given the number of surgeons involved and, thus, conclusions based on treatment outcomes are imperfect. Another major weakness of this survey is the relatively small number of patients and respondents. An a priori power analysis was not available, as this was a retrospective review. A comparative trial will be necessary to definitively support one treatment over another. Assuming a 95% return to play in the nonoperatively treated group, approximately 300 patients would be needed in a prospective 2-armed study with 80% power to detect a 10% reduction in the incidence of return to play using an alpha level of 0.05 and assuming no loss to follow-up. This sample size would be difficult to achieve in this patient population.
However, compared to past series,13 the number of professional baseball players treated by the collective experience of these MLB team physicians is the largest reported to date. As suggested above, the rarity of this condition in elite athletes precludes the ability to have matched controls to definitively determine the optimal treatment, which may explain the lack of difference in the return to play, ROM, and pain relief outcomes. Instead, we can only extrapolate based on the collective anecdotal experience of the MLB team physicians.
CONCLUSION
Despite advances in surgical technique and understanding of throwing mechanics, the majority of MLB team physicians preferred nonoperative management for an acute grade III AC joint separation in a professional baseball pitcher. Open coracoclavicular ligament reconstruction was preferred for those who preferred operative intervention. An increasing number of orthopedic surgeons now consider a distal clavicle excision as an adjunct procedure.
This paper will be judged for the Resident Writer’s Award.
ABSTRACT
Despite advancements in surgical technique and understanding of throwing mechanics, controversy persists regarding the treatment of grade III acromioclavicular (AC) joint separations, particularly in throwing athletes. Twenty-eight major league baseball (MLB) orthopedic team physicians were surveyed to determine their definitive management of a grade III AC separation in the dominant arm of a professional baseball pitcher and their experience treating AC joint separations in starting pitchers and position players. Return-to-play outcomes were also evaluated. Twenty (71.4%) team physicians recommended nonoperative intervention compared to 8 (28.6%) who would have operated acutely. Eighteen (64.3%) team physicians had treated at least 1 professional pitcher with a grade III AC separation; 51 (77.3%) pitchers had been treated nonoperatively compared to 15 (22.7%) operatively. No difference was observed in the proportion of pitchers who returned to the same level of play (P = .54), had full, unrestricted range of motion (P = .23), or had full pain relief (P = .19) between the operatively and nonoperatively treated MLB pitchers. The majority (53.6%) of physicians would not include an injection if the injury was treated nonoperatively. Open coracoclavicular reconstruction (65.2%) was preferred for operative cases; 66.7% of surgeons would also include distal clavicle excision as an adjunct procedure. About 90% of physicians would return pitchers to throwing >12 weeks after surgery compared to after 4 to 6 weeks in nonoperatively treated cases. In conclusion, MLB team physicians preferred nonoperative management for an acute grade III AC joint separation in professional pitchers. If operative intervention is required, ligament reconstruction with adjunct distal clavicle excision were the most commonly performed procedures.
Continue to: Despite advancements in surgucal technique...
Despite advancements in surgical technique and improved understanding of the physiology of throwing mechanics, controversy persists regarding the preferred treatment for grade III acromioclavicular (AC) joint separations.1-6 Nonsurgical management has demonstrated return to prior function with fewer complications.7 However, there is a growing body of evidence demonstrating that surgical intervention is associated with more favorable outcomes8 and should be considered in patients who place high functional demands on their shoulders.9
The reported results on professional athletes in the literature remain ambivalent. Multiple small case reports/series have reported successful nonoperative treatment of elite athletes.10-12 Not surprisingly, McFarland and colleagues13 reported in 1997 that 69% of major league baseball (MLB) team physicians preferred nonoperative treatment for a theoretical starting pitcher sustaining a grade III AC separation 1 week prior to the start of the season. In contrast, reports of an inability to throw at a pre-injury level are equally commonplace.14,15 Nevertheless, all of these studies were limited to small cohorts, as the incidence of grade III AC separations in elite throwing athletes is relatively uncommon.13,16
In this study, we re-evaluated the study performed by McFarland and colleagues13 in 1997 by surveying all active MLB team orthopedic surgeons. We asked them how they would treat a grade III AC separation in a starting professional baseball pitcher. The physicians were also asked about their personal experience evaluating outcomes in these elite athletes. Given our improved understanding of the anatomy, pathophysiology, and surgical techniques for treating grade III AC separations, we hypothesize that more MLB team physicians would favor operative intervention treatment in professional baseball pitchers, as their vocation places higher demands on their shoulders.
MATERIALS AND METHODS
A questionnaire (Appendix A) was distributed to the team physicians of all 30 MLB teams. In addition to surgeon demographics, including age, years in practice, and years of taking care of an MLB team, the initial section of the questionnaire asked orthopedic surgeons how they would treat a theoretical starting pitcher who sustained a grade III AC joint separation of the dominant throwing arm 1 week prior to the start of the season. Physicians who preferred nonoperative treatment were asked whether they would use an injection (and what type), as well as when they would allow the pitcher to start a progressive interval throwing program. Physicians who preferred operative treatment were asked to rank their indications for operating, what procedure they would use (eg, open vs arthroscopic or coracoclavicular ligament repair vs reconstruction), and whether the surgical intervention would include distal clavicle excision. Both groups of physicians were also asked if their preferred treatment would change if the injury were to occur at the end of the season.
The second portion of the questionnaire asked surgeons about their experience treating AC joint separations in both starting pitchers and position players, as well as to describe the long-term outcomes of their preferred treatment, including time to return to full clearance for pitching, whether their patients returned to their prior level of play, and whether these patients had full pain relief. Finally, physicians were asked if any of the nonoperatively treated players ultimately crossed over and required operative intervention.
Continue to: Statistics...
STATISTICS
Descriptive statistics were used for continuous variables, and frequencies were used for categorical variables. Linear regression was performed to determine the correlation between the physician’s training or experience in treating AC joint separations and their recommended treatment. Fischer’s exact test/chi-square analysis was used to compare categorical variables. All tests were conducted using 2-sided hypothesis testing with statistical significance set at P < .05. All statistical analyses were conducted with SPSS 21.0 software (IBM Corporation).
RESULTS
A total of 28 MLB team physicians completed the questionnaires from 18 of the 30 MLB teams. The average age of the responders was 50.5 years (range, 34-60 years), with an average of 18.2 years in practice (range, 2-30 years) and 10.8 years (range, 1-24 years) taking care of their current professional baseball team. About 82% of the team physicians completed a sports medicine fellowship. On average, physicians saw 16.6 (range, 5-50) grade III or higher AC joint separations per year, and operated on 4.6 (range, 0-10) per year.
Nonoperative treatment was the preferred treatment for a grade III AC joint separation in a starting professional baseball pitcher for the majority of team physicians (20/28). No correlation was observed between the physician’s age (P = .881), years in practice (P = .915), years taking care of their professional team (P = .989), percentage of practice focused on shoulders (P = .986), number of AC joint injuries seen (P = .325), or number of surgeries performed per year (P = .807) with the team physician’s preferred treatment. Compared to the proportion reported originally by McFarland and colleagues13 in 1997 (69%), there was no difference in the proportion of team physicians that recommended nonoperative treatment (P = 1).
If treating this injury nonoperatively, 46.4% of physicians would also use an injection, with orthobiologics (eg, platelet-rich plasma) as the most popular choice (Table 1). No consensus was provided on the timeframe to return pitchers back to a progressive interval throwing program; however, 46.67% of physicians would return pitchers 4 to 6 weeks after a nonoperatively treated injury, while 35.7% would return pitchers 7 to 12 weeks after the initial injury.
Table 1. Treatment Preferences of Grade III AC Separation by MLB Team Physicians
Nonoperativea | |
Yes injection | 13 (46.4%) |
Cortisone | 3 (23.1%) |
Orthobiologic | 10 (76.9%) |
Local anesthetic (eg, lidocaine) | 1 (7.7%) |
Intramuscular toradol | 3 (23.1%) |
No injection | 15 (53.6%) |
Operativea | |
Open coracoclavicular ligament repair | 3 (13.0%) |
Open coracoclavicular ligament reconstruction | 15 (65.2%) |
Arthroscopic reconstruction with graft | 6 (26.1%) |
Arthroscopic repair with implant (ie, tight-rope) | 2 (8.7%) |
Distal clavicle excisionb | 16 (66.7%) |
Would not intervene operatively | 5 (17.9%) |
|
|
aRespondents were allowed to choose more than 1 treatment in each category. bChosen as an adjunct treatment.
Abbreviations: AC, acromioclavicular; MLB, major league baseball.
Most physicians (64.3%) cited functional limitations as the most important reason for indicating operative treatment, followed by pain (21.4%), and a deformity (14.3%). About 65% preferred open coracoclavicular ligament reconstruction. No physician recommended the Weaver-Dunn procedure or use of hardware (eg, hook plate). Of those who preferred an operative intervention, 66.7% would also include a distal clavicle excision, which is significantly higher than the proportion reported by McFarland and colleagues13 (23%, P = .0170). About 90% of physicians would return pitchers to play >12 weeks after operative treatment.
Continue to: If the injury occurred at the end ...
If the injury occurred at the end of the season, 7 of the 20 orthopedists (35%) who recommended nonoperative treatment said they would change to an operative intervention. Eighteen of 28 responders would have the same algorithm for MLB position players. Team physicians were less likely to recommend operative intervention in position players due to less demand on the arm and increased ability to accommodate the injury by altering their throwing mechanics.
Eighteen (64%) of the team physicians had treated at least 1 professional pitcher with a grade III AC separation in his dominant arm, and 11 (39.3%) had treated >1. Collectively, team physicians had treated 15 professional pitchers operatively, and 51 nonoperatively; only 3 patients converted to operative intervention after a failed nonoperative treatment.
Of the pitchers treated operatively, 93.3% (14) of pitchers returned to their prior level of pitching. The 1 patient who failed to return to the same level of pitching retired instead of returning to play. About 80% (12) of the pitchers had full pain relief, and 93.3% (14) had full range of motion (ROM). The pitcher who failed to regain full ROM also had a concomitant rotator cuff repair. The only complication reported from an operative intervention was a pitcher who sustained a coracoid fracture 10 months postoperatively while throwing 100 mph. Of the pitchers treated nonoperatively, 96% returned to their prior level of pitching, 92.2% (47) had full complete pain relief when throwing, and 100% had full ROM. No differences were observed between the proportion of pitchers who returned to their prior level of pitching, regained full ROM, or had full pain relief in the operative and nonoperative groups (Table 2).
Table 2. Outcomes of Treatment of Grade III AC Separation in 58 Professional Baseball Players
| Operative | Nonoperative | P-value |
Return to same level of play | 14/15 (93.3%) | 49/51 (96%) | 0.54 |
Full pain relief | 12/15 (80%) | 47/51 (92.2%) | 0.19 |
Full ROM | 14/15 (93.3%) | 51/51 (100%) | 0.23 |
Abbreviations: AC, acromioclavicular; ROM, range of motion.
DISCUSSION
Controversy persists regarding the optimal management of acute grade III AC separations, with the current available evidence potentially suggesting better cosmetic and radiological results but no definite differences in clinical results.1-6,17,18 In the absence of formal clinical practice guidelines, surgeons rely on their own experience or defer to the anecdotal experience of experts in the field. Our initial hypothesis was false in this survey of MLB team physicians taking care of overhead throwing athletes at the highest level. Our results demonstrate that despite improved techniques and an increased understanding of the pathophysiology of AC joint separations, conservative management is still the preferred treatment for acute grade III AC joint separations in professional baseball pitchers. The proportion of team physicians recommending nonoperative treatment in our series was essentially equivalent to the results reported by McFarland and colleagues13 in 1997, suggesting that the pendulum continues to favor conservative management initially. This status quo likely reflects both the dearth of literature suggesting a substantial benefit of acute operative repair, as well as the ability to accommodate with conservative measures after most grade III AC injuries, even at the highest level of athletic competition.
These results are also consistent with trends from the last few decades. In the 1970s, the overwhelming preference for treating an acute complete AC joint separation was surgical repair, with Powers and Bach10 reporting in a 1974 survey of 163 chairmen of orthopedic programs around the country that 91.5% advocated surgical treatment. However, surgical preference had reversed by the 1990s. Of the 187 chairmen and 59 team physicians surveyed by Cox19 in 1992, 72% and 86% respectively preferred nonoperative treatment in a theoretical 21-year-old athlete with a grade III AC separation. Nissen and Chatterjee20 reported in 2007 on a survey of all American Orthopaedic Society for Sports Medicine surgeons (N = 577) and Accreditation Council for Graduate Medical Education orthopedic program residency directors (N = 87) that >80% of responders preferred conservative measures for this acute injury. The reversal of trends has also been corroborated by recent multicenter trials demonstrating no difference in clinical outcomes between operative and nonoperative treatment of high grade AC joint dislocations, albeit these patients were not all high level overhead throwing athletes.17,18
Continue to: The trends in surgical interventions are notable...
The trends in surgical interventions are notable within the smaller subset of patients who are indicated for operative repair. Use of hardware and primary ligament repair, while popular in the surveys conducted in the 1970s10 and 1990s13 and even present in Nissen and Chatterjee’s20 2007 survey, were noticeably absent from our survey results, with the majority of respondents preferring open coracoclavicular ligament reconstruction. The role of distal clavicle excision has also expanded, from 23% of team physicians recommending it in 199713 to 57% to 59% in Nissen and Chatterjee’s20 2007 survey, to 66.7% in our series. This trend is not surprising as several recent cadaveric biomechanical studies have demonstrated that not only do peak graft forces not increase significantly,21 the anterior-posterior and superior-inferior motion at the AC joint following ligament reconstruction is maintained despite resection of the lateral clavicle.22 Additionally, primary distal clavicle excision may prevent the development of post-traumatic arthritis at the AC joint and osteolysis of the distal clavicle as a possible pain generator in the future.23 However, some respondents cautioned against performing a concomitant distal clavicle excision, as some biomechanical data demonstrate that resecting the distal clavicle may lead to increased horizontal translation at the AC joint despite intact superior and posterior AC capsules.24 Professional baseball pitchers may also be more lax and thus prone to more instability. Primary repair or reconstruction may not always lead to complete pre-injury stability in these individuals. This subtle unrecognized instability is hard to diagnosis and may be a persistent source of pain; thus, adding a distal clavicle excision may actually exacerbate the instability.
The nuanced indications for operative intervention, such as the presence of associated lesions were not captured by our survey.25 While most team physicians cited functional limitations as their most common reason for offering surgery, several MLB orthopedic surgeons also commented on evaluating the stability of the AC joint after a grade III injury, akin to the consensus statement from the International Society of Arthroscopy, Knee Surgery and Orthopaedic Sports Medicine (ISAKOS) Upper Extremity Committee26 in 2014 that diversified the Rockwood Grade III AC joint separation into its IIIA and IIIB classifications. The ISAKOS recommendations include initial conservative management and a second evaluation (both clinical and radiographic) for grade III lesions 3 to 6 weeks after the injury. However, as professional baseball is an incredibly profitable sport with an annual revenue approaching $9.5 billion27 and pitching salaries up to $32.5 million in 2015, serious financial considerations must be given to players who wish to avoid undergoing delayed surgery.
This study has shortcomings typical of expert opinion papers. The retrospective nature of this study places the data at risk of recall bias. Objective data (eg, terminal ROM, pain relief, and return to play) were obtained from a retrospective chart review; however, no standard documentation or collection method was used given the number of surgeons involved and, thus, conclusions based on treatment outcomes are imperfect. Another major weakness of this survey is the relatively small number of patients and respondents. An a priori power analysis was not available, as this was a retrospective review. A comparative trial will be necessary to definitively support one treatment over another. Assuming a 95% return to play in the nonoperatively treated group, approximately 300 patients would be needed in a prospective 2-armed study with 80% power to detect a 10% reduction in the incidence of return to play using an alpha level of 0.05 and assuming no loss to follow-up. This sample size would be difficult to achieve in this patient population.
However, compared to past series,13 the number of professional baseball players treated by the collective experience of these MLB team physicians is the largest reported to date. As suggested above, the rarity of this condition in elite athletes precludes the ability to have matched controls to definitively determine the optimal treatment, which may explain the lack of difference in the return to play, ROM, and pain relief outcomes. Instead, we can only extrapolate based on the collective anecdotal experience of the MLB team physicians.
CONCLUSION
Despite advances in surgical technique and understanding of throwing mechanics, the majority of MLB team physicians preferred nonoperative management for an acute grade III AC joint separation in a professional baseball pitcher. Open coracoclavicular ligament reconstruction was preferred for those who preferred operative intervention. An increasing number of orthopedic surgeons now consider a distal clavicle excision as an adjunct procedure.
This paper will be judged for the Resident Writer’s Award.
- Spencer EE Jr. Treatment of grade III acromioclavicular joint injuries: a systematic review. Clin Orthop Relat Res. 2007;455:38-44. doi:10.1097/BLO.0b013e318030df83.
- Ceccarelli E, Bondì R, Alviti F, Garofalo R, Miulli F, Padua R. Treatment of acute grade III acromioclavicular dislocation: A lack of evidence. J Orthop Traumatol. 2008;9(2):105-108. doi:10.1007/s10195-008-0013-7.
- Smith TO, Chester R, Pearse EO, Hing CB. Operative versus non-operative management following rockwood grade III acromioclavicular separation: a meta-analysis of the current evidence base. J Orthop Traumatol. 2011;12(1):19-27. doi:10.1007/s10195-011-0127-1.
- Beitzel K, Cote MP, Apostolakos J, et al. Current concepts in the treatment of acromioclavicular joint dislocations. Arthroscopy. 2013;29(2):387-397. doi:10.1016/j.arthro.2012.11.023.
- Korsten K, Gunning AC, Leenen LP. Operative or conservative treatment in patients with rockwood type III acromioclavicular dislocation: a systematic review and update of current literature. Int Orthop. 2014;38(4):831-838. doi:10.1007/s00264-013-2143-7.
- Modi CS, Beazley J, Zywiel MG, Lawrence TM, Veillette CJ. Controversies relating to the management of acromioclavicular joint dislocations. Bone Joint J. 2013;95-B(12):1595-1602. doi:10.1302/0301-620X.95B12.31802.
- Reid D, Polson K, Johnson L. Acromioclavicular joint separations grades I-III: a review of the literature and development of best practice guidelines. Sports Med. 2012;42(8):681-696. doi:10.2165/11633460-000000000-00000.
- Farber AJ, Cascio BM, Wilckens JH. Type III acromioclavicular separation: rationale for anatomical reconstruction. Am J Orthop. 2008;37(7):349-355.
- Li X, Ma R, Bedi A, Dines DM, Altchek DW, Dines JS. Management of acromioclavicular joint injuries. J Bone Joint Surg Am. 2014;96(1):73-84. doi:10.2106/JBJS.L.00734.
- Powers JA, Bach PJ. Acromioclavicular separations. Closed or open treatment? Clin Orthop Relat Res. 1974;104(104):213-223. doi:10.1097/00003086-197410000-00024.
- Glick JM, Milburn LJ, Haggerty JF, Nishimoto D. Dislocated acromioclavicular joint: follow-up study of 35 unreduced acromioclavicular dislocations. Am J Sports Med. 1977;5(6):264-270. doi:10.1177/036354657700500614.
- Watson ST, Wyland DJ. Return to play after nonoperative management for a severe type III acromioclavicular separation in the throwing shoulder of a collegiate pitcher. Phys Sportsmed. 2015;43(1):99-103. doi:10.1080/00913847.2015.1001937.
- McFarland EG, Blivin SJ, Doehring CB, Curl LA, Silberstein C. Treatment of grade III acromioclavicular separations in professional throwing athletes: results of a survey. Am J Orthop. 1997;26(11):771-774.
- Wojtys EM, Nelson G. Conservative treatment of grade III acromioclavicular dislocations. Clin Orthop Relat Res. 1991;268(268):112-119.
- Galpin RD, Hawkins RJ, Grainger RW. A comparative analysis of operative versus nonoperative treatment of grade III acromioclavicular separations. Clin Orthop Relat Res. 1985;193(193):150-155. doi:10.1097/00003086-198503000-00020.
- Pallis M, Cameron KL, Svoboda SJ, Owens BD. Epidemiology of acromioclavicular joint injury in young athletes. Am J Sports Med. 2012;40(9):2072-2077. doi:10.1177/0363546512450162.
- Canadian Orthopaedic Trauma Society. Multicenter randomized clinical trial of nonoperative versus operative treatment of acute acromio-clavicular joint dislocation. J Orthop Trauma. 2015;29(11):479-487. doi:10.1097/BOT.0000000000000437.
- Joukainen A, Kröger H, Niemitukia L, Mäkelä EA, Väätäinen U. Results of operative and nonoperative treatment of rockwood types III and V acromioclavicular joint dislocation: a prospective, randomized trial with an 18- to 20-year follow-up. Orthop J Sports Med. 2014;2(12):2325967114560130. doi:10.1177/2325967114560130.
- Cox JS. Current method of treatment of acromioclavicular joint dislocations. Orthopedics. 1992;15(9):1041-1044.
- Nissen CW, Chatterjee A. Type III acromioclavicular separation: results of a recent survey on its management. Am J Orthop. 2007;36(2):89-93.
- Kowalsky MS, Kremenic IJ, Orishimo KF, McHugh MP, Nicholas SJ, Lee SJ. The effect of distal clavicle excision on in situ graft forces in coracoclavicular ligament reconstruction. Am J Sports Med. 2010;38(11):2313-2319. doi:10.1177/0363546510374447.
- Beaver AB, Parks BG, Hinton RY. Biomechanical analysis of distal clavicle excision with acromioclavicular joint reconstruction. Am J Sports Med. 2013;41(7):1684-1688. doi:10.1177/0363546513488750.
- Mumford EB. Acromioclavicular dislocation. J Bone Joint Surg Am. 1941;23:799-802.
- Beitzel K, Sablan N, Chowaniec DM, et al. Sequential resection of the distal clavicle and its effects on horizontal acromioclavicular joint translation. Am J Sports Med. 2012;40(3):681-685. doi:10.1177/0363546511428880.
- Arrigoni P, Brady PC, Zottarelli L, et al. Associated lesions requiring additional surgical treatment in grade 3 acromioclavicular joint dislocations. Arthroscopy. 2014;30(1):6-10. doi:10.1016/j.arthro.2013.10.006.
- Beitzel K, Mazzocca AD, Bak K, et al. ISAKOS upper extremity committee consensus statement on the need for diversification of the rockwood classification for acromioclavicular joint injuries. Arthroscopy. 2014;30(2):271-278. doi:10.1016/j.arthro.2013.11.005.
- Brown M. MLB sees record revenues for 2015, up $500 million and approaching $9.5 billion. Forbes Web site. http://www.forbes.com/sites/maurybrown/2015/12/04/mlb-sees-record-revenu.... Published December 4, 2015. Accessed February 4, 2016.
- Spencer EE Jr. Treatment of grade III acromioclavicular joint injuries: a systematic review. Clin Orthop Relat Res. 2007;455:38-44. doi:10.1097/BLO.0b013e318030df83.
- Ceccarelli E, Bondì R, Alviti F, Garofalo R, Miulli F, Padua R. Treatment of acute grade III acromioclavicular dislocation: A lack of evidence. J Orthop Traumatol. 2008;9(2):105-108. doi:10.1007/s10195-008-0013-7.
- Smith TO, Chester R, Pearse EO, Hing CB. Operative versus non-operative management following rockwood grade III acromioclavicular separation: a meta-analysis of the current evidence base. J Orthop Traumatol. 2011;12(1):19-27. doi:10.1007/s10195-011-0127-1.
- Beitzel K, Cote MP, Apostolakos J, et al. Current concepts in the treatment of acromioclavicular joint dislocations. Arthroscopy. 2013;29(2):387-397. doi:10.1016/j.arthro.2012.11.023.
- Korsten K, Gunning AC, Leenen LP. Operative or conservative treatment in patients with rockwood type III acromioclavicular dislocation: a systematic review and update of current literature. Int Orthop. 2014;38(4):831-838. doi:10.1007/s00264-013-2143-7.
- Modi CS, Beazley J, Zywiel MG, Lawrence TM, Veillette CJ. Controversies relating to the management of acromioclavicular joint dislocations. Bone Joint J. 2013;95-B(12):1595-1602. doi:10.1302/0301-620X.95B12.31802.
- Reid D, Polson K, Johnson L. Acromioclavicular joint separations grades I-III: a review of the literature and development of best practice guidelines. Sports Med. 2012;42(8):681-696. doi:10.2165/11633460-000000000-00000.
- Farber AJ, Cascio BM, Wilckens JH. Type III acromioclavicular separation: rationale for anatomical reconstruction. Am J Orthop. 2008;37(7):349-355.
- Li X, Ma R, Bedi A, Dines DM, Altchek DW, Dines JS. Management of acromioclavicular joint injuries. J Bone Joint Surg Am. 2014;96(1):73-84. doi:10.2106/JBJS.L.00734.
- Powers JA, Bach PJ. Acromioclavicular separations. Closed or open treatment? Clin Orthop Relat Res. 1974;104(104):213-223. doi:10.1097/00003086-197410000-00024.
- Glick JM, Milburn LJ, Haggerty JF, Nishimoto D. Dislocated acromioclavicular joint: follow-up study of 35 unreduced acromioclavicular dislocations. Am J Sports Med. 1977;5(6):264-270. doi:10.1177/036354657700500614.
- Watson ST, Wyland DJ. Return to play after nonoperative management for a severe type III acromioclavicular separation in the throwing shoulder of a collegiate pitcher. Phys Sportsmed. 2015;43(1):99-103. doi:10.1080/00913847.2015.1001937.
- McFarland EG, Blivin SJ, Doehring CB, Curl LA, Silberstein C. Treatment of grade III acromioclavicular separations in professional throwing athletes: results of a survey. Am J Orthop. 1997;26(11):771-774.
- Wojtys EM, Nelson G. Conservative treatment of grade III acromioclavicular dislocations. Clin Orthop Relat Res. 1991;268(268):112-119.
- Galpin RD, Hawkins RJ, Grainger RW. A comparative analysis of operative versus nonoperative treatment of grade III acromioclavicular separations. Clin Orthop Relat Res. 1985;193(193):150-155. doi:10.1097/00003086-198503000-00020.
- Pallis M, Cameron KL, Svoboda SJ, Owens BD. Epidemiology of acromioclavicular joint injury in young athletes. Am J Sports Med. 2012;40(9):2072-2077. doi:10.1177/0363546512450162.
- Canadian Orthopaedic Trauma Society. Multicenter randomized clinical trial of nonoperative versus operative treatment of acute acromio-clavicular joint dislocation. J Orthop Trauma. 2015;29(11):479-487. doi:10.1097/BOT.0000000000000437.
- Joukainen A, Kröger H, Niemitukia L, Mäkelä EA, Väätäinen U. Results of operative and nonoperative treatment of rockwood types III and V acromioclavicular joint dislocation: a prospective, randomized trial with an 18- to 20-year follow-up. Orthop J Sports Med. 2014;2(12):2325967114560130. doi:10.1177/2325967114560130.
- Cox JS. Current method of treatment of acromioclavicular joint dislocations. Orthopedics. 1992;15(9):1041-1044.
- Nissen CW, Chatterjee A. Type III acromioclavicular separation: results of a recent survey on its management. Am J Orthop. 2007;36(2):89-93.
- Kowalsky MS, Kremenic IJ, Orishimo KF, McHugh MP, Nicholas SJ, Lee SJ. The effect of distal clavicle excision on in situ graft forces in coracoclavicular ligament reconstruction. Am J Sports Med. 2010;38(11):2313-2319. doi:10.1177/0363546510374447.
- Beaver AB, Parks BG, Hinton RY. Biomechanical analysis of distal clavicle excision with acromioclavicular joint reconstruction. Am J Sports Med. 2013;41(7):1684-1688. doi:10.1177/0363546513488750.
- Mumford EB. Acromioclavicular dislocation. J Bone Joint Surg Am. 1941;23:799-802.
- Beitzel K, Sablan N, Chowaniec DM, et al. Sequential resection of the distal clavicle and its effects on horizontal acromioclavicular joint translation. Am J Sports Med. 2012;40(3):681-685. doi:10.1177/0363546511428880.
- Arrigoni P, Brady PC, Zottarelli L, et al. Associated lesions requiring additional surgical treatment in grade 3 acromioclavicular joint dislocations. Arthroscopy. 2014;30(1):6-10. doi:10.1016/j.arthro.2013.10.006.
- Beitzel K, Mazzocca AD, Bak K, et al. ISAKOS upper extremity committee consensus statement on the need for diversification of the rockwood classification for acromioclavicular joint injuries. Arthroscopy. 2014;30(2):271-278. doi:10.1016/j.arthro.2013.11.005.
- Brown M. MLB sees record revenues for 2015, up $500 million and approaching $9.5 billion. Forbes Web site. http://www.forbes.com/sites/maurybrown/2015/12/04/mlb-sees-record-revenu.... Published December 4, 2015. Accessed February 4, 2016.
TAKE-HOME POINTS
- There was no difference in return to previous level of play between professional pitchers treated nonoperatively and operatively for grade III AC separation.
- MLB team physicians prefer nonoperative management for acute grade III AC joint separation in professional pitchers.
- The majority of MLB physicians do not use injections for nonoperative treatment of grade III AC separations; however, use of orthobiologics (eg, PRP) is becoming more commonplace.
- Persistent functional limitations and pain are the most common surgical indications for treatment of grade III AC separation in high level throwing athletes.
- If operative intervention is indicated for grade III AC separation, open coracoclavicular reconstruction and adjunct distal clavicle excision are preferred by most MLB team physicians.
Short-Term Storage of Platelet-Rich Plasma at Room Temperature Does Not Affect Growth Factor or Catabolic Cytokine Concentration
ABSTRACT
The aim of this study was to provide clinical recommendations about the use of platelet-rich plasma (PRP) that was subjected to short-term storage at room temperature. We determined bioactive growth factor and cytokine concentrations as indicators of platelet and white blood cell degranulation in blood and PRP. Additionally, this study sought to validate the use of manual, direct smear analysis as an alternative to automated methods for platelet quantification in PRP.
Blood was used to generate low-leukocyte PRP (Llo PRP) or high-leukocyte PRP (Lhi PRP). Blood was either processed immediately or kept at room temperature for 2 or 4 hours prior to generation of PRP, which was then held at room temperature for 0, 1, 2, or 4 hours. Subsequently, bioactive transforming growth factor beta-1 and matrix metalloproteinase-9 were measured by ELISA (enzyme-linked immunosorbent assay). Manual and automated platelet counts were performed on all blood and PRP samples.
There were no differences in growth factor or cytokine concentration when blood or Llo PRP or Lhi PRP was retained at room temperature for up to 4 hours. Manual, direct smear analysis for platelet quantification was not different from the use of automated machine counting for PRP samples, but in the starting blood samples, manual platelet counts were significantly higher than those generated using automated technology.
When there is a delay of up to 4 hours in the generation of PRP from blood or in the application of PRP to the patient, bioactive growth factor and cytokine concentrations remain stable in both blood and PRP. A manual direct counting method is a simple, cost-effective, and valid method to measure the contents of the PRP product being delivered to the patient.
Platelet-rich plasma (PRP) is used to promote healing in many areas of medicine, such as dental surgery,1,2 soft-tissue injury,3,4 orthopedic surgery,5,6 wound healing,7 and veterinary medicine.8,9 Despite its extensive use, there are still questions about the clinical efficacy of PRP.10-12 Due to biological heterogeneity between patients13,14 and differences between available manufacturing kits,13,15 PRP can be highly variable between patients. There are classification schemes to categorize the various types of PRP,16-18 which can be divided broadly into low-leukocyte PRP (Llo PRP) and high-leukocyte PRP (Lhi PRP). PRP can be used as a point of care therapy, prepared and used immediately, or it can be used during a surgical procedure. In some institutions, blood is drawn by a phlebotomist, processed in the hospital laboratory, and then delivered to the operating room. In other instances, PRP is generated patient-side by the primary attending physician’s team, who draws the blood and processes it for immediate use.5,19 Delays at any step in these various scenarios could lead to the blood or the resultant PRP remaining at room temperature from minutes to several hours prior to administration to the patient. This variability in PRP protocols between clinical and surgical settings adds to concerns regarding the stability and efficacy of the biologic.
Continue to: When performing clinical or research...
When performing clinical or research studies using PRP, it is important to report the contents of the PRP delivered to the patient. By documenting the cellular content of the PRP delivered to the patient, the common questions of optimal platelet dose and the importance of leukocytes in PRP can begin to be answered. There are some known factors that contribute to PRP variability, such as patient biology and operator technique, but there are many other unknown factors. In some instances, there is a failure to generate PRP, defined as a lower platelet count in the PRP preparation than in the starting blood sample.13,14 To measure the platelet and cellular contents of the starting blood and PRP, samples can be submitted to a clinical pathology laboratory for a complete blood count, which adds cost to the patient above the typically unreimbursed cost of the PRP injection itself. An alternative method for measuring platelet concentrations is the use of direct smear analysis on glass slides. The use of direct smears to measure platelet concentration is well validated for blood,20,21 but the use of direct smears of PRP for determining platelet concentrations has not been previously validated. The use of manual platelet counts would provide an alternative to automated platelet counting for clinical and preclinical research studies to characterize the type of PRP administered to the patient.
The primary aim of this study was to determine if retention of blood or PRP at room temperature for various time intervals had an effect on final growth factor or catabolic cytokine concentration. Bioactive transforming growth factor-β1 (TGF-β1) and matrix metalloproteinase-9 (MMP-9) were measured as representatives of growth factors and catabolic cytokines, respectively. The secondary aim was to identify if manual platelet counts were an accurate reflection of automated counts. The outcomes of these experiments should provide immediately relevant information for the clinical application of PRP.
MATERIALS AND METHODS
Blood Collection and Generation of PRP
Under Institutional Review Board approval, blood (105 mL) was collected from healthy human volunteers (N = 5) into a syringe containing acid citrate dextrose anticoagulant to a final concentration of 10% acid citrate dextrose. Three 15-mL aliquots of blood were used to generate Llo PRP (Autologous Conditioned Plasma Double Syringe, Arthrex) and three 20-mL aliquots were used to generate Lhi PRP (SmartPReP 2, Harvest Technologies) (Figure 1).
Automated and Manual Counts
Automated complete blood counts were performed by a board certified clinical pathologist in the clinical pathology department of Cornell University on all blood, Llo PRP, and Lhi PRP samples. A manual platelet count, using a modified Giemsa stain,22 was performed on smears of all blood and PRP samples (Video). Slides were scanned at 10x magnification to identify an area where many red blood cells were present while maintaining a clear field of view (Figure 2A). The magnification was then increased to 100x using oil immersion, and the total number of platelets was counted in 10 fields of view (Figure 2B).
Growth Factor and Catabolic Cytokine Measurements
Blood and PRP samples were thawed for ELISA (enzyme-linked immunosorbent assay) analysis. TGF-β1 concentration was determined using the TGF-β1 Emax ImmunoAssay System (Promega Corporation), which measures biologically active TGF-β1. We chose TGF-β1 because it is commonly measured in PRP studies as an anabolic cytokine with multiple effects on tissue healing. The functions of TGF-β1 include stimulation of undifferentiated mesenchymal cell proliferation; regulation of endothelial, fibroblast, and osteoblast mitogenesis; coordination of collagen synthesis; promotion of endothelial chemotaxis and angiogenesis; activation of extracellular matrix synthesis in cartilage; and reduction of the catabolic activity of interluekin-1 and MMPs.23-25 In addition, TGF-β1 concentration strongly correlates with platelet concentration.26 MMP-9 concentration was determined using the MMP-9 Biotrak Activity Assay (GE Healthcare Biosciences) which measures both active and pro- forms of MMP-9. In PRP, MMP-9 was measured as an indicator of white blood cell (WBC) concentration.26 A catabolic cytokine capable of degrading collagen,13,27 MMP-9 has been linked to poor healing.28 For both assays, samples were measured in duplicate using a multiple detection plate reader (Tecan Safire).
Continue to: Statistical Analysis...
Statistical Analysis
Data were tested for the normal distribution to determine the appropriate statistical test. Manual and automated platelet counts were compared to each other in whole blood, Llo PRP, and Lhi PRP samples using a paired t test. Bioactive TGF-β1 concentrations in blood, Llo PRP, and Lhi PRP, were compared using a Kruskal-Wallis one-way analysis of variance (ANOVA) with Dunn’s all-pairwise comparison. Bioactive and pro-MMP-9 concentrations measured in the retained blood or PRP samples were compared using a one-way ANOVA with Tukey’s all-pairwise comparison. Statistical analyses were performed using Statistix 9 software (Analytical Software). A P value of <0.05 was considered significant.
RESULTS
Validation of PRP
PRP, as defined by an increase in platelet concentration in PRP compared with blood, was successfully generated in all samples by both systems. There was an average 1.98 ± 0.14-fold increase in platelet concentration in Llo PRP and an average 3.06 ± 0.24-fold increase in Lhi PRP. Platelet concentration was significantly higher in Lhi PRP than in Llo PRP (P = 0.001). Compared to whole blood, WBC concentration was 0.47 ± 0.07-fold lower in Llo PRP and 1.98 ± 0.14-fold greater in Lhi PRP. Similar to platelets, WBCs were significantly greater in Lhi PRP than in Llo PRP (P = 0.02).
Bioactive TGF-β1 and MMP-9 Concentration in Blood Retained at Room Temperature
To reflect the clinical situation where blood would be drawn from a patient, but there would be a delay in processing the blood to generate PRP, blood samples were retained at room temperature for up to 4 hours prior to analysis. Neither bioactive TGF-β1 (Figure 3) nor bioactive/pro-MMP-9 concentrations (Figure 4) changed significantly over time when blood was retained at room temperature prior to centrifugation to generate PRP.
Bioactive TGF-β1 and MMP-9 Concentration in PRP Retained at Room Temperature
In order to mimic the clinical situation where PRP would be generated but might sit out prior to being administered to the patient, PRP samples were retained at room temperature for up to 4 hours prior to analysis. In these samples, bioactive TGF-β1 concentrations were not significantly different between PRP products analyzed immediately and those samples retained at room temperature for up to 4 hours (Figure 5).
Automatic vs Manual Platelet Count
Manual platelet counts were compared to automated platelet counts to determine if a manual platelet smear analysis could be a reliable method for analyzing PRP in clinical and pre-clinical studies. There was a significant difference between the automated and manual platelet counts in blood samples (Table) (P = 0.05, N = 5) with the manual platelet count having a higher average (99.1 thou/uL) platelet concentration than automated counts. Platelet clumping was identified in 2 automated counts, which falsely decreased platelet concentration by an unknown quantity. Manual platelet counts for both Llo PRP (n = 30) and Lhi PRP (n = 30) were not different from automated platelet counts. Platelet clumping was not reported on any manual platelet counts performed on PRP samples.
Table. Platelet Concentrations of Whole Blood, Llo PRP, and Lhi PRP (N = 5)
| |||||
| Automated Count | Manual Count | P Value | ||
| Mean ± SD | Range | Mean ± SD | Range |
|
Blood | 111.8 ± 59.5 | 54-202 | 210.9 ± 59.4 | 144-297 | 0.05 |
Llo PRP | 421.4 ± 132.8 | 319-620 | 410.1 ± 94.2 | 318-543 | 0.61 |
Lhi PRP | 634.4 ± 88.8 | 517-766 | 635.4 ± 176.6 | 491-933 | 0.99 |
A paired t test was performed to compare results obtained from an automated platelet count and those obtained from a manual count.
Abbreviations: Lhi PRP, high-leukocyte platelet-rich plasma; Llo PRP, low-leukocyte platelet-rich plasma; SD, standard deviation.
Continue to:The primary aim of this study...
DISCUSSION
The primary aim of this study was to improve the clinical use of PRP by characterizing changes that might occur due to extended preparation times. Physicians commonly question the stability of blood or PRP if it is retained at room temperature prior to being administered to the patient. Clinical recommendations to optimize PRP preparation can be derived from a better understanding of the stability of platelets and WBCs, which contribute to the anabolic and catabolic cytokines in PRP.
The results of this study suggest that platelets and WBCs remain stable in blood and both Llo PRP and Lhi PRP for up to 4 hours. The use of bioactive ELISAs to measure TGF-β1 and MMP-9 allows for determination of stability of the PRP product retained at room temperature for up to 4 hours. This provides a time buffer to allow for delays from either institutional logistics or unanticipated clinical delays, without adverse effects on the generation of the final PRP product. As with all biologics, there are many factors that contribute to variability, but a relatively short delay of up to 4 hours in either generation of PRP from blood or in administration of PRP to the patient does not appear to contribute to that variability. Similar studies have been performed on equine PRP and suggest that growth factor concentrations remain stable for up to 6 hours after preparation of PRP29 and in human PRP, which implies that although samples degrade over time, platelet integrity might be acceptable for clinical use for up to 5 days after preparation, particularly if stored in oxygen.30 In contrast to this study, neither of the previously published reports used assays to measure biological activity in the stored PRP. Regardless of the variability between the studies with respect to the type of PRP evaluated and the outcome measures used, all of the studies support the concept that PRP can be stored at room temperature for at least a few hours before clinical use.
Centrifugation of blood does not guarantee the generation of PRP.13,14 In some cases, platelet counts in PRP are similar to or even less than that in the starting whole blood sample. To determine whether a clinical outcome is attributed to PRP, it is vital to know the platelet concentration and, arguably, the WBC concentration in the blood used to generate PRP and in the PRP sample administered to the patient. The platelet concentration in blood and PRP samples can be quantified using automated or manual methods. The use of automated methods can add significant cost to a study or procedure. Manually evaluating a blood smear is an accepted, though more time consuming, method of analyzing cellular components of a blood sample. Depending on the standard operating procedure of the laboratory, manual smears are often done in conjunction with an automated count. This identifies abnormalities in cellular shape or size, or platelet clumping, which are not consistently recognized by automated methods. Manually evaluating a blood smear does take some training, but the material cost is very low, which has added value for clinical or preclinical research studies. Interestingly, the results of this study indicate that manual platelet counts in blood may be more accurate than the count generated from an automated counter because the automated platelet counts were falsely low due to platelet clumping. Platelet clumping can occur as early as 1 hour after blood collection, regardless of the type of anticoagulant used.31
LIMITATIONS
The sample size of this study was small. However, variability in PRP has been well documented in multiple other studies using slightly larger sample sizes.13,14,16 Another potential limitation of this study could be that only one growth factor, TGF-β1, and one catabolic cytokine, MMP-9, were used as surrogate measures to represent platelet and WBC stability, respectively. We chose TGF-β1 because it is correlated with platelet concentrations14,15,26 and MMP-9 because it is an indicator of catabolic factors in PRP that have been correlated with WBC concentrations.26
CONCLUSION
This study illustrated that growth factor and cytokine concentrations in both Llo PRP and Lhi PRP are stable for up to 4 hours. The clinical implications of these results suggest that if the generation or administration of PRP is delayed by up to 4 hours, the resultant PRP retains its bioactivity and is acceptable for clinical application. However, given the known variability of PRP generated due to patient and manufacturer variability,13,14 it is still important to ensure that the product is indeed PRP, with an increase in platelet number over the starting sample of blood. This validation can be performed with a simple and cost-effective manual smear analysis of blood and PRP. The results of this study provide information that can be immediately translated into clinical, surgical, and research practices.
1. Nikolidakis D, Jansen JA. The biology of platelet-rich plasma and its application in oral surgery: Literature review. Tissue Eng Part B Rev. 2008;14(3):249-258. doi:10.1089/ten.teb.2008.0062.
2. Sánchez AR, Sheridan PJ, Kupp LI. Is platelet-rich plasma the perfect enhancement factor? A current review. Int J Oral Maxillofac Implants. 2003;18(1):93-103.
3. Monto RR. Platelet rich plasma treatment for chronic achilles tendinosis. Foot Ankle Int. 2012;33(5):379-385. doi:10.3113/FAI.2012.0379.
4. Owens RF, Ginnetti J, Conti SF, Latona C. Clinical and magnetic resonance imaging outcomes following platelet rich plasma injection for chronic midsubstance Achilles tendinopathy. Foot ankle Int. 2011;32(11):1032-1039. doi:10.3113/FAI.2011.1032.
5. Sánchez M, Anitua E, Azofra J, Andía I, Padilla S, Mujika I. Comparison of surgically repaired achilles tendon tears using platelet-rich fibrin matrices. Am J Sports Med. 2007;35(2):245-251. doi:10.1177/0363546506294078.
6. Silva A, Sampaio R. Anatomic ACL reconstruction: does the platelet-rich plasma accelerate tendon healing? Knee Surg Sports Traumatol Arthrosc. 2009;17(6):676-682. doi:10.1007/s00167-009-0762-8.
7. Fréchette JP, Martineau I, Gagnon G. Platelet-rich plasmas: growth factor content and roles in wound healing. J Dent Res. 2005;84(5):434-439. doi:10.1177/154405910508400507.
8. Bosch G, René van Weeren P, Barneveld A, van Schie HTM. Computerised analysis of standardised ultrasonographic images to monitor the repair of surgically created core lesions in equine superficial digital flexor tendons following treatment with intratendinous platelet rich plasma or placebo. Vet J. 2011;187(1):92-98. doi:10.1016/j.tvjl.2009.10.014.
9. Torricelli P, Fini M, Filardo G, et al. Regenerative medicine for the treatment of musculoskeletal overuse injuries in competition horses. Int Orthop. 2011;35(10):1569-1576. doi:10.1007/s00264-011-1237-3.
10. Sampson S, Gerhardt M, Mandelbaum B. Platelet rich plasma injection grafts for musculoskeletal injuries: a review. Curr Rev Musculoskelet Med. 2008;1(3-4):165-174. doi:10.1007/s12178-008-9032-5.
11. Sheth U, Simunovic N, Klein G, et al. Efficacy of autologous platelet-rich plasma use for orthopaedic indications: a meta-analysis. J Bone Joint Surg Am. 2012;94(4):298-307. doi:10.2106/JBJS.K.00154.
12. Vannini F, Di Matteo B, Filardo G, Kon E, Marcacci M, Giannini S. Platelet-rich plasma for foot and ankle pathologies: a systematic review. Foot Ankle Surg. 2014;20(1):2-9. doi:10.1016/j.fas.2013.08.001.
13. Boswell SG, Cole BJ, Sundman EA, Karas V, Fortier LA. Platelet-rich plasma: a milieu of bioactive factors. Arthroscopy. 2012;28(3):429-439. doi:10.1016/j.arthro.2011.10.018.
14. Mazzocca AD, McCarthy MBR, Chowaniec DM, et al. Platelet-rich plasma differs according to preparation method and human variability. J Bone Joint Surg Am. 2012;94(4):308-316. doi:10.2106/JBJS.K.00430.
15. Castillo TN, Pouliot MA, Kim HJ, Dragoo JL. Comparison of growth factor and platelet concentration from commercial platelet-rich plasma separation systems. Am J Sports Med. 2011;39(2):266-271. doi:10.1177/0363546510387517.
16. Arnoczky SP, Sheibani-Rad S, Shebani-Rad S. The basic science of platelet-rich plasma (PRP): what clinicians need to know. Sports Med Arthrosc. 2013;21(4):180-185. doi:10.1097/JSA.0b013e3182999712.
17. Dohan Ehrenfest DM, Bielecki T, Corso M Del, Inchingolo F, Sammartino G. Shedding light in the controversial terminology for platelet-rich products: Platelet-rich plasma (PRP), platelet-rich fibrin (PRF), platelet-leukocyte gel (PLG), preparation rich in growth factors (PRGF), classification and commercialism. J Biomed Mater Res Part A. 2010;95A(4):1280-1282. doi:10.1002/jbm.a.32894.
18. Dohan Ehrenfest DM, Rasmusson L, Albrektsson T. Classification of platelet concentrates: from pure platelet-rich plasma (P-PRP) to leucocyte- and platelet-rich fibrin (L-PRF). Trends Biotechnol. 2009;27(3):158-167. doi:10.1016/j.tibtech.2008.11.009.
19. Everts PA, Knape JT, Weibrich G, et al. Platelet-rich plasma and platelet gel: a review. J Extra Corpor Technol. 2006;38(2):174-187.
20. Malok M, Titchener EH, Bridgers C, Lee BY, Bamberg R. Comparison of two platelet count estimation methodologies for peripheral blood smears. Clin Lab Sci. 2007;20(3):154-160.
21. Gulati G, Uppal G, Florea AD, Gong J. Detection of platelet clumps on peripheral blood smears by CellaVision DM96 System and Microscopic Review. Lab Med. 2014;45(4):368-371. doi:10.1309/LM604RQVKVLRFXOR.
22. Gulati G, Song J, Florea AD, Gong J. Purpose and criteria for blood smear scan, blood smear examination, and blood smear review. Ann Lab Med. 2013;33(1):1-7. doi:10.3343/alm.2013.33.1.1.
23. Barrientos S, Stojadinovic O, Golinko MS, Brem H, Tomic-Canic M. Perspective article: Growth factors and cytokines in wound healing. Wound Repair Regen. 2008;16(5):585-601. doi:10.1111/j.1524-475X.2008.00410.x.
24. Crane D, Everts P. Platelet rich plasma (PRP) matrix grafts. Pract Pain Manag. 2008;8(1):12-26.
25. Fortier LA, Barker JU, Strauss EJ, McCarrel TM, Cole BJ. The role of growth factors in cartilage repair. Clin Orthop Relat Res. 2011;469(10):2706-2715. doi:10.1007/s11999-011-1857-3.
26. Sundman EA, Cole BJ, Fortier LA. Growth factor and catabolic cytokine concentrations are influenced by the cellular composition of platelet-rich plasma. Am J Sports Med. 2011;39(10):2135-2140. doi:10.1177/0363546511417792.
27. Vu TH, Shipley JM, Bergers G, et al. MMP-9/gelatinase B is a key regulator of growth plate angiogenesis and apoptosis of hypertrophic chondrocytes. Cell. 1998;93(3):411-422.
28. Watelet JB, Demetter P, Claeys C, Van Cauwenberge P, Cuvelier C, Bachert C. Neutrophil-derived metalloproteinase-9 predicts healing quality after sinus surgery. Laryngoscope. 2005;115(1):56-61. doi:10.1097/01.mlg.0000150674.30237.3f.
29. Hauschild G, Geburek F, Gosheger G, et al. Short term storage stability at room temperature of two different platelet-rich plasma preparations from equine donors and potential impact on growth factor concentrations. BMC Vet Res. 2017;13(1):7. doi:10.1186/s12917-016-0920-4.
30. Moore GW, Maloney JC, Archer RA, et al. Platelet-rich plasma for tissue regeneration can be stored at room temperature for at least five days. Br J Biomed Sci. 2017;74(2):71-77. doi:10.1080/09674845.2016.1233792.
31. McShine RL, Sibinga S, Brozovic B. Differences between the effects of EDTA and citrate anticoagulants on platelet count and mean platelet volume. Clin Lab Haematol. 1990;12(3):277-285.
ABSTRACT
The aim of this study was to provide clinical recommendations about the use of platelet-rich plasma (PRP) that was subjected to short-term storage at room temperature. We determined bioactive growth factor and cytokine concentrations as indicators of platelet and white blood cell degranulation in blood and PRP. Additionally, this study sought to validate the use of manual, direct smear analysis as an alternative to automated methods for platelet quantification in PRP.
Blood was used to generate low-leukocyte PRP (Llo PRP) or high-leukocyte PRP (Lhi PRP). Blood was either processed immediately or kept at room temperature for 2 or 4 hours prior to generation of PRP, which was then held at room temperature for 0, 1, 2, or 4 hours. Subsequently, bioactive transforming growth factor beta-1 and matrix metalloproteinase-9 were measured by ELISA (enzyme-linked immunosorbent assay). Manual and automated platelet counts were performed on all blood and PRP samples.
There were no differences in growth factor or cytokine concentration when blood or Llo PRP or Lhi PRP was retained at room temperature for up to 4 hours. Manual, direct smear analysis for platelet quantification was not different from the use of automated machine counting for PRP samples, but in the starting blood samples, manual platelet counts were significantly higher than those generated using automated technology.
When there is a delay of up to 4 hours in the generation of PRP from blood or in the application of PRP to the patient, bioactive growth factor and cytokine concentrations remain stable in both blood and PRP. A manual direct counting method is a simple, cost-effective, and valid method to measure the contents of the PRP product being delivered to the patient.
Platelet-rich plasma (PRP) is used to promote healing in many areas of medicine, such as dental surgery,1,2 soft-tissue injury,3,4 orthopedic surgery,5,6 wound healing,7 and veterinary medicine.8,9 Despite its extensive use, there are still questions about the clinical efficacy of PRP.10-12 Due to biological heterogeneity between patients13,14 and differences between available manufacturing kits,13,15 PRP can be highly variable between patients. There are classification schemes to categorize the various types of PRP,16-18 which can be divided broadly into low-leukocyte PRP (Llo PRP) and high-leukocyte PRP (Lhi PRP). PRP can be used as a point of care therapy, prepared and used immediately, or it can be used during a surgical procedure. In some institutions, blood is drawn by a phlebotomist, processed in the hospital laboratory, and then delivered to the operating room. In other instances, PRP is generated patient-side by the primary attending physician’s team, who draws the blood and processes it for immediate use.5,19 Delays at any step in these various scenarios could lead to the blood or the resultant PRP remaining at room temperature from minutes to several hours prior to administration to the patient. This variability in PRP protocols between clinical and surgical settings adds to concerns regarding the stability and efficacy of the biologic.
Continue to: When performing clinical or research...
When performing clinical or research studies using PRP, it is important to report the contents of the PRP delivered to the patient. By documenting the cellular content of the PRP delivered to the patient, the common questions of optimal platelet dose and the importance of leukocytes in PRP can begin to be answered. There are some known factors that contribute to PRP variability, such as patient biology and operator technique, but there are many other unknown factors. In some instances, there is a failure to generate PRP, defined as a lower platelet count in the PRP preparation than in the starting blood sample.13,14 To measure the platelet and cellular contents of the starting blood and PRP, samples can be submitted to a clinical pathology laboratory for a complete blood count, which adds cost to the patient above the typically unreimbursed cost of the PRP injection itself. An alternative method for measuring platelet concentrations is the use of direct smear analysis on glass slides. The use of direct smears to measure platelet concentration is well validated for blood,20,21 but the use of direct smears of PRP for determining platelet concentrations has not been previously validated. The use of manual platelet counts would provide an alternative to automated platelet counting for clinical and preclinical research studies to characterize the type of PRP administered to the patient.
The primary aim of this study was to determine if retention of blood or PRP at room temperature for various time intervals had an effect on final growth factor or catabolic cytokine concentration. Bioactive transforming growth factor-β1 (TGF-β1) and matrix metalloproteinase-9 (MMP-9) were measured as representatives of growth factors and catabolic cytokines, respectively. The secondary aim was to identify if manual platelet counts were an accurate reflection of automated counts. The outcomes of these experiments should provide immediately relevant information for the clinical application of PRP.
MATERIALS AND METHODS
Blood Collection and Generation of PRP
Under Institutional Review Board approval, blood (105 mL) was collected from healthy human volunteers (N = 5) into a syringe containing acid citrate dextrose anticoagulant to a final concentration of 10% acid citrate dextrose. Three 15-mL aliquots of blood were used to generate Llo PRP (Autologous Conditioned Plasma Double Syringe, Arthrex) and three 20-mL aliquots were used to generate Lhi PRP (SmartPReP 2, Harvest Technologies) (Figure 1).
Automated and Manual Counts
Automated complete blood counts were performed by a board certified clinical pathologist in the clinical pathology department of Cornell University on all blood, Llo PRP, and Lhi PRP samples. A manual platelet count, using a modified Giemsa stain,22 was performed on smears of all blood and PRP samples (Video). Slides were scanned at 10x magnification to identify an area where many red blood cells were present while maintaining a clear field of view (Figure 2A). The magnification was then increased to 100x using oil immersion, and the total number of platelets was counted in 10 fields of view (Figure 2B).
Growth Factor and Catabolic Cytokine Measurements
Blood and PRP samples were thawed for ELISA (enzyme-linked immunosorbent assay) analysis. TGF-β1 concentration was determined using the TGF-β1 Emax ImmunoAssay System (Promega Corporation), which measures biologically active TGF-β1. We chose TGF-β1 because it is commonly measured in PRP studies as an anabolic cytokine with multiple effects on tissue healing. The functions of TGF-β1 include stimulation of undifferentiated mesenchymal cell proliferation; regulation of endothelial, fibroblast, and osteoblast mitogenesis; coordination of collagen synthesis; promotion of endothelial chemotaxis and angiogenesis; activation of extracellular matrix synthesis in cartilage; and reduction of the catabolic activity of interluekin-1 and MMPs.23-25 In addition, TGF-β1 concentration strongly correlates with platelet concentration.26 MMP-9 concentration was determined using the MMP-9 Biotrak Activity Assay (GE Healthcare Biosciences) which measures both active and pro- forms of MMP-9. In PRP, MMP-9 was measured as an indicator of white blood cell (WBC) concentration.26 A catabolic cytokine capable of degrading collagen,13,27 MMP-9 has been linked to poor healing.28 For both assays, samples were measured in duplicate using a multiple detection plate reader (Tecan Safire).
Continue to: Statistical Analysis...
Statistical Analysis
Data were tested for the normal distribution to determine the appropriate statistical test. Manual and automated platelet counts were compared to each other in whole blood, Llo PRP, and Lhi PRP samples using a paired t test. Bioactive TGF-β1 concentrations in blood, Llo PRP, and Lhi PRP, were compared using a Kruskal-Wallis one-way analysis of variance (ANOVA) with Dunn’s all-pairwise comparison. Bioactive and pro-MMP-9 concentrations measured in the retained blood or PRP samples were compared using a one-way ANOVA with Tukey’s all-pairwise comparison. Statistical analyses were performed using Statistix 9 software (Analytical Software). A P value of <0.05 was considered significant.
RESULTS
Validation of PRP
PRP, as defined by an increase in platelet concentration in PRP compared with blood, was successfully generated in all samples by both systems. There was an average 1.98 ± 0.14-fold increase in platelet concentration in Llo PRP and an average 3.06 ± 0.24-fold increase in Lhi PRP. Platelet concentration was significantly higher in Lhi PRP than in Llo PRP (P = 0.001). Compared to whole blood, WBC concentration was 0.47 ± 0.07-fold lower in Llo PRP and 1.98 ± 0.14-fold greater in Lhi PRP. Similar to platelets, WBCs were significantly greater in Lhi PRP than in Llo PRP (P = 0.02).
Bioactive TGF-β1 and MMP-9 Concentration in Blood Retained at Room Temperature
To reflect the clinical situation where blood would be drawn from a patient, but there would be a delay in processing the blood to generate PRP, blood samples were retained at room temperature for up to 4 hours prior to analysis. Neither bioactive TGF-β1 (Figure 3) nor bioactive/pro-MMP-9 concentrations (Figure 4) changed significantly over time when blood was retained at room temperature prior to centrifugation to generate PRP.
Bioactive TGF-β1 and MMP-9 Concentration in PRP Retained at Room Temperature
In order to mimic the clinical situation where PRP would be generated but might sit out prior to being administered to the patient, PRP samples were retained at room temperature for up to 4 hours prior to analysis. In these samples, bioactive TGF-β1 concentrations were not significantly different between PRP products analyzed immediately and those samples retained at room temperature for up to 4 hours (Figure 5).
Automatic vs Manual Platelet Count
Manual platelet counts were compared to automated platelet counts to determine if a manual platelet smear analysis could be a reliable method for analyzing PRP in clinical and pre-clinical studies. There was a significant difference between the automated and manual platelet counts in blood samples (Table) (P = 0.05, N = 5) with the manual platelet count having a higher average (99.1 thou/uL) platelet concentration than automated counts. Platelet clumping was identified in 2 automated counts, which falsely decreased platelet concentration by an unknown quantity. Manual platelet counts for both Llo PRP (n = 30) and Lhi PRP (n = 30) were not different from automated platelet counts. Platelet clumping was not reported on any manual platelet counts performed on PRP samples.
Table. Platelet Concentrations of Whole Blood, Llo PRP, and Lhi PRP (N = 5)
| |||||
| Automated Count | Manual Count | P Value | ||
| Mean ± SD | Range | Mean ± SD | Range |
|
Blood | 111.8 ± 59.5 | 54-202 | 210.9 ± 59.4 | 144-297 | 0.05 |
Llo PRP | 421.4 ± 132.8 | 319-620 | 410.1 ± 94.2 | 318-543 | 0.61 |
Lhi PRP | 634.4 ± 88.8 | 517-766 | 635.4 ± 176.6 | 491-933 | 0.99 |
A paired t test was performed to compare results obtained from an automated platelet count and those obtained from a manual count.
Abbreviations: Lhi PRP, high-leukocyte platelet-rich plasma; Llo PRP, low-leukocyte platelet-rich plasma; SD, standard deviation.
Continue to:The primary aim of this study...
DISCUSSION
The primary aim of this study was to improve the clinical use of PRP by characterizing changes that might occur due to extended preparation times. Physicians commonly question the stability of blood or PRP if it is retained at room temperature prior to being administered to the patient. Clinical recommendations to optimize PRP preparation can be derived from a better understanding of the stability of platelets and WBCs, which contribute to the anabolic and catabolic cytokines in PRP.
The results of this study suggest that platelets and WBCs remain stable in blood and both Llo PRP and Lhi PRP for up to 4 hours. The use of bioactive ELISAs to measure TGF-β1 and MMP-9 allows for determination of stability of the PRP product retained at room temperature for up to 4 hours. This provides a time buffer to allow for delays from either institutional logistics or unanticipated clinical delays, without adverse effects on the generation of the final PRP product. As with all biologics, there are many factors that contribute to variability, but a relatively short delay of up to 4 hours in either generation of PRP from blood or in administration of PRP to the patient does not appear to contribute to that variability. Similar studies have been performed on equine PRP and suggest that growth factor concentrations remain stable for up to 6 hours after preparation of PRP29 and in human PRP, which implies that although samples degrade over time, platelet integrity might be acceptable for clinical use for up to 5 days after preparation, particularly if stored in oxygen.30 In contrast to this study, neither of the previously published reports used assays to measure biological activity in the stored PRP. Regardless of the variability between the studies with respect to the type of PRP evaluated and the outcome measures used, all of the studies support the concept that PRP can be stored at room temperature for at least a few hours before clinical use.
Centrifugation of blood does not guarantee the generation of PRP.13,14 In some cases, platelet counts in PRP are similar to or even less than that in the starting whole blood sample. To determine whether a clinical outcome is attributed to PRP, it is vital to know the platelet concentration and, arguably, the WBC concentration in the blood used to generate PRP and in the PRP sample administered to the patient. The platelet concentration in blood and PRP samples can be quantified using automated or manual methods. The use of automated methods can add significant cost to a study or procedure. Manually evaluating a blood smear is an accepted, though more time consuming, method of analyzing cellular components of a blood sample. Depending on the standard operating procedure of the laboratory, manual smears are often done in conjunction with an automated count. This identifies abnormalities in cellular shape or size, or platelet clumping, which are not consistently recognized by automated methods. Manually evaluating a blood smear does take some training, but the material cost is very low, which has added value for clinical or preclinical research studies. Interestingly, the results of this study indicate that manual platelet counts in blood may be more accurate than the count generated from an automated counter because the automated platelet counts were falsely low due to platelet clumping. Platelet clumping can occur as early as 1 hour after blood collection, regardless of the type of anticoagulant used.31
LIMITATIONS
The sample size of this study was small. However, variability in PRP has been well documented in multiple other studies using slightly larger sample sizes.13,14,16 Another potential limitation of this study could be that only one growth factor, TGF-β1, and one catabolic cytokine, MMP-9, were used as surrogate measures to represent platelet and WBC stability, respectively. We chose TGF-β1 because it is correlated with platelet concentrations14,15,26 and MMP-9 because it is an indicator of catabolic factors in PRP that have been correlated with WBC concentrations.26
CONCLUSION
This study illustrated that growth factor and cytokine concentrations in both Llo PRP and Lhi PRP are stable for up to 4 hours. The clinical implications of these results suggest that if the generation or administration of PRP is delayed by up to 4 hours, the resultant PRP retains its bioactivity and is acceptable for clinical application. However, given the known variability of PRP generated due to patient and manufacturer variability,13,14 it is still important to ensure that the product is indeed PRP, with an increase in platelet number over the starting sample of blood. This validation can be performed with a simple and cost-effective manual smear analysis of blood and PRP. The results of this study provide information that can be immediately translated into clinical, surgical, and research practices.
ABSTRACT
The aim of this study was to provide clinical recommendations about the use of platelet-rich plasma (PRP) that was subjected to short-term storage at room temperature. We determined bioactive growth factor and cytokine concentrations as indicators of platelet and white blood cell degranulation in blood and PRP. Additionally, this study sought to validate the use of manual, direct smear analysis as an alternative to automated methods for platelet quantification in PRP.
Blood was used to generate low-leukocyte PRP (Llo PRP) or high-leukocyte PRP (Lhi PRP). Blood was either processed immediately or kept at room temperature for 2 or 4 hours prior to generation of PRP, which was then held at room temperature for 0, 1, 2, or 4 hours. Subsequently, bioactive transforming growth factor beta-1 and matrix metalloproteinase-9 were measured by ELISA (enzyme-linked immunosorbent assay). Manual and automated platelet counts were performed on all blood and PRP samples.
There were no differences in growth factor or cytokine concentration when blood or Llo PRP or Lhi PRP was retained at room temperature for up to 4 hours. Manual, direct smear analysis for platelet quantification was not different from the use of automated machine counting for PRP samples, but in the starting blood samples, manual platelet counts were significantly higher than those generated using automated technology.
When there is a delay of up to 4 hours in the generation of PRP from blood or in the application of PRP to the patient, bioactive growth factor and cytokine concentrations remain stable in both blood and PRP. A manual direct counting method is a simple, cost-effective, and valid method to measure the contents of the PRP product being delivered to the patient.
Platelet-rich plasma (PRP) is used to promote healing in many areas of medicine, such as dental surgery,1,2 soft-tissue injury,3,4 orthopedic surgery,5,6 wound healing,7 and veterinary medicine.8,9 Despite its extensive use, there are still questions about the clinical efficacy of PRP.10-12 Due to biological heterogeneity between patients13,14 and differences between available manufacturing kits,13,15 PRP can be highly variable between patients. There are classification schemes to categorize the various types of PRP,16-18 which can be divided broadly into low-leukocyte PRP (Llo PRP) and high-leukocyte PRP (Lhi PRP). PRP can be used as a point of care therapy, prepared and used immediately, or it can be used during a surgical procedure. In some institutions, blood is drawn by a phlebotomist, processed in the hospital laboratory, and then delivered to the operating room. In other instances, PRP is generated patient-side by the primary attending physician’s team, who draws the blood and processes it for immediate use.5,19 Delays at any step in these various scenarios could lead to the blood or the resultant PRP remaining at room temperature from minutes to several hours prior to administration to the patient. This variability in PRP protocols between clinical and surgical settings adds to concerns regarding the stability and efficacy of the biologic.
Continue to: When performing clinical or research...
When performing clinical or research studies using PRP, it is important to report the contents of the PRP delivered to the patient. By documenting the cellular content of the PRP delivered to the patient, the common questions of optimal platelet dose and the importance of leukocytes in PRP can begin to be answered. There are some known factors that contribute to PRP variability, such as patient biology and operator technique, but there are many other unknown factors. In some instances, there is a failure to generate PRP, defined as a lower platelet count in the PRP preparation than in the starting blood sample.13,14 To measure the platelet and cellular contents of the starting blood and PRP, samples can be submitted to a clinical pathology laboratory for a complete blood count, which adds cost to the patient above the typically unreimbursed cost of the PRP injection itself. An alternative method for measuring platelet concentrations is the use of direct smear analysis on glass slides. The use of direct smears to measure platelet concentration is well validated for blood,20,21 but the use of direct smears of PRP for determining platelet concentrations has not been previously validated. The use of manual platelet counts would provide an alternative to automated platelet counting for clinical and preclinical research studies to characterize the type of PRP administered to the patient.
The primary aim of this study was to determine if retention of blood or PRP at room temperature for various time intervals had an effect on final growth factor or catabolic cytokine concentration. Bioactive transforming growth factor-β1 (TGF-β1) and matrix metalloproteinase-9 (MMP-9) were measured as representatives of growth factors and catabolic cytokines, respectively. The secondary aim was to identify if manual platelet counts were an accurate reflection of automated counts. The outcomes of these experiments should provide immediately relevant information for the clinical application of PRP.
MATERIALS AND METHODS
Blood Collection and Generation of PRP
Under Institutional Review Board approval, blood (105 mL) was collected from healthy human volunteers (N = 5) into a syringe containing acid citrate dextrose anticoagulant to a final concentration of 10% acid citrate dextrose. Three 15-mL aliquots of blood were used to generate Llo PRP (Autologous Conditioned Plasma Double Syringe, Arthrex) and three 20-mL aliquots were used to generate Lhi PRP (SmartPReP 2, Harvest Technologies) (Figure 1).
Automated and Manual Counts
Automated complete blood counts were performed by a board certified clinical pathologist in the clinical pathology department of Cornell University on all blood, Llo PRP, and Lhi PRP samples. A manual platelet count, using a modified Giemsa stain,22 was performed on smears of all blood and PRP samples (Video). Slides were scanned at 10x magnification to identify an area where many red blood cells were present while maintaining a clear field of view (Figure 2A). The magnification was then increased to 100x using oil immersion, and the total number of platelets was counted in 10 fields of view (Figure 2B).
Growth Factor and Catabolic Cytokine Measurements
Blood and PRP samples were thawed for ELISA (enzyme-linked immunosorbent assay) analysis. TGF-β1 concentration was determined using the TGF-β1 Emax ImmunoAssay System (Promega Corporation), which measures biologically active TGF-β1. We chose TGF-β1 because it is commonly measured in PRP studies as an anabolic cytokine with multiple effects on tissue healing. The functions of TGF-β1 include stimulation of undifferentiated mesenchymal cell proliferation; regulation of endothelial, fibroblast, and osteoblast mitogenesis; coordination of collagen synthesis; promotion of endothelial chemotaxis and angiogenesis; activation of extracellular matrix synthesis in cartilage; and reduction of the catabolic activity of interluekin-1 and MMPs.23-25 In addition, TGF-β1 concentration strongly correlates with platelet concentration.26 MMP-9 concentration was determined using the MMP-9 Biotrak Activity Assay (GE Healthcare Biosciences) which measures both active and pro- forms of MMP-9. In PRP, MMP-9 was measured as an indicator of white blood cell (WBC) concentration.26 A catabolic cytokine capable of degrading collagen,13,27 MMP-9 has been linked to poor healing.28 For both assays, samples were measured in duplicate using a multiple detection plate reader (Tecan Safire).
Continue to: Statistical Analysis...
Statistical Analysis
Data were tested for the normal distribution to determine the appropriate statistical test. Manual and automated platelet counts were compared to each other in whole blood, Llo PRP, and Lhi PRP samples using a paired t test. Bioactive TGF-β1 concentrations in blood, Llo PRP, and Lhi PRP, were compared using a Kruskal-Wallis one-way analysis of variance (ANOVA) with Dunn’s all-pairwise comparison. Bioactive and pro-MMP-9 concentrations measured in the retained blood or PRP samples were compared using a one-way ANOVA with Tukey’s all-pairwise comparison. Statistical analyses were performed using Statistix 9 software (Analytical Software). A P value of <0.05 was considered significant.
RESULTS
Validation of PRP
PRP, as defined by an increase in platelet concentration in PRP compared with blood, was successfully generated in all samples by both systems. There was an average 1.98 ± 0.14-fold increase in platelet concentration in Llo PRP and an average 3.06 ± 0.24-fold increase in Lhi PRP. Platelet concentration was significantly higher in Lhi PRP than in Llo PRP (P = 0.001). Compared to whole blood, WBC concentration was 0.47 ± 0.07-fold lower in Llo PRP and 1.98 ± 0.14-fold greater in Lhi PRP. Similar to platelets, WBCs were significantly greater in Lhi PRP than in Llo PRP (P = 0.02).
Bioactive TGF-β1 and MMP-9 Concentration in Blood Retained at Room Temperature
To reflect the clinical situation where blood would be drawn from a patient, but there would be a delay in processing the blood to generate PRP, blood samples were retained at room temperature for up to 4 hours prior to analysis. Neither bioactive TGF-β1 (Figure 3) nor bioactive/pro-MMP-9 concentrations (Figure 4) changed significantly over time when blood was retained at room temperature prior to centrifugation to generate PRP.
Bioactive TGF-β1 and MMP-9 Concentration in PRP Retained at Room Temperature
In order to mimic the clinical situation where PRP would be generated but might sit out prior to being administered to the patient, PRP samples were retained at room temperature for up to 4 hours prior to analysis. In these samples, bioactive TGF-β1 concentrations were not significantly different between PRP products analyzed immediately and those samples retained at room temperature for up to 4 hours (Figure 5).
Automatic vs Manual Platelet Count
Manual platelet counts were compared to automated platelet counts to determine if a manual platelet smear analysis could be a reliable method for analyzing PRP in clinical and pre-clinical studies. There was a significant difference between the automated and manual platelet counts in blood samples (Table) (P = 0.05, N = 5) with the manual platelet count having a higher average (99.1 thou/uL) platelet concentration than automated counts. Platelet clumping was identified in 2 automated counts, which falsely decreased platelet concentration by an unknown quantity. Manual platelet counts for both Llo PRP (n = 30) and Lhi PRP (n = 30) were not different from automated platelet counts. Platelet clumping was not reported on any manual platelet counts performed on PRP samples.
Table. Platelet Concentrations of Whole Blood, Llo PRP, and Lhi PRP (N = 5)
| |||||
| Automated Count | Manual Count | P Value | ||
| Mean ± SD | Range | Mean ± SD | Range |
|
Blood | 111.8 ± 59.5 | 54-202 | 210.9 ± 59.4 | 144-297 | 0.05 |
Llo PRP | 421.4 ± 132.8 | 319-620 | 410.1 ± 94.2 | 318-543 | 0.61 |
Lhi PRP | 634.4 ± 88.8 | 517-766 | 635.4 ± 176.6 | 491-933 | 0.99 |
A paired t test was performed to compare results obtained from an automated platelet count and those obtained from a manual count.
Abbreviations: Lhi PRP, high-leukocyte platelet-rich plasma; Llo PRP, low-leukocyte platelet-rich plasma; SD, standard deviation.
Continue to:The primary aim of this study...
DISCUSSION
The primary aim of this study was to improve the clinical use of PRP by characterizing changes that might occur due to extended preparation times. Physicians commonly question the stability of blood or PRP if it is retained at room temperature prior to being administered to the patient. Clinical recommendations to optimize PRP preparation can be derived from a better understanding of the stability of platelets and WBCs, which contribute to the anabolic and catabolic cytokines in PRP.
The results of this study suggest that platelets and WBCs remain stable in blood and both Llo PRP and Lhi PRP for up to 4 hours. The use of bioactive ELISAs to measure TGF-β1 and MMP-9 allows for determination of stability of the PRP product retained at room temperature for up to 4 hours. This provides a time buffer to allow for delays from either institutional logistics or unanticipated clinical delays, without adverse effects on the generation of the final PRP product. As with all biologics, there are many factors that contribute to variability, but a relatively short delay of up to 4 hours in either generation of PRP from blood or in administration of PRP to the patient does not appear to contribute to that variability. Similar studies have been performed on equine PRP and suggest that growth factor concentrations remain stable for up to 6 hours after preparation of PRP29 and in human PRP, which implies that although samples degrade over time, platelet integrity might be acceptable for clinical use for up to 5 days after preparation, particularly if stored in oxygen.30 In contrast to this study, neither of the previously published reports used assays to measure biological activity in the stored PRP. Regardless of the variability between the studies with respect to the type of PRP evaluated and the outcome measures used, all of the studies support the concept that PRP can be stored at room temperature for at least a few hours before clinical use.
Centrifugation of blood does not guarantee the generation of PRP.13,14 In some cases, platelet counts in PRP are similar to or even less than that in the starting whole blood sample. To determine whether a clinical outcome is attributed to PRP, it is vital to know the platelet concentration and, arguably, the WBC concentration in the blood used to generate PRP and in the PRP sample administered to the patient. The platelet concentration in blood and PRP samples can be quantified using automated or manual methods. The use of automated methods can add significant cost to a study or procedure. Manually evaluating a blood smear is an accepted, though more time consuming, method of analyzing cellular components of a blood sample. Depending on the standard operating procedure of the laboratory, manual smears are often done in conjunction with an automated count. This identifies abnormalities in cellular shape or size, or platelet clumping, which are not consistently recognized by automated methods. Manually evaluating a blood smear does take some training, but the material cost is very low, which has added value for clinical or preclinical research studies. Interestingly, the results of this study indicate that manual platelet counts in blood may be more accurate than the count generated from an automated counter because the automated platelet counts were falsely low due to platelet clumping. Platelet clumping can occur as early as 1 hour after blood collection, regardless of the type of anticoagulant used.31
LIMITATIONS
The sample size of this study was small. However, variability in PRP has been well documented in multiple other studies using slightly larger sample sizes.13,14,16 Another potential limitation of this study could be that only one growth factor, TGF-β1, and one catabolic cytokine, MMP-9, were used as surrogate measures to represent platelet and WBC stability, respectively. We chose TGF-β1 because it is correlated with platelet concentrations14,15,26 and MMP-9 because it is an indicator of catabolic factors in PRP that have been correlated with WBC concentrations.26
CONCLUSION
This study illustrated that growth factor and cytokine concentrations in both Llo PRP and Lhi PRP are stable for up to 4 hours. The clinical implications of these results suggest that if the generation or administration of PRP is delayed by up to 4 hours, the resultant PRP retains its bioactivity and is acceptable for clinical application. However, given the known variability of PRP generated due to patient and manufacturer variability,13,14 it is still important to ensure that the product is indeed PRP, with an increase in platelet number over the starting sample of blood. This validation can be performed with a simple and cost-effective manual smear analysis of blood and PRP. The results of this study provide information that can be immediately translated into clinical, surgical, and research practices.
1. Nikolidakis D, Jansen JA. The biology of platelet-rich plasma and its application in oral surgery: Literature review. Tissue Eng Part B Rev. 2008;14(3):249-258. doi:10.1089/ten.teb.2008.0062.
2. Sánchez AR, Sheridan PJ, Kupp LI. Is platelet-rich plasma the perfect enhancement factor? A current review. Int J Oral Maxillofac Implants. 2003;18(1):93-103.
3. Monto RR. Platelet rich plasma treatment for chronic achilles tendinosis. Foot Ankle Int. 2012;33(5):379-385. doi:10.3113/FAI.2012.0379.
4. Owens RF, Ginnetti J, Conti SF, Latona C. Clinical and magnetic resonance imaging outcomes following platelet rich plasma injection for chronic midsubstance Achilles tendinopathy. Foot ankle Int. 2011;32(11):1032-1039. doi:10.3113/FAI.2011.1032.
5. Sánchez M, Anitua E, Azofra J, Andía I, Padilla S, Mujika I. Comparison of surgically repaired achilles tendon tears using platelet-rich fibrin matrices. Am J Sports Med. 2007;35(2):245-251. doi:10.1177/0363546506294078.
6. Silva A, Sampaio R. Anatomic ACL reconstruction: does the platelet-rich plasma accelerate tendon healing? Knee Surg Sports Traumatol Arthrosc. 2009;17(6):676-682. doi:10.1007/s00167-009-0762-8.
7. Fréchette JP, Martineau I, Gagnon G. Platelet-rich plasmas: growth factor content and roles in wound healing. J Dent Res. 2005;84(5):434-439. doi:10.1177/154405910508400507.
8. Bosch G, René van Weeren P, Barneveld A, van Schie HTM. Computerised analysis of standardised ultrasonographic images to monitor the repair of surgically created core lesions in equine superficial digital flexor tendons following treatment with intratendinous platelet rich plasma or placebo. Vet J. 2011;187(1):92-98. doi:10.1016/j.tvjl.2009.10.014.
9. Torricelli P, Fini M, Filardo G, et al. Regenerative medicine for the treatment of musculoskeletal overuse injuries in competition horses. Int Orthop. 2011;35(10):1569-1576. doi:10.1007/s00264-011-1237-3.
10. Sampson S, Gerhardt M, Mandelbaum B. Platelet rich plasma injection grafts for musculoskeletal injuries: a review. Curr Rev Musculoskelet Med. 2008;1(3-4):165-174. doi:10.1007/s12178-008-9032-5.
11. Sheth U, Simunovic N, Klein G, et al. Efficacy of autologous platelet-rich plasma use for orthopaedic indications: a meta-analysis. J Bone Joint Surg Am. 2012;94(4):298-307. doi:10.2106/JBJS.K.00154.
12. Vannini F, Di Matteo B, Filardo G, Kon E, Marcacci M, Giannini S. Platelet-rich plasma for foot and ankle pathologies: a systematic review. Foot Ankle Surg. 2014;20(1):2-9. doi:10.1016/j.fas.2013.08.001.
13. Boswell SG, Cole BJ, Sundman EA, Karas V, Fortier LA. Platelet-rich plasma: a milieu of bioactive factors. Arthroscopy. 2012;28(3):429-439. doi:10.1016/j.arthro.2011.10.018.
14. Mazzocca AD, McCarthy MBR, Chowaniec DM, et al. Platelet-rich plasma differs according to preparation method and human variability. J Bone Joint Surg Am. 2012;94(4):308-316. doi:10.2106/JBJS.K.00430.
15. Castillo TN, Pouliot MA, Kim HJ, Dragoo JL. Comparison of growth factor and platelet concentration from commercial platelet-rich plasma separation systems. Am J Sports Med. 2011;39(2):266-271. doi:10.1177/0363546510387517.
16. Arnoczky SP, Sheibani-Rad S, Shebani-Rad S. The basic science of platelet-rich plasma (PRP): what clinicians need to know. Sports Med Arthrosc. 2013;21(4):180-185. doi:10.1097/JSA.0b013e3182999712.
17. Dohan Ehrenfest DM, Bielecki T, Corso M Del, Inchingolo F, Sammartino G. Shedding light in the controversial terminology for platelet-rich products: Platelet-rich plasma (PRP), platelet-rich fibrin (PRF), platelet-leukocyte gel (PLG), preparation rich in growth factors (PRGF), classification and commercialism. J Biomed Mater Res Part A. 2010;95A(4):1280-1282. doi:10.1002/jbm.a.32894.
18. Dohan Ehrenfest DM, Rasmusson L, Albrektsson T. Classification of platelet concentrates: from pure platelet-rich plasma (P-PRP) to leucocyte- and platelet-rich fibrin (L-PRF). Trends Biotechnol. 2009;27(3):158-167. doi:10.1016/j.tibtech.2008.11.009.
19. Everts PA, Knape JT, Weibrich G, et al. Platelet-rich plasma and platelet gel: a review. J Extra Corpor Technol. 2006;38(2):174-187.
20. Malok M, Titchener EH, Bridgers C, Lee BY, Bamberg R. Comparison of two platelet count estimation methodologies for peripheral blood smears. Clin Lab Sci. 2007;20(3):154-160.
21. Gulati G, Uppal G, Florea AD, Gong J. Detection of platelet clumps on peripheral blood smears by CellaVision DM96 System and Microscopic Review. Lab Med. 2014;45(4):368-371. doi:10.1309/LM604RQVKVLRFXOR.
22. Gulati G, Song J, Florea AD, Gong J. Purpose and criteria for blood smear scan, blood smear examination, and blood smear review. Ann Lab Med. 2013;33(1):1-7. doi:10.3343/alm.2013.33.1.1.
23. Barrientos S, Stojadinovic O, Golinko MS, Brem H, Tomic-Canic M. Perspective article: Growth factors and cytokines in wound healing. Wound Repair Regen. 2008;16(5):585-601. doi:10.1111/j.1524-475X.2008.00410.x.
24. Crane D, Everts P. Platelet rich plasma (PRP) matrix grafts. Pract Pain Manag. 2008;8(1):12-26.
25. Fortier LA, Barker JU, Strauss EJ, McCarrel TM, Cole BJ. The role of growth factors in cartilage repair. Clin Orthop Relat Res. 2011;469(10):2706-2715. doi:10.1007/s11999-011-1857-3.
26. Sundman EA, Cole BJ, Fortier LA. Growth factor and catabolic cytokine concentrations are influenced by the cellular composition of platelet-rich plasma. Am J Sports Med. 2011;39(10):2135-2140. doi:10.1177/0363546511417792.
27. Vu TH, Shipley JM, Bergers G, et al. MMP-9/gelatinase B is a key regulator of growth plate angiogenesis and apoptosis of hypertrophic chondrocytes. Cell. 1998;93(3):411-422.
28. Watelet JB, Demetter P, Claeys C, Van Cauwenberge P, Cuvelier C, Bachert C. Neutrophil-derived metalloproteinase-9 predicts healing quality after sinus surgery. Laryngoscope. 2005;115(1):56-61. doi:10.1097/01.mlg.0000150674.30237.3f.
29. Hauschild G, Geburek F, Gosheger G, et al. Short term storage stability at room temperature of two different platelet-rich plasma preparations from equine donors and potential impact on growth factor concentrations. BMC Vet Res. 2017;13(1):7. doi:10.1186/s12917-016-0920-4.
30. Moore GW, Maloney JC, Archer RA, et al. Platelet-rich plasma for tissue regeneration can be stored at room temperature for at least five days. Br J Biomed Sci. 2017;74(2):71-77. doi:10.1080/09674845.2016.1233792.
31. McShine RL, Sibinga S, Brozovic B. Differences between the effects of EDTA and citrate anticoagulants on platelet count and mean platelet volume. Clin Lab Haematol. 1990;12(3):277-285.
1. Nikolidakis D, Jansen JA. The biology of platelet-rich plasma and its application in oral surgery: Literature review. Tissue Eng Part B Rev. 2008;14(3):249-258. doi:10.1089/ten.teb.2008.0062.
2. Sánchez AR, Sheridan PJ, Kupp LI. Is platelet-rich plasma the perfect enhancement factor? A current review. Int J Oral Maxillofac Implants. 2003;18(1):93-103.
3. Monto RR. Platelet rich plasma treatment for chronic achilles tendinosis. Foot Ankle Int. 2012;33(5):379-385. doi:10.3113/FAI.2012.0379.
4. Owens RF, Ginnetti J, Conti SF, Latona C. Clinical and magnetic resonance imaging outcomes following platelet rich plasma injection for chronic midsubstance Achilles tendinopathy. Foot ankle Int. 2011;32(11):1032-1039. doi:10.3113/FAI.2011.1032.
5. Sánchez M, Anitua E, Azofra J, Andía I, Padilla S, Mujika I. Comparison of surgically repaired achilles tendon tears using platelet-rich fibrin matrices. Am J Sports Med. 2007;35(2):245-251. doi:10.1177/0363546506294078.
6. Silva A, Sampaio R. Anatomic ACL reconstruction: does the platelet-rich plasma accelerate tendon healing? Knee Surg Sports Traumatol Arthrosc. 2009;17(6):676-682. doi:10.1007/s00167-009-0762-8.
7. Fréchette JP, Martineau I, Gagnon G. Platelet-rich plasmas: growth factor content and roles in wound healing. J Dent Res. 2005;84(5):434-439. doi:10.1177/154405910508400507.
8. Bosch G, René van Weeren P, Barneveld A, van Schie HTM. Computerised analysis of standardised ultrasonographic images to monitor the repair of surgically created core lesions in equine superficial digital flexor tendons following treatment with intratendinous platelet rich plasma or placebo. Vet J. 2011;187(1):92-98. doi:10.1016/j.tvjl.2009.10.014.
9. Torricelli P, Fini M, Filardo G, et al. Regenerative medicine for the treatment of musculoskeletal overuse injuries in competition horses. Int Orthop. 2011;35(10):1569-1576. doi:10.1007/s00264-011-1237-3.
10. Sampson S, Gerhardt M, Mandelbaum B. Platelet rich plasma injection grafts for musculoskeletal injuries: a review. Curr Rev Musculoskelet Med. 2008;1(3-4):165-174. doi:10.1007/s12178-008-9032-5.
11. Sheth U, Simunovic N, Klein G, et al. Efficacy of autologous platelet-rich plasma use for orthopaedic indications: a meta-analysis. J Bone Joint Surg Am. 2012;94(4):298-307. doi:10.2106/JBJS.K.00154.
12. Vannini F, Di Matteo B, Filardo G, Kon E, Marcacci M, Giannini S. Platelet-rich plasma for foot and ankle pathologies: a systematic review. Foot Ankle Surg. 2014;20(1):2-9. doi:10.1016/j.fas.2013.08.001.
13. Boswell SG, Cole BJ, Sundman EA, Karas V, Fortier LA. Platelet-rich plasma: a milieu of bioactive factors. Arthroscopy. 2012;28(3):429-439. doi:10.1016/j.arthro.2011.10.018.
14. Mazzocca AD, McCarthy MBR, Chowaniec DM, et al. Platelet-rich plasma differs according to preparation method and human variability. J Bone Joint Surg Am. 2012;94(4):308-316. doi:10.2106/JBJS.K.00430.
15. Castillo TN, Pouliot MA, Kim HJ, Dragoo JL. Comparison of growth factor and platelet concentration from commercial platelet-rich plasma separation systems. Am J Sports Med. 2011;39(2):266-271. doi:10.1177/0363546510387517.
16. Arnoczky SP, Sheibani-Rad S, Shebani-Rad S. The basic science of platelet-rich plasma (PRP): what clinicians need to know. Sports Med Arthrosc. 2013;21(4):180-185. doi:10.1097/JSA.0b013e3182999712.
17. Dohan Ehrenfest DM, Bielecki T, Corso M Del, Inchingolo F, Sammartino G. Shedding light in the controversial terminology for platelet-rich products: Platelet-rich plasma (PRP), platelet-rich fibrin (PRF), platelet-leukocyte gel (PLG), preparation rich in growth factors (PRGF), classification and commercialism. J Biomed Mater Res Part A. 2010;95A(4):1280-1282. doi:10.1002/jbm.a.32894.
18. Dohan Ehrenfest DM, Rasmusson L, Albrektsson T. Classification of platelet concentrates: from pure platelet-rich plasma (P-PRP) to leucocyte- and platelet-rich fibrin (L-PRF). Trends Biotechnol. 2009;27(3):158-167. doi:10.1016/j.tibtech.2008.11.009.
19. Everts PA, Knape JT, Weibrich G, et al. Platelet-rich plasma and platelet gel: a review. J Extra Corpor Technol. 2006;38(2):174-187.
20. Malok M, Titchener EH, Bridgers C, Lee BY, Bamberg R. Comparison of two platelet count estimation methodologies for peripheral blood smears. Clin Lab Sci. 2007;20(3):154-160.
21. Gulati G, Uppal G, Florea AD, Gong J. Detection of platelet clumps on peripheral blood smears by CellaVision DM96 System and Microscopic Review. Lab Med. 2014;45(4):368-371. doi:10.1309/LM604RQVKVLRFXOR.
22. Gulati G, Song J, Florea AD, Gong J. Purpose and criteria for blood smear scan, blood smear examination, and blood smear review. Ann Lab Med. 2013;33(1):1-7. doi:10.3343/alm.2013.33.1.1.
23. Barrientos S, Stojadinovic O, Golinko MS, Brem H, Tomic-Canic M. Perspective article: Growth factors and cytokines in wound healing. Wound Repair Regen. 2008;16(5):585-601. doi:10.1111/j.1524-475X.2008.00410.x.
24. Crane D, Everts P. Platelet rich plasma (PRP) matrix grafts. Pract Pain Manag. 2008;8(1):12-26.
25. Fortier LA, Barker JU, Strauss EJ, McCarrel TM, Cole BJ. The role of growth factors in cartilage repair. Clin Orthop Relat Res. 2011;469(10):2706-2715. doi:10.1007/s11999-011-1857-3.
26. Sundman EA, Cole BJ, Fortier LA. Growth factor and catabolic cytokine concentrations are influenced by the cellular composition of platelet-rich plasma. Am J Sports Med. 2011;39(10):2135-2140. doi:10.1177/0363546511417792.
27. Vu TH, Shipley JM, Bergers G, et al. MMP-9/gelatinase B is a key regulator of growth plate angiogenesis and apoptosis of hypertrophic chondrocytes. Cell. 1998;93(3):411-422.
28. Watelet JB, Demetter P, Claeys C, Van Cauwenberge P, Cuvelier C, Bachert C. Neutrophil-derived metalloproteinase-9 predicts healing quality after sinus surgery. Laryngoscope. 2005;115(1):56-61. doi:10.1097/01.mlg.0000150674.30237.3f.
29. Hauschild G, Geburek F, Gosheger G, et al. Short term storage stability at room temperature of two different platelet-rich plasma preparations from equine donors and potential impact on growth factor concentrations. BMC Vet Res. 2017;13(1):7. doi:10.1186/s12917-016-0920-4.
30. Moore GW, Maloney JC, Archer RA, et al. Platelet-rich plasma for tissue regeneration can be stored at room temperature for at least five days. Br J Biomed Sci. 2017;74(2):71-77. doi:10.1080/09674845.2016.1233792.
31. McShine RL, Sibinga S, Brozovic B. Differences between the effects of EDTA and citrate anticoagulants on platelet count and mean platelet volume. Clin Lab Haematol. 1990;12(3):277-285.
TAKE-HOME POINTS
- Blood can be stored at room temperature for up to 4 hours before making PRP without loss in activity.
- PRP can be stored at room temperature for up to 4 hours before administration to a patient without loss in activity.
- Manual, direct smear analysis is as accurate as automated counting for measuring platelet concentration in PRP.
Transformation of Benign Giant Cell Tumor of Bone Into Epithelioid Angiosarcoma
Take-Home Points
- Malignant transformation of a benign GCT is extremely rare.
- It is difficult to distinguish between an early malignant transformation and an overlooked malignancy.
- The most common clinical presentation of transformation of GCT into malignancy is pain, often with swelling.
- Interval monitoring of GCTs may be necessary in patients with symptoms concerning for malignant transformation.
- Clinicians should maintain a high clinical suspicion for malignant transformation or late recurrence of GCT in a patient with new pain at the wound site.
Giant cell tumors (GCTs) of bone account for about 5% of all primary bone tumors in adults, with a predominance in the third decade in life.1 Clinically, GCT of bone often presents with pain, pathologic fracture, and/or soft- tissue expansion in the epiphysis of long bones. However, GCT of bone also has been reported in non-long bones, such as the talus and the calcaneus.2,3 Histologically, GCT of bone consists of neoplastic stromal cells, mononuclear histiocytic cells, and multinucleated giant cells that resemble osteoclasts.4 The radiologic appearance of GCT is often described as a lytic, eccentrically located bony lesion that extends near the articular surface in patients with closed physes. Many GCTs have aggressive radiologic features with possible extensive bony destruction and soft-tissue extension.
Although categorized as a benign lesion, GCT can be locally aggressive, with a variable local recurrence rate of 0% to 65%, depending on treatment modality and skeletal location. Given the aggressiveness of GCT of bone, recommendations for operative intervention include intralesional curettage with adjuvant therapy (eg, cryotherapy, phenol, argon beam, electrocautery) and placement of bone void fillers (eg, bone graft polymethylmethacrylate). Wide resection is recommended when the articular surface is no longer viable for reconstruction secondary to extensive destruction. Some authors have reported that surgical margin is the only risk factor in local recurrence,5,6 and thus complete resection may be needed for tumor eradication. In addition, about 3% of GCTs demonstrate benign pulmonary implants, which have been cited as cause of death in 16% to 25% of reported cases of pulmonary spread.7,8
The literature includes few reports of primary or secondary malignant transformation of GCT. Hutter and colleagues9 defined primary malignant GCT as GCT with sarcomatous tissue juxtaposed with zones of typical benign GCT cells. Secondary malignant GCT is a sarcomatous lesion at the site of a previously documented benign GCT. Secondary malignant GCT of bone histologically has been classified as a fibrosarcoma, malignant fibrous histiocytoma, or osteosarcoma transformation.10
Most malignant transformations of GCT of bone have been attributed to previous irradiation of the lesion.11,12 However, there are some case reports of benign bone GCT malignant transformation in situ without any other medical intervention. It was reported that non-radiation-induced secondary transformations occur relatively early after GCT treatment.13 During the early stages of tumor recurrence, however, it is difficult to distinguish between malignant transformation and primary disease overlooked as a result of sampling error.
We report a case of secondary malignant transformation of GCT of bone 11 years after surgical curettage, cryotherapy, and cementation without adjuvant radiation therapy. To our knowledge, this case report is the first to describe transformation of a nonirradiated benign GCT into an aggressive, high-grade epithelioid angiosarcoma, a very rare vascular bone tumor. The patient provided written informed consent for print and electronic publication of this case report.
Case Report
In July 2003, a 46-year-old woman presented with left heel pain of several months’ duration. Plain radiographs showed a nonaggressive-appearing lytic lesion of the superior aspect of the posterior calcaneal tuberosity with a small cortical incongruity along the superior margin of the lesion (Figures 1A-1D).
A postoperative splint was placed, and weight-bearing progressed over 6 weeks. The patient was followed at 2- to 3-month intervals over the first 5 postoperative years. She was able to work and perform activities of daily living, but her postoperative course was complicated by significant chronic pain in multiple extremities and long-term treatment by the chronic pain service. At no time did postoperative imaging—magnetic resonance imaging (MRI) at 6 years, whole-body bone scan at 7 years, plain radiographs at 10 years—show evidence of recurrence.
Radiographs showed stable postoperative changes with a small radiolucent area (with sclerotic rim) surrounding the cement-bone interface. Given its proximity to the Achilles tendon and more motion than usual at the wound site, the radiolucency likely was caused by small movements of the interface. The radiolucent area remained stable over a 15-month period.
Whole-body bone scan showed a small area of osteoblastic activity in the left calcaneus, consistent with inflammation surrounding the bone- cement interface, but the uptake was minor relative to other areas of signal, and there were no significant inflammatory reactive changes on MRI (Figures 3A, 3B).
Over 11 years, regular 6- to 12-month follow-up examinations revealed no significant changes in the left foot or in plain radiographs of the chest. In addition, physical examinations revealed no evidence of a palpable mass of the left foot.
In July 2014 (11 years after curettage and cementation), the patient presented to her pain clinic appointment with severe left foot pain. She said that, over a few weeks, she experienced a significant increase in pain and developed posterolateral foot swelling, which limited her ability to ambulate. Plain radiographs showed a significant soft-tissue prominence around the posterior calcaneus, increased lucency around the bone-cement interface in the calcaneus with elevation, and a cortical break of the superior margin of the posterior calcaneus (Figures 3C, 3D). MRI showed a large lobular mass in the calcaneus and surrounding soft tissue with T1 and T2 signal heterogeneity and enhancement after administration of gadolinium (Figures 4A-4D). There was a large extraosseous extension of the calcaneus-based mass laterally and superiorly with edema in the surrounding hindfoot region (Figure 4).
Physical examination revealed exquisite tenderness along the lateral and posterior aspects of the left hindfoot. The patient was unable to bear weight and had soft-tissue swelling throughout the foot and mid calf as well as a palpable mass in the posterior heel. She was otherwise neurovascularly intact through all distributions of the left lower extremity. It was unclear if the GCT of the calcaneus had recurred or if there was a new, secondary tumor. Given her severe pain and morbidity, the patient decided to proceed with open biopsy and a pathology-pending plan for possible amputation in the near future.
In August 2014, an open biopsy with intraoperative frozen evaluation yielded a diagnosis of malignant neoplasm not otherwise specified. Permanent sections showed a proliferation of malignant epithelioid cells with extensive necrosis, hemorrhage, and hemosiderin deposition but no multinucleated giant cells.
Transformation of the GCT into a high-grade epithelioid angiosarcoma prompted presentation of the patient’s case to a multidisciplinary board of physicians with a focused clinical practice in sarcoma management. The board included board-certified specialists in orthopedic oncology, pathology, musculoskeletal radiology, medical oncology, and radiation oncology. Although discussion included pre-resection use of neoadjuvant chemotherapy to evaluate for disease response, the patient’s severe pain led her to forgo this treatment and proceed directly to below-knee amputation.
Amputation revealed a 7.7-cm hemorrhagic necrotic mass composed of a highly cellular spindle and epithelioid malignancy with abundant hemosiderin deposition (Figure 5). In addition, several atypical mitotic figures and malignant multinucleated tumor giant cells were randomly scattered throughout the neoplasm.
At first follow-up, the patient reported significant pain relief and asked to begin titrating off her chronic pain medicine. Clinical staging, which involved performing whole-body positron emission tomography/computed tomography, revealed nothing concerning for metastases. When this report was being written, the patient was being monitored for recurrent disease in accordance with National Comprehensive Cancer Network guidelines. In the absence of residual sarcoma, our medical oncology team discussed adjuvant chemotherapy options with her. Subsequently, however, she proceeded only with observation and periodic imaging.
Discussion
Malignant transformation of a benign GCT is extremely rare, especially in cases in which the tumor bed has not previously undergone radiation therapy. Although the literature includes historical case reports, primary and secondary malignant GCTs comprise <9% of all GCTs.11,13,14 Primary bone epithelioid angiosarcoma is also extremely rare, especially in the calcaneus; only 1 case is described in the literature.15 In this article, we report on a benign GCT of bone that transformed into an epithelioid angiosarcoma more than a decade after the GCT was treated with curettage and cementation.
The fact that the malignant areas of a previous tumor may have been missed because of sampling error is important for benign GCT of bone in the early postoperative period, as distinguishing between early malignant transformation and an overlooked malignancy may not be possible. However, transformation is more likely the case when a benign GCT becomes a high-grade malignancy after a long disease-free interval. Several authors have indicated that a benign GCT tumor recurring with a secondary malignancy 2 to 5 years after initial GCT treatment suggests malignant transformation.16 Grote and colleagues10 compiled reports of malignant transformation of GCT of bone and described the clinicopathologic features of secondary malignant transformation of GCTs. The data they compiled and data from several other studies indicate a poor prognosis after malignant transformation of GCT; 4 years after diagnosis, mean survival is 40% to 50%.10,16 The most common clinical presentation of transformation of GCT into malignancy is pain, often with coincident swelling of the native wound bed. However, a few cases have been identified with radiologic imaging alone and without a period of clinical symptoms.16
To our knowledge, this case report is the first to describe a longitudinal assessment of the transformation of a benign GCT of bone into an epithelioid angiosarcoma. Whereas an earlier reported GCT of bone transformed into epithelioid angiosarcoma after irradiation,12 our patient’s GCT of bone transformed without irradiation. GCTs of bone are locally aggressive benign tumors and are relatively rare. Malignant transformation of a benign bone tumor a decade after initial, definitive treatment is concerning, especially given the poor prognosis after malignant transformation in this clinical scenario. Current adjuvant treatments have not changed the prognosis. The literature includes a wide variety of histologic transformations, including high-grade sarcomas, after a long disease-free interval. Although malignant transformation of benign GCTs is rare, clinicians should be aware of the potential. Interval monitoring of GCTs may be necessary in patients with symptoms concerning for malignant transformation—pain or swelling in the wound bed—and patients should know to immediately inform their physician of any changes in pain level or local wound bed. Clinicians should maintain a high clinical suspicion for malignant transformation or late recurrence of GCT in a patient with new pain at the site of a previously treated GCT of bone with a disease-free interval of several years.
1. Unni KK. Dahlin’s Bone Tumors: General Aspects and Data on 11,087 Cases. 5th ed. Philadelphia, PA: Lippincott-Raven; 1996.
2. Errani C, Ruggieri P, Asenzio MA, et al. Giant cell tumor of the extremity: a review of 349 cases from a single institution. Cancer Treat Rev. 2010;36(1):1-7.
3. Campanacci M, Baldini N, Boriani S, Sudanese A. Giant-cell tumor of bone. J Bone Joint Surg Am. 1987;69(1):106-114.
4. Werner M. Giant cell tumour of bone: morphological, biological and histogenetical aspects. Int Orthop. 2006;30(6):484-489.
5 Klenke FM, Wenger DE, Inwards CY, Rose PS, Sim FH. Recurrent giant cell tumor of long bones: analysis of surgical management. Clin Orthop Relat Res. 2011;469(4):1181-1187.
6. McDonald DJ, Sim FH, McLeod RA, Dahlin DC. Giant-cell tumor of bone. J Bone Joint Surg Am. 1986;68(2):235-242.
7. Kay RM, Eckardt JJ, Seeger LL, Mirra JM, Hak DJ. Pulmonary metastasis of benign giant cell tumor of bone. Six histologically confirmed cases, including one of spontaneous regression. Clin Orthop Relat Res. 1994;(302):219-230.
8. Maloney WJ, Vaughan LM, Jones HH, Ross J, Nagel DA. Benign metastasizing giant-cell tumor of bone. Report of three cases and review of the literature. Clin Orthop Relat Res. 1989;(243):208-215.
9. Hutter RV, Worcester JN Jr, Francis KC, Foote FW Jr, Stewart FW. Benign and malignant giant cell tumors of bone. A clinicopathological analysis of the natural history of the disease. Cancer. 1962;15:653-690.
10. Grote HJ, Braun M, Kalinski T, et al. Spontaneous malignant transformation of conventional giant cell tumor. Skeletal Radiol. 2004;33(3):169-175.
11. Rock MG, Sim FH, Unni KK, et al. Secondary malignant giant-cell tumor of bone. Clinicopathological assessment of nineteen patients. J Bone Joint Surg Am. 1986;68(7):1073-1079.
12. Mittal S, Goswami C, Kanoria N, Bhattacharya A. Post-irradiation angiosarcoma of bone. J Cancer Res Ther. 2007;3(2):96-99.
13. Bertoni F, Bacchini P, Staals EL. Malignancy in giant cell tumor of bone. Cancer. 2003;97(10):2520-2529.
14. Dahlin DC, Cupps RE, Johnson EW Jr. Giant-cell tumor: a study of 195 cases. Cancer. 1970;25(5):1061-1070.
15. Balaji GG, Arockiaraj JS, Roy AC, Deepak B. Primary epithelioid angiosarcoma of the calcaneum: a diagnostic dilemma. J Foot Ankle Surg. 2014;53(2):239-242.
16. Anract P, De Pinieux G, Cottias P, Pouillart P, Forest M, Tomeno B. Malignant giant-cell tumours of bone. Clinico-pathological types and prognosis: a review of 29 cases. Int Orthop. 1998;22(1):19-26.
Take-Home Points
- Malignant transformation of a benign GCT is extremely rare.
- It is difficult to distinguish between an early malignant transformation and an overlooked malignancy.
- The most common clinical presentation of transformation of GCT into malignancy is pain, often with swelling.
- Interval monitoring of GCTs may be necessary in patients with symptoms concerning for malignant transformation.
- Clinicians should maintain a high clinical suspicion for malignant transformation or late recurrence of GCT in a patient with new pain at the wound site.
Giant cell tumors (GCTs) of bone account for about 5% of all primary bone tumors in adults, with a predominance in the third decade in life.1 Clinically, GCT of bone often presents with pain, pathologic fracture, and/or soft- tissue expansion in the epiphysis of long bones. However, GCT of bone also has been reported in non-long bones, such as the talus and the calcaneus.2,3 Histologically, GCT of bone consists of neoplastic stromal cells, mononuclear histiocytic cells, and multinucleated giant cells that resemble osteoclasts.4 The radiologic appearance of GCT is often described as a lytic, eccentrically located bony lesion that extends near the articular surface in patients with closed physes. Many GCTs have aggressive radiologic features with possible extensive bony destruction and soft-tissue extension.
Although categorized as a benign lesion, GCT can be locally aggressive, with a variable local recurrence rate of 0% to 65%, depending on treatment modality and skeletal location. Given the aggressiveness of GCT of bone, recommendations for operative intervention include intralesional curettage with adjuvant therapy (eg, cryotherapy, phenol, argon beam, electrocautery) and placement of bone void fillers (eg, bone graft polymethylmethacrylate). Wide resection is recommended when the articular surface is no longer viable for reconstruction secondary to extensive destruction. Some authors have reported that surgical margin is the only risk factor in local recurrence,5,6 and thus complete resection may be needed for tumor eradication. In addition, about 3% of GCTs demonstrate benign pulmonary implants, which have been cited as cause of death in 16% to 25% of reported cases of pulmonary spread.7,8
The literature includes few reports of primary or secondary malignant transformation of GCT. Hutter and colleagues9 defined primary malignant GCT as GCT with sarcomatous tissue juxtaposed with zones of typical benign GCT cells. Secondary malignant GCT is a sarcomatous lesion at the site of a previously documented benign GCT. Secondary malignant GCT of bone histologically has been classified as a fibrosarcoma, malignant fibrous histiocytoma, or osteosarcoma transformation.10
Most malignant transformations of GCT of bone have been attributed to previous irradiation of the lesion.11,12 However, there are some case reports of benign bone GCT malignant transformation in situ without any other medical intervention. It was reported that non-radiation-induced secondary transformations occur relatively early after GCT treatment.13 During the early stages of tumor recurrence, however, it is difficult to distinguish between malignant transformation and primary disease overlooked as a result of sampling error.
We report a case of secondary malignant transformation of GCT of bone 11 years after surgical curettage, cryotherapy, and cementation without adjuvant radiation therapy. To our knowledge, this case report is the first to describe transformation of a nonirradiated benign GCT into an aggressive, high-grade epithelioid angiosarcoma, a very rare vascular bone tumor. The patient provided written informed consent for print and electronic publication of this case report.
Case Report
In July 2003, a 46-year-old woman presented with left heel pain of several months’ duration. Plain radiographs showed a nonaggressive-appearing lytic lesion of the superior aspect of the posterior calcaneal tuberosity with a small cortical incongruity along the superior margin of the lesion (Figures 1A-1D).
A postoperative splint was placed, and weight-bearing progressed over 6 weeks. The patient was followed at 2- to 3-month intervals over the first 5 postoperative years. She was able to work and perform activities of daily living, but her postoperative course was complicated by significant chronic pain in multiple extremities and long-term treatment by the chronic pain service. At no time did postoperative imaging—magnetic resonance imaging (MRI) at 6 years, whole-body bone scan at 7 years, plain radiographs at 10 years—show evidence of recurrence.
Radiographs showed stable postoperative changes with a small radiolucent area (with sclerotic rim) surrounding the cement-bone interface. Given its proximity to the Achilles tendon and more motion than usual at the wound site, the radiolucency likely was caused by small movements of the interface. The radiolucent area remained stable over a 15-month period.
Whole-body bone scan showed a small area of osteoblastic activity in the left calcaneus, consistent with inflammation surrounding the bone- cement interface, but the uptake was minor relative to other areas of signal, and there were no significant inflammatory reactive changes on MRI (Figures 3A, 3B).
Over 11 years, regular 6- to 12-month follow-up examinations revealed no significant changes in the left foot or in plain radiographs of the chest. In addition, physical examinations revealed no evidence of a palpable mass of the left foot.
In July 2014 (11 years after curettage and cementation), the patient presented to her pain clinic appointment with severe left foot pain. She said that, over a few weeks, she experienced a significant increase in pain and developed posterolateral foot swelling, which limited her ability to ambulate. Plain radiographs showed a significant soft-tissue prominence around the posterior calcaneus, increased lucency around the bone-cement interface in the calcaneus with elevation, and a cortical break of the superior margin of the posterior calcaneus (Figures 3C, 3D). MRI showed a large lobular mass in the calcaneus and surrounding soft tissue with T1 and T2 signal heterogeneity and enhancement after administration of gadolinium (Figures 4A-4D). There was a large extraosseous extension of the calcaneus-based mass laterally and superiorly with edema in the surrounding hindfoot region (Figure 4).
Physical examination revealed exquisite tenderness along the lateral and posterior aspects of the left hindfoot. The patient was unable to bear weight and had soft-tissue swelling throughout the foot and mid calf as well as a palpable mass in the posterior heel. She was otherwise neurovascularly intact through all distributions of the left lower extremity. It was unclear if the GCT of the calcaneus had recurred or if there was a new, secondary tumor. Given her severe pain and morbidity, the patient decided to proceed with open biopsy and a pathology-pending plan for possible amputation in the near future.
In August 2014, an open biopsy with intraoperative frozen evaluation yielded a diagnosis of malignant neoplasm not otherwise specified. Permanent sections showed a proliferation of malignant epithelioid cells with extensive necrosis, hemorrhage, and hemosiderin deposition but no multinucleated giant cells.
Transformation of the GCT into a high-grade epithelioid angiosarcoma prompted presentation of the patient’s case to a multidisciplinary board of physicians with a focused clinical practice in sarcoma management. The board included board-certified specialists in orthopedic oncology, pathology, musculoskeletal radiology, medical oncology, and radiation oncology. Although discussion included pre-resection use of neoadjuvant chemotherapy to evaluate for disease response, the patient’s severe pain led her to forgo this treatment and proceed directly to below-knee amputation.
Amputation revealed a 7.7-cm hemorrhagic necrotic mass composed of a highly cellular spindle and epithelioid malignancy with abundant hemosiderin deposition (Figure 5). In addition, several atypical mitotic figures and malignant multinucleated tumor giant cells were randomly scattered throughout the neoplasm.
At first follow-up, the patient reported significant pain relief and asked to begin titrating off her chronic pain medicine. Clinical staging, which involved performing whole-body positron emission tomography/computed tomography, revealed nothing concerning for metastases. When this report was being written, the patient was being monitored for recurrent disease in accordance with National Comprehensive Cancer Network guidelines. In the absence of residual sarcoma, our medical oncology team discussed adjuvant chemotherapy options with her. Subsequently, however, she proceeded only with observation and periodic imaging.
Discussion
Malignant transformation of a benign GCT is extremely rare, especially in cases in which the tumor bed has not previously undergone radiation therapy. Although the literature includes historical case reports, primary and secondary malignant GCTs comprise <9% of all GCTs.11,13,14 Primary bone epithelioid angiosarcoma is also extremely rare, especially in the calcaneus; only 1 case is described in the literature.15 In this article, we report on a benign GCT of bone that transformed into an epithelioid angiosarcoma more than a decade after the GCT was treated with curettage and cementation.
The fact that the malignant areas of a previous tumor may have been missed because of sampling error is important for benign GCT of bone in the early postoperative period, as distinguishing between early malignant transformation and an overlooked malignancy may not be possible. However, transformation is more likely the case when a benign GCT becomes a high-grade malignancy after a long disease-free interval. Several authors have indicated that a benign GCT tumor recurring with a secondary malignancy 2 to 5 years after initial GCT treatment suggests malignant transformation.16 Grote and colleagues10 compiled reports of malignant transformation of GCT of bone and described the clinicopathologic features of secondary malignant transformation of GCTs. The data they compiled and data from several other studies indicate a poor prognosis after malignant transformation of GCT; 4 years after diagnosis, mean survival is 40% to 50%.10,16 The most common clinical presentation of transformation of GCT into malignancy is pain, often with coincident swelling of the native wound bed. However, a few cases have been identified with radiologic imaging alone and without a period of clinical symptoms.16
To our knowledge, this case report is the first to describe a longitudinal assessment of the transformation of a benign GCT of bone into an epithelioid angiosarcoma. Whereas an earlier reported GCT of bone transformed into epithelioid angiosarcoma after irradiation,12 our patient’s GCT of bone transformed without irradiation. GCTs of bone are locally aggressive benign tumors and are relatively rare. Malignant transformation of a benign bone tumor a decade after initial, definitive treatment is concerning, especially given the poor prognosis after malignant transformation in this clinical scenario. Current adjuvant treatments have not changed the prognosis. The literature includes a wide variety of histologic transformations, including high-grade sarcomas, after a long disease-free interval. Although malignant transformation of benign GCTs is rare, clinicians should be aware of the potential. Interval monitoring of GCTs may be necessary in patients with symptoms concerning for malignant transformation—pain or swelling in the wound bed—and patients should know to immediately inform their physician of any changes in pain level or local wound bed. Clinicians should maintain a high clinical suspicion for malignant transformation or late recurrence of GCT in a patient with new pain at the site of a previously treated GCT of bone with a disease-free interval of several years.
Take-Home Points
- Malignant transformation of a benign GCT is extremely rare.
- It is difficult to distinguish between an early malignant transformation and an overlooked malignancy.
- The most common clinical presentation of transformation of GCT into malignancy is pain, often with swelling.
- Interval monitoring of GCTs may be necessary in patients with symptoms concerning for malignant transformation.
- Clinicians should maintain a high clinical suspicion for malignant transformation or late recurrence of GCT in a patient with new pain at the wound site.
Giant cell tumors (GCTs) of bone account for about 5% of all primary bone tumors in adults, with a predominance in the third decade in life.1 Clinically, GCT of bone often presents with pain, pathologic fracture, and/or soft- tissue expansion in the epiphysis of long bones. However, GCT of bone also has been reported in non-long bones, such as the talus and the calcaneus.2,3 Histologically, GCT of bone consists of neoplastic stromal cells, mononuclear histiocytic cells, and multinucleated giant cells that resemble osteoclasts.4 The radiologic appearance of GCT is often described as a lytic, eccentrically located bony lesion that extends near the articular surface in patients with closed physes. Many GCTs have aggressive radiologic features with possible extensive bony destruction and soft-tissue extension.
Although categorized as a benign lesion, GCT can be locally aggressive, with a variable local recurrence rate of 0% to 65%, depending on treatment modality and skeletal location. Given the aggressiveness of GCT of bone, recommendations for operative intervention include intralesional curettage with adjuvant therapy (eg, cryotherapy, phenol, argon beam, electrocautery) and placement of bone void fillers (eg, bone graft polymethylmethacrylate). Wide resection is recommended when the articular surface is no longer viable for reconstruction secondary to extensive destruction. Some authors have reported that surgical margin is the only risk factor in local recurrence,5,6 and thus complete resection may be needed for tumor eradication. In addition, about 3% of GCTs demonstrate benign pulmonary implants, which have been cited as cause of death in 16% to 25% of reported cases of pulmonary spread.7,8
The literature includes few reports of primary or secondary malignant transformation of GCT. Hutter and colleagues9 defined primary malignant GCT as GCT with sarcomatous tissue juxtaposed with zones of typical benign GCT cells. Secondary malignant GCT is a sarcomatous lesion at the site of a previously documented benign GCT. Secondary malignant GCT of bone histologically has been classified as a fibrosarcoma, malignant fibrous histiocytoma, or osteosarcoma transformation.10
Most malignant transformations of GCT of bone have been attributed to previous irradiation of the lesion.11,12 However, there are some case reports of benign bone GCT malignant transformation in situ without any other medical intervention. It was reported that non-radiation-induced secondary transformations occur relatively early after GCT treatment.13 During the early stages of tumor recurrence, however, it is difficult to distinguish between malignant transformation and primary disease overlooked as a result of sampling error.
We report a case of secondary malignant transformation of GCT of bone 11 years after surgical curettage, cryotherapy, and cementation without adjuvant radiation therapy. To our knowledge, this case report is the first to describe transformation of a nonirradiated benign GCT into an aggressive, high-grade epithelioid angiosarcoma, a very rare vascular bone tumor. The patient provided written informed consent for print and electronic publication of this case report.
Case Report
In July 2003, a 46-year-old woman presented with left heel pain of several months’ duration. Plain radiographs showed a nonaggressive-appearing lytic lesion of the superior aspect of the posterior calcaneal tuberosity with a small cortical incongruity along the superior margin of the lesion (Figures 1A-1D).
A postoperative splint was placed, and weight-bearing progressed over 6 weeks. The patient was followed at 2- to 3-month intervals over the first 5 postoperative years. She was able to work and perform activities of daily living, but her postoperative course was complicated by significant chronic pain in multiple extremities and long-term treatment by the chronic pain service. At no time did postoperative imaging—magnetic resonance imaging (MRI) at 6 years, whole-body bone scan at 7 years, plain radiographs at 10 years—show evidence of recurrence.
Radiographs showed stable postoperative changes with a small radiolucent area (with sclerotic rim) surrounding the cement-bone interface. Given its proximity to the Achilles tendon and more motion than usual at the wound site, the radiolucency likely was caused by small movements of the interface. The radiolucent area remained stable over a 15-month period.
Whole-body bone scan showed a small area of osteoblastic activity in the left calcaneus, consistent with inflammation surrounding the bone- cement interface, but the uptake was minor relative to other areas of signal, and there were no significant inflammatory reactive changes on MRI (Figures 3A, 3B).
Over 11 years, regular 6- to 12-month follow-up examinations revealed no significant changes in the left foot or in plain radiographs of the chest. In addition, physical examinations revealed no evidence of a palpable mass of the left foot.
In July 2014 (11 years after curettage and cementation), the patient presented to her pain clinic appointment with severe left foot pain. She said that, over a few weeks, she experienced a significant increase in pain and developed posterolateral foot swelling, which limited her ability to ambulate. Plain radiographs showed a significant soft-tissue prominence around the posterior calcaneus, increased lucency around the bone-cement interface in the calcaneus with elevation, and a cortical break of the superior margin of the posterior calcaneus (Figures 3C, 3D). MRI showed a large lobular mass in the calcaneus and surrounding soft tissue with T1 and T2 signal heterogeneity and enhancement after administration of gadolinium (Figures 4A-4D). There was a large extraosseous extension of the calcaneus-based mass laterally and superiorly with edema in the surrounding hindfoot region (Figure 4).
Physical examination revealed exquisite tenderness along the lateral and posterior aspects of the left hindfoot. The patient was unable to bear weight and had soft-tissue swelling throughout the foot and mid calf as well as a palpable mass in the posterior heel. She was otherwise neurovascularly intact through all distributions of the left lower extremity. It was unclear if the GCT of the calcaneus had recurred or if there was a new, secondary tumor. Given her severe pain and morbidity, the patient decided to proceed with open biopsy and a pathology-pending plan for possible amputation in the near future.
In August 2014, an open biopsy with intraoperative frozen evaluation yielded a diagnosis of malignant neoplasm not otherwise specified. Permanent sections showed a proliferation of malignant epithelioid cells with extensive necrosis, hemorrhage, and hemosiderin deposition but no multinucleated giant cells.
Transformation of the GCT into a high-grade epithelioid angiosarcoma prompted presentation of the patient’s case to a multidisciplinary board of physicians with a focused clinical practice in sarcoma management. The board included board-certified specialists in orthopedic oncology, pathology, musculoskeletal radiology, medical oncology, and radiation oncology. Although discussion included pre-resection use of neoadjuvant chemotherapy to evaluate for disease response, the patient’s severe pain led her to forgo this treatment and proceed directly to below-knee amputation.
Amputation revealed a 7.7-cm hemorrhagic necrotic mass composed of a highly cellular spindle and epithelioid malignancy with abundant hemosiderin deposition (Figure 5). In addition, several atypical mitotic figures and malignant multinucleated tumor giant cells were randomly scattered throughout the neoplasm.
At first follow-up, the patient reported significant pain relief and asked to begin titrating off her chronic pain medicine. Clinical staging, which involved performing whole-body positron emission tomography/computed tomography, revealed nothing concerning for metastases. When this report was being written, the patient was being monitored for recurrent disease in accordance with National Comprehensive Cancer Network guidelines. In the absence of residual sarcoma, our medical oncology team discussed adjuvant chemotherapy options with her. Subsequently, however, she proceeded only with observation and periodic imaging.
Discussion
Malignant transformation of a benign GCT is extremely rare, especially in cases in which the tumor bed has not previously undergone radiation therapy. Although the literature includes historical case reports, primary and secondary malignant GCTs comprise <9% of all GCTs.11,13,14 Primary bone epithelioid angiosarcoma is also extremely rare, especially in the calcaneus; only 1 case is described in the literature.15 In this article, we report on a benign GCT of bone that transformed into an epithelioid angiosarcoma more than a decade after the GCT was treated with curettage and cementation.
The fact that the malignant areas of a previous tumor may have been missed because of sampling error is important for benign GCT of bone in the early postoperative period, as distinguishing between early malignant transformation and an overlooked malignancy may not be possible. However, transformation is more likely the case when a benign GCT becomes a high-grade malignancy after a long disease-free interval. Several authors have indicated that a benign GCT tumor recurring with a secondary malignancy 2 to 5 years after initial GCT treatment suggests malignant transformation.16 Grote and colleagues10 compiled reports of malignant transformation of GCT of bone and described the clinicopathologic features of secondary malignant transformation of GCTs. The data they compiled and data from several other studies indicate a poor prognosis after malignant transformation of GCT; 4 years after diagnosis, mean survival is 40% to 50%.10,16 The most common clinical presentation of transformation of GCT into malignancy is pain, often with coincident swelling of the native wound bed. However, a few cases have been identified with radiologic imaging alone and without a period of clinical symptoms.16
To our knowledge, this case report is the first to describe a longitudinal assessment of the transformation of a benign GCT of bone into an epithelioid angiosarcoma. Whereas an earlier reported GCT of bone transformed into epithelioid angiosarcoma after irradiation,12 our patient’s GCT of bone transformed without irradiation. GCTs of bone are locally aggressive benign tumors and are relatively rare. Malignant transformation of a benign bone tumor a decade after initial, definitive treatment is concerning, especially given the poor prognosis after malignant transformation in this clinical scenario. Current adjuvant treatments have not changed the prognosis. The literature includes a wide variety of histologic transformations, including high-grade sarcomas, after a long disease-free interval. Although malignant transformation of benign GCTs is rare, clinicians should be aware of the potential. Interval monitoring of GCTs may be necessary in patients with symptoms concerning for malignant transformation—pain or swelling in the wound bed—and patients should know to immediately inform their physician of any changes in pain level or local wound bed. Clinicians should maintain a high clinical suspicion for malignant transformation or late recurrence of GCT in a patient with new pain at the site of a previously treated GCT of bone with a disease-free interval of several years.
1. Unni KK. Dahlin’s Bone Tumors: General Aspects and Data on 11,087 Cases. 5th ed. Philadelphia, PA: Lippincott-Raven; 1996.
2. Errani C, Ruggieri P, Asenzio MA, et al. Giant cell tumor of the extremity: a review of 349 cases from a single institution. Cancer Treat Rev. 2010;36(1):1-7.
3. Campanacci M, Baldini N, Boriani S, Sudanese A. Giant-cell tumor of bone. J Bone Joint Surg Am. 1987;69(1):106-114.
4. Werner M. Giant cell tumour of bone: morphological, biological and histogenetical aspects. Int Orthop. 2006;30(6):484-489.
5 Klenke FM, Wenger DE, Inwards CY, Rose PS, Sim FH. Recurrent giant cell tumor of long bones: analysis of surgical management. Clin Orthop Relat Res. 2011;469(4):1181-1187.
6. McDonald DJ, Sim FH, McLeod RA, Dahlin DC. Giant-cell tumor of bone. J Bone Joint Surg Am. 1986;68(2):235-242.
7. Kay RM, Eckardt JJ, Seeger LL, Mirra JM, Hak DJ. Pulmonary metastasis of benign giant cell tumor of bone. Six histologically confirmed cases, including one of spontaneous regression. Clin Orthop Relat Res. 1994;(302):219-230.
8. Maloney WJ, Vaughan LM, Jones HH, Ross J, Nagel DA. Benign metastasizing giant-cell tumor of bone. Report of three cases and review of the literature. Clin Orthop Relat Res. 1989;(243):208-215.
9. Hutter RV, Worcester JN Jr, Francis KC, Foote FW Jr, Stewart FW. Benign and malignant giant cell tumors of bone. A clinicopathological analysis of the natural history of the disease. Cancer. 1962;15:653-690.
10. Grote HJ, Braun M, Kalinski T, et al. Spontaneous malignant transformation of conventional giant cell tumor. Skeletal Radiol. 2004;33(3):169-175.
11. Rock MG, Sim FH, Unni KK, et al. Secondary malignant giant-cell tumor of bone. Clinicopathological assessment of nineteen patients. J Bone Joint Surg Am. 1986;68(7):1073-1079.
12. Mittal S, Goswami C, Kanoria N, Bhattacharya A. Post-irradiation angiosarcoma of bone. J Cancer Res Ther. 2007;3(2):96-99.
13. Bertoni F, Bacchini P, Staals EL. Malignancy in giant cell tumor of bone. Cancer. 2003;97(10):2520-2529.
14. Dahlin DC, Cupps RE, Johnson EW Jr. Giant-cell tumor: a study of 195 cases. Cancer. 1970;25(5):1061-1070.
15. Balaji GG, Arockiaraj JS, Roy AC, Deepak B. Primary epithelioid angiosarcoma of the calcaneum: a diagnostic dilemma. J Foot Ankle Surg. 2014;53(2):239-242.
16. Anract P, De Pinieux G, Cottias P, Pouillart P, Forest M, Tomeno B. Malignant giant-cell tumours of bone. Clinico-pathological types and prognosis: a review of 29 cases. Int Orthop. 1998;22(1):19-26.
1. Unni KK. Dahlin’s Bone Tumors: General Aspects and Data on 11,087 Cases. 5th ed. Philadelphia, PA: Lippincott-Raven; 1996.
2. Errani C, Ruggieri P, Asenzio MA, et al. Giant cell tumor of the extremity: a review of 349 cases from a single institution. Cancer Treat Rev. 2010;36(1):1-7.
3. Campanacci M, Baldini N, Boriani S, Sudanese A. Giant-cell tumor of bone. J Bone Joint Surg Am. 1987;69(1):106-114.
4. Werner M. Giant cell tumour of bone: morphological, biological and histogenetical aspects. Int Orthop. 2006;30(6):484-489.
5 Klenke FM, Wenger DE, Inwards CY, Rose PS, Sim FH. Recurrent giant cell tumor of long bones: analysis of surgical management. Clin Orthop Relat Res. 2011;469(4):1181-1187.
6. McDonald DJ, Sim FH, McLeod RA, Dahlin DC. Giant-cell tumor of bone. J Bone Joint Surg Am. 1986;68(2):235-242.
7. Kay RM, Eckardt JJ, Seeger LL, Mirra JM, Hak DJ. Pulmonary metastasis of benign giant cell tumor of bone. Six histologically confirmed cases, including one of spontaneous regression. Clin Orthop Relat Res. 1994;(302):219-230.
8. Maloney WJ, Vaughan LM, Jones HH, Ross J, Nagel DA. Benign metastasizing giant-cell tumor of bone. Report of three cases and review of the literature. Clin Orthop Relat Res. 1989;(243):208-215.
9. Hutter RV, Worcester JN Jr, Francis KC, Foote FW Jr, Stewart FW. Benign and malignant giant cell tumors of bone. A clinicopathological analysis of the natural history of the disease. Cancer. 1962;15:653-690.
10. Grote HJ, Braun M, Kalinski T, et al. Spontaneous malignant transformation of conventional giant cell tumor. Skeletal Radiol. 2004;33(3):169-175.
11. Rock MG, Sim FH, Unni KK, et al. Secondary malignant giant-cell tumor of bone. Clinicopathological assessment of nineteen patients. J Bone Joint Surg Am. 1986;68(7):1073-1079.
12. Mittal S, Goswami C, Kanoria N, Bhattacharya A. Post-irradiation angiosarcoma of bone. J Cancer Res Ther. 2007;3(2):96-99.
13. Bertoni F, Bacchini P, Staals EL. Malignancy in giant cell tumor of bone. Cancer. 2003;97(10):2520-2529.
14. Dahlin DC, Cupps RE, Johnson EW Jr. Giant-cell tumor: a study of 195 cases. Cancer. 1970;25(5):1061-1070.
15. Balaji GG, Arockiaraj JS, Roy AC, Deepak B. Primary epithelioid angiosarcoma of the calcaneum: a diagnostic dilemma. J Foot Ankle Surg. 2014;53(2):239-242.
16. Anract P, De Pinieux G, Cottias P, Pouillart P, Forest M, Tomeno B. Malignant giant-cell tumours of bone. Clinico-pathological types and prognosis: a review of 29 cases. Int Orthop. 1998;22(1):19-26.
Application of Amniotic Tissue in Orthopedic Surgery
The amniotic membrane is a multilayer tissue forming the innermost layer of the amniotic sac that surrounds the developing fetus. It is comprised of 5 layers, from the inside out: a single layer of epithelial cells, a thick basement membrane, a compact layer, a fibroblast layer, and a spongy layer that abuts the surrounding chorion (Figure 1).1
Amniotic epithelial cells are derived from the pluripotent epiblast at approximately day 8 of gestation. This is well before gastrulation occurs at days 15 to 17, considered the “tipping point” when pluripotent cells differentiate into ectoderm, mesoderm, and endoderm.3 These cells express Oct-4 and Nanog, 2 molecular markers that are indicative of pluripotency.3 Two cell types have been identified in amniotic tissues that possess stem cell-like characteristics: human amniotic epithelial cells and human amniotic mesenchymal stromal cells.4 Both of these cell types have demonstrated the ability to differentiate into various cell lineages, including endothelial cells, adipocytes, myogenic cells, neurogenic cells, chondrocytes, tenocytes, and osteogenic cells.5-7 These previously reported findings indicate that amniotic cells and tissue have the capability to generate mesenchymal tissues.
FDA Classification and Available Forms
The US Food and Drug Administration (FDA) classifies amnion as an allograft tissue under Human Cells, Tissues, and Cellular and Tissue-Based Products (HCT/Ps) 361. To meet criteria, the tissue needs to be minimally manipulated. It is to be for homologous use and cannot be combined with other cells or tissues. There can be no systemic effect or dependence on the metabolic activity of living cells to achieve its primary function. The tissue has to have a localized effect in vivo. Therefore, amnion allograft tissue can be commercialized, provided it is not marketed as a stem cell product or to contain viable cells.
Amniotic tissue is commercially available in several forms.
Safety
Amniotic tissue has been used for over 100 years in burn, ophthalmology, and chronic wound patients with favorable outcomes and no adverse effects reported in the literature. Unlike embryonic stem cells, which may be tumorigenic,8 amniotic cells do not possess any known tumorigenicity.9 In one study, 50 immunodeficient mice were injected with 1 to 2 million amniotic epithelial cells and observed for a maximum of 516 days with no tumorigenicity observed in any of the animals.10 In another study, amniotic epithelial cells were implanted into the forearms of healthy volunteers and no immunologic response was observed in any of the recipients.11 Furthermore, viable amniotic cells were recovered via biopsy 7 weeks following transplantation, demonstrating viability of the transplanted cells.11 The lack of tumorigenicity and immunologic response in hosts is due in part to the fact that amniotic cells do not express human leukocyte antigen class II antigens and only express class I antigens in small amounts.3
Advantages of Amnion Tissue
Amniotic tissue is readily available, as it is often discarded after childbirth. The use of this tissue poses no added risk to the fetus or mother, eliminating the ethical concerns associated with obtaining embryonic stem cells. Amniotic tissue is comprised of an extracellular matrix, which acts as a natural scaffold for cellular attachment and structural support for cells as well as collagen types I, III, IV, V, and VI, hyaluronic acid, and a host of growth factors.12 In addition, it possesses antimicrobial properties, including beta-defensins.13
Amniotic tissue has been shown to exert an anti-inflammatory effect by inhibiting the inflammatory cascade. Specifically, it has been shown to inhibit cytokines such as tumor necrosis factor-alpha in the presence of dendritic cells,14 as well as inhibiting transforming growth factor-beta, interleukin-8, and fibroblast proliferation.15 These findings indicate that amniotic tissue has the ability to dampen the “cytokine storm” that occurs after an injury in an adult, which would lead to beneficial impacts on healing and scar formation in patients.16
Basic Science and Animal Studies
Several studies have demonstrated promising outcomes for orthopedic applications in vitro. A comparison of osteogenic potential found that amniotic fluid-derived cells were able to produce approximately 5 times more mineralized matrix than bone marrow-derived mesenchymal stem cells.17 More recently, Si and colleagues18 compared the osteogenic potential of human amniotic epithelial cells, amniotic cells, and human bone marrow-derived mesenchymal stem cells. They found that all 3 cell lines were osteogenic, though the amniotic epithelial cells had better immunomodulatory properties and marginally less osteogenic potential than the other 2 cell types. Furthermore, several in vivo animal studies have demonstrated the ability of human amniotic cells to stimulate bone growth in rats,19,20 rabbits,21 and sheep.22
Amniotic tissue also possesses potential for chondrogenesis. Cryopreserved human amniotic membrane cells used for in vitro human osteoarthritis tissue scaffolds did not differentiate in culture, and they integrated and repaired damaged articular cartilage.23 Various in vitro24,25 and animal in vivo26,27 studies have reported similar supportive findings. Kunisaki and colleagues28 used sheep amniotic fluid mesenchymal stem cells to reconstruct lamb tracheal cartilage in utero, concluding that cells obtained from the amniotic fluid possess chondrogenic capabilities. Further in utero lamb studies of cartilage artificial defects, given 7 days to settle before adding a hypocellular matrix as a scaffold, showed chondrocyte density and cell architecture was restored at the defect site after 28 days without the formation of an inflammatory response or scar tissue.29
Amniotic tissue has had similar success in tendon repair studies in vivo.9,30,31 Barboni and colleagues32 implanted amniotic epithelial cells (AECs) into artificially created sheep Achilles tendon defects in situ, inducing superior structural and mechanical recovery in the defects at a faster rate compared to controls not receiving AECs. Healing via AECs started at the healthy tissue around the borders of the defect and progressed centrally, suggesting recruitment of native progenitor cells to the lesion.32 Kueckelhaus and colleagues33 investigated the role of amnion-derived cellular cytokine solution in the healing of transections of rat Achilles tendons, reporting improved mechanical properties of healing tendons at early time points compared to controls. Beredjiklian and colleagues34 compared the healing of transected extensor tendons of pregnant ewes and of their fetus in utero, reporting a reparative form of healing with scar formation in adult subjects and regenerative form of healing without scar formation or inflammation in fetal subjects.
Amniotic tissue has properties that prevent adhesion formation around tendons following injury and reconstruction.35 Ozgenel36 investigated the effects of hyaluronic acid and amniotic membrane alone and in combination on the presence of adhesions and the rate of healing following chicken flexor tendon repair. The study found amniotic membrane wrapped around the repaired tendon was superior in preventing adhesion formation. Kim and colleagues37 report a similar reduction in fibrosis and adhesion following application of a human amniotic membrane wrap to rabbit ulnar neurorrhaphy sites.
This barrier function of amniotic tissue has also been investigated in the prevention of surgical scarring and peridural fibrosis in animal models following spinal discectomy. A study in canine models showed a reduction of scarring following the application of cross-linked amniotic membrane compared to freeze dried amniotic membrane.38 Similar reductions in scarring in rat models with the application of freeze-dried amniotic membrane compared to negative controls have been reported.39
Human Studies
A randomized trial investigated the outcomes of prenatal vs postnatal repair of myelomeningocele in humans, finding a reduced need for implanted shunts and improved functional outcomes at 30 months of life in the prenatal intervention group compared to the postnatal group.40 This study was concluded early due to the efficacy of prenatal surgery and the benefit of nervous system repair in utero in the presence of amniotic growth factors.
Vines and colleagues41 performed a 6-patient feasibility study using amnion injections to treat symptomatic knee osteoarthritis. Each patient received a single intra-articular cryopreserved amniotic suspension allograft (ASA) injection and was followed for 1 year. No adverse outcomes were reported, with the only abnormal finding being a small increase in serum immunoglobulin G and immunoglobulin E levels. Intra-articular ASA injection was found to be safe, but a large-scale trial investigating symptomatic relief was recommended.41
Most of the human studies using amnion pertain to foot and ankle surgery. Its use as a treatment for diabetic foot ulcers and recalcitrant plantar fasciitis was one of the early-recognized successes.42-45 Zelen and colleagues46 investigated the applications of injectable micronized dehydrated human amniotic/chorionic membrane as an alternative to surgical intervention in the treatment of refractory plantar fasciitis. This prospective, randomized trial with 45 patients showed significant improvement in plantar fasciitis symptoms at 8 weeks compared to controls (saline injections). A similar study compared the use of cryopreserved human amniotic membrane (c-hAM) injections to corticosteroid injections in plantar fasciitis patients.47 The results indicated that c-hAM is safe and comparable to corticosteroids, with the authors noting that pain improvement was greatest in patients receiving 2 injections of c-hAM at 18 weeks.
Tendon wrapping, in which the amniotic membrane is laid over a tendon repair, has been reported with success. Amniotic membrane is superior to collagen for tendon wrapping as it actively contributes to healing while minimizing adhesions, which collagen alone cannot do.48 The membrane serves as a protective sheath around repaired tendons with anti-inflammatory, anti-adhesive, immunomodulatory, and antimicrobial benefits. A 124-patient study demonstrated the safety of using amnion in this manner, and the authors reported a decreased rate of complication compared to previously published data.49 Another study of 14 patients undergoing foot and ankle surgery with tendon wrapping reported clinical improvement with reduced pain and greater functional outcomes postoperatively compared to preoperative measurements.50
Conclusion
Amniotic membrane-derived tissues are safe and non-tumorigenic, producing an abundance of growth factors that have shown promise as tissue scaffolds and as aids in the regeneration of human bone and soft tissues. Amnion applications in orthopedic surgery may be numerous, but development is ongoing. Given the vast array of in vitro and in vivo animal data supporting the benefits of amnion in tissue regeneration, orthopedic surgeons and researchers should place emphasis on conducting clinical studies to validate the safety and efficacy of amniotic cells in the treatment of orthopedic conditions.
Am J Orthop. 2016;45(7):E421-E425. Copyright Frontline Medical Communications Inc. 2016. All rights reserved.
1. Benirschke K, Kaufman P. Anatomy and pathology of the placental membranes. In: Pathology of the Human Placent., 4th ed. New York, NY: Springer-Verlag; 2000:281-334.
2. Mamede AC, Carvalho MJ, Abrantes AM, Laranjo M, Maia CJ, Botelho MF. Amniotic membrane: from structure and functions to clinical applications. Cell Tissue Res. 2012;349(2):447-458.
3. Miki T, Strom SC. Amnion-derived pluripotent/multipotent stem cells. Stem Cell Rev. 2006;2(2):133-142.
4. Parolini O, Alviano F, Bagnara GP, et al. Concise review: isolation and characterization of cells from human term placenta: outcome of the first international workshop on placenta derived stem cells. Stem Cells. 2008;26(2):300-311.
5. Ilancheran S, Michalska A, Peh G, Wallace EM, Pera M, Manuelpillai U. Stem cells derived from human fetal membranes display multilineage differentiation potential. Biol Reprod. 2007;77(3):577-588.
6. Alviano F, Fossati V, Marchionni C, et al. Term amniotic membrane is a high throughput source for multipotent mesenchymal stem cells with the ability to differentiate into endothelial cells in vitro. BMC Dev Biol. 2007;7:11.
7. Barboni B, Curini V, Russo V, et al. Indirect co-culture with tendons or tenocytes can program amniotic epithelial cells towards stepwise tenogenic differentiation. PLoS One. 2012;7(2):e30974.
8. Ben-David U, Benvenisty N. The tumorigenicity of human embryonic and induced pluripotent stem cells. Nature Reviews Cancer. 2011;11(4):268-277.
9. Lange-Consiglio A, Rossi D, Tassan S, Perego R, Cremonesi F, Parolini O. Conditioned medium from horse amniotic membrane-derived multipotent progenitor cells: immunomodulatory activity in vitro and first clinical application in tendon and ligament injuries in vivo. Stem Cells Dev. 2013;22(22):3015-3024.
10. Miki T. Amnion-derived stem cells: in quest of clinical applications. Stem Cell Res Ther. 2011;2(3):25.
11. Akle CA, Adinolfi M, Welsh KI, Leibowitz S, McColl I. Immunogenicity of human amniotic epithelial cells after transplantation into volunteers. Lancet. 1981;2(8254):1003-1035.
12. Gupta A, Kedige SD, Jain K. Amnion and chorion membranes: potential stem cell reservoir with wide applications in periodontics. Int J Biomater. 2015;2015:274082.
13. Buhimschi IA, Jabr M, Buhimschi CS, Petkova AP, Weiner CP, Saed GM. The novel antimicrobial peptide beta3-defensin is produced by the amnion: a possible role of the fetal membranes in innate immunity of the amniotic cavity. Am J Obstet Gynecol. 2004;191(5):1678-1687.
14. Magatti M, De Munari S, Vertua E, et al. Amniotic mesenchymal tissue cells inhibit dendritic cell differentiation of peripheral blood and amnion resident monocytes. Cell Transplant. 2009;18(8):899-914.
15. Solomon A, Wajngarten M, Alviano F, et al. Suppression of inflammatory and fibrotic responses in allergic inflammation by the amniotic membrane stromal matrix. Clin Exp Allergy. 2005;35(7):941-948.
16. Silini A, Parolini O, Huppertz B, Lang I. Soluble factors of amnion-derived cells in treatment of inflammatory and fibrotic pathologies. Curr Stem Cell Res Ther. 2013;8(1):6-14.
17. Peister A, Woodruff MA, Prince JJ, Gray DP, Hutmacher DW, Guldberg RE. Cell sourcing for bone tissue engineering: amniotic fluid stem cells have a delayed, robust differentiation compared to mesenchymal stem cells. Stem Cell Res. 2011;7(1):17-27.
18. Si J, Dai J, Zhang J, et al. Comparative investigation of human amniotic epithelial cells and mesenchymal stem cells for application in bone tissue engineering. Stem Cells Int. 2015;2015:565732.
19. Starecki M, Schwartz JA, Grande DA. Evaluation of amniotic-derived membrane biomaterial as an adjunct for repair of critical sized bone defects. Advances in Orthopedic Surgery. 2014;2014:572586.
20. Kerimoglu S, Livaoglu M, Sönmez B, et al. Effects of human amniotic fluid on fracture healing in rat tibia. J Surg Res. 2009;152(2):281-287.
21. Karaçal N, Kocucu P, Cobanglu U, Kutlu N. Effect of human amniotic fluid on bone healing. J Surg Res. 2005;129(2):283-287.
22. Barboni B, Mangano C, Valbonetti L, et al. Synthetic bone substitute engineered with amniotic epithelial cells enhances bone regeneration after maxillary sinus augmentation. PLoS One. 2013;8(5):e63256.
23. Díaz-Prado S, Rendal-Vázquez ME, Muiños-Lopez E, et al. Potential use of the human amniotic membrane as a scaffold in human articular cartilage repair. Cell Tissue Bank. 2010;11(2):183-195.
24. Krishnamurithy G, Shilpa PN, Ahmad RE, Sulaiman S, Ng CL, Kamarul T. Human amniotic membrane as a chondrocyte carrier vehicle/substrate: in vitro study. J Biomed Mater Res A. 2011;99(3):500-506.
25. Tan SL, Sulaiman S, Pingguan-Murphy B, Selvaratnam L, Tai CC, Kamarul T. Human amnion as a novel cell delivery vehicle for chondrogenic mesenchymal stem cells. Cell Tissue Bank. 2011;12(1):59-70.
26. Jin CZ, Park SR, Choi BH, Lee KY, Kang CK, Min BH. Human amniotic membrane as a delivery matrix for articular cartilage repair. Tissue Eng. 2007;13(4):693-702.
27. Garcia D, Longo UG, Vaquero J, et al. Amniotic membrane transplant for articular cartilage repair: an experimental study in sheep. Curr Stem Cell Res Ther. 2014;10(1):77-83.
28. Kunisaki SM, Freedman DA, Fauza DO. Fetal tracheal reconstruction with cartilaginous grafts engineered from mesenchymal amniocytes. J Pediatr Surg. 2006;41(4):675-682.
29. Namba RS, Meuli M, Sullivan KM, Le AX, Adzick NS. Spontaneous repair of superficial defects in articular cartilage in a fetal lamb model. J Bone Joint Surg Am. 1998;80(1):4-10.
30. Philip J, Hackl F, Canseco JA, et al. Amnion-derived multipotent progenitor cells improve achilles tendon repair in rats. Eplasty. 2013;13:e31.
31. Lange-Consiglio A, Tassan S, Corradetti B, et al. Investigating the efficacy of amnion-derived compared with bone marrow–derived mesenchymal stromal cells in equine tendon and ligament injuries. Cytotherapy. 2013;15(8):1011-1020.
32. Barboni B, Russo V, Curini V, et al. Achilles tendon regeneration can be improved by amniotic epithelial cell allotransplantation. Cell Transplant. 2012;21(11):2377-2395.
33. Kueckelhaus M, Philip J, Kamel RA, et al. Sustained release of amnion-derived cellular cytokine solution facilitates achilles tendon healing in rats. Eplasty. 2014;14:e29.
34. Beredjiklian PK, Favata M, Cartmell JS, Flanagan CL, Crombleholme TM, Soslowski LJ. Regenerative versus reparative healing in tendon: a study of biomechanical and histological properties in fetal sheep. Ann Biomed Eng. 2003;31(10):1143-1152.
35. Demirkan F, Colakoglu N, Herek O, Erkula G. The use of amniotic membrane in flexor tendon repair: an experimental model. Arch Orthop Trauma Surg. 2002;122(7):396-369.
36. Ozgenel GY. The effects of a combination of hyaluronic and amniotic membrane on the formation of peritendinous adhesions after flexor tendon surgery in chickens. J Bone Joint Surg Br. 2004;86(2):301-307.
37. Kim SS, Sohn SK, Lee KY, Lee MJ, Roh MS, Kim CH. Use of human amniotic membrane wrap in reducing perineural adhesions in a rabbit model of ulnar nerve neurorrhaphy. J Hand Surg Eur Vol. 2010;35(3):214-219.
38. Tao H, Fan H. Implantation of amniotic membrane to reduce postlaminectomy epidural adhesions. Eur Spine J. 2009;18(8):1202-1212.
39. Choi HJ, Kim KB, Kwon YM. Effect of amniotic membrane to reduce postlaminectomy epidural adhesion on a rat model. J Korean Neurosurg Soc. 2011;49(6):323-328.
40. Adzick NS, Thom EA, Spong CY, et al. A randomized trial of prenatal versus postnatal repair of myelomeningocele. N Engl J Med. 2011;364(11):993-1004.
41. Vines JB, Aliprantis AO, Gomoll AH, Farr J. Cryopreserved amniotic suspension for the treatment of knee osteoarthritis. J Knee Surg. 2016;29(6):443-450.
42. Zelen CM. An evaluation of dehydrated human amniotic membrane allografts in patients with DFUs. J Wound Care. 2013;22(7):347-348,350-351.
43. Zelen CM, Serena TE, Denoziere G, Fetterolf DE. A prospective randomised comparative parallel study of amniotic membrane wound graft in the management of diabetic foot ulcers. Int Wound J. 2013;10(5):502-507.
44. Zelen CM, Serena TE, Snyder RJ. A prospective, randomised comparative study of weekly versus biweekly application of dehydrated human amnion/chorion membrane allograft in the management of diabetic foot ulcers. Int Wound J. 2014;11(2):122-128.
45. Zelen CM, Snyder RJ, Serena TE, Li WW. The use of human amnion/chorion membrane in the clinical setting for lower extremity repair: a review. Clin Podiatr Med Surg. 2015;32(1):135-146.
46. Zelen CM, Poka A, Andrews J. Prospective, randomized, blinded, comparative study of injectable micronized dehydrated amniotic/chorionic membrane allograft for plantar fasciitis: a feasibility study. Foot Ankle Int. 2013;34(10):1332-1339.
47. Hanselman AE, Tidwell JE, Santrock RD. Cryopreserved human amniotic membrane injection for plantar fasciitis: a randomized, controlled, double-blind pilot study. Foot Ankle Int. 2015;36(2):151-158.
48. Jay RM. Initial clinical experience with the use of human amniotic membrane tissue during repair of posterior tibial and achilles tendons. 2009. http://encompassbiologics.com/wp-content/uploads/2015/07/DrJayClinicalExperience.pdf. Accessed September 29, 2016.
49. DeMill SL, Granata JD, McAlister JE, Berlet GC, Hyer CF. Safety analysis of cryopreserved amniotic membrane/umbilical cord tissue in foot and ankle surgery: a consecutive case series of 124 patients. Surg Technol Int. 2014;25:257-261.
50. Warner M, Lasyone L. An open-label, single-center, retrospective study of cryopreserved amniotic membrane and umbilical cord tissue as an adjunct for foot and ankle surgery. Surg Technol Int. 2014;25:251-255.
The amniotic membrane is a multilayer tissue forming the innermost layer of the amniotic sac that surrounds the developing fetus. It is comprised of 5 layers, from the inside out: a single layer of epithelial cells, a thick basement membrane, a compact layer, a fibroblast layer, and a spongy layer that abuts the surrounding chorion (Figure 1).1
Amniotic epithelial cells are derived from the pluripotent epiblast at approximately day 8 of gestation. This is well before gastrulation occurs at days 15 to 17, considered the “tipping point” when pluripotent cells differentiate into ectoderm, mesoderm, and endoderm.3 These cells express Oct-4 and Nanog, 2 molecular markers that are indicative of pluripotency.3 Two cell types have been identified in amniotic tissues that possess stem cell-like characteristics: human amniotic epithelial cells and human amniotic mesenchymal stromal cells.4 Both of these cell types have demonstrated the ability to differentiate into various cell lineages, including endothelial cells, adipocytes, myogenic cells, neurogenic cells, chondrocytes, tenocytes, and osteogenic cells.5-7 These previously reported findings indicate that amniotic cells and tissue have the capability to generate mesenchymal tissues.
FDA Classification and Available Forms
The US Food and Drug Administration (FDA) classifies amnion as an allograft tissue under Human Cells, Tissues, and Cellular and Tissue-Based Products (HCT/Ps) 361. To meet criteria, the tissue needs to be minimally manipulated. It is to be for homologous use and cannot be combined with other cells or tissues. There can be no systemic effect or dependence on the metabolic activity of living cells to achieve its primary function. The tissue has to have a localized effect in vivo. Therefore, amnion allograft tissue can be commercialized, provided it is not marketed as a stem cell product or to contain viable cells.
Amniotic tissue is commercially available in several forms.
Safety
Amniotic tissue has been used for over 100 years in burn, ophthalmology, and chronic wound patients with favorable outcomes and no adverse effects reported in the literature. Unlike embryonic stem cells, which may be tumorigenic,8 amniotic cells do not possess any known tumorigenicity.9 In one study, 50 immunodeficient mice were injected with 1 to 2 million amniotic epithelial cells and observed for a maximum of 516 days with no tumorigenicity observed in any of the animals.10 In another study, amniotic epithelial cells were implanted into the forearms of healthy volunteers and no immunologic response was observed in any of the recipients.11 Furthermore, viable amniotic cells were recovered via biopsy 7 weeks following transplantation, demonstrating viability of the transplanted cells.11 The lack of tumorigenicity and immunologic response in hosts is due in part to the fact that amniotic cells do not express human leukocyte antigen class II antigens and only express class I antigens in small amounts.3
Advantages of Amnion Tissue
Amniotic tissue is readily available, as it is often discarded after childbirth. The use of this tissue poses no added risk to the fetus or mother, eliminating the ethical concerns associated with obtaining embryonic stem cells. Amniotic tissue is comprised of an extracellular matrix, which acts as a natural scaffold for cellular attachment and structural support for cells as well as collagen types I, III, IV, V, and VI, hyaluronic acid, and a host of growth factors.12 In addition, it possesses antimicrobial properties, including beta-defensins.13
Amniotic tissue has been shown to exert an anti-inflammatory effect by inhibiting the inflammatory cascade. Specifically, it has been shown to inhibit cytokines such as tumor necrosis factor-alpha in the presence of dendritic cells,14 as well as inhibiting transforming growth factor-beta, interleukin-8, and fibroblast proliferation.15 These findings indicate that amniotic tissue has the ability to dampen the “cytokine storm” that occurs after an injury in an adult, which would lead to beneficial impacts on healing and scar formation in patients.16
Basic Science and Animal Studies
Several studies have demonstrated promising outcomes for orthopedic applications in vitro. A comparison of osteogenic potential found that amniotic fluid-derived cells were able to produce approximately 5 times more mineralized matrix than bone marrow-derived mesenchymal stem cells.17 More recently, Si and colleagues18 compared the osteogenic potential of human amniotic epithelial cells, amniotic cells, and human bone marrow-derived mesenchymal stem cells. They found that all 3 cell lines were osteogenic, though the amniotic epithelial cells had better immunomodulatory properties and marginally less osteogenic potential than the other 2 cell types. Furthermore, several in vivo animal studies have demonstrated the ability of human amniotic cells to stimulate bone growth in rats,19,20 rabbits,21 and sheep.22
Amniotic tissue also possesses potential for chondrogenesis. Cryopreserved human amniotic membrane cells used for in vitro human osteoarthritis tissue scaffolds did not differentiate in culture, and they integrated and repaired damaged articular cartilage.23 Various in vitro24,25 and animal in vivo26,27 studies have reported similar supportive findings. Kunisaki and colleagues28 used sheep amniotic fluid mesenchymal stem cells to reconstruct lamb tracheal cartilage in utero, concluding that cells obtained from the amniotic fluid possess chondrogenic capabilities. Further in utero lamb studies of cartilage artificial defects, given 7 days to settle before adding a hypocellular matrix as a scaffold, showed chondrocyte density and cell architecture was restored at the defect site after 28 days without the formation of an inflammatory response or scar tissue.29
Amniotic tissue has had similar success in tendon repair studies in vivo.9,30,31 Barboni and colleagues32 implanted amniotic epithelial cells (AECs) into artificially created sheep Achilles tendon defects in situ, inducing superior structural and mechanical recovery in the defects at a faster rate compared to controls not receiving AECs. Healing via AECs started at the healthy tissue around the borders of the defect and progressed centrally, suggesting recruitment of native progenitor cells to the lesion.32 Kueckelhaus and colleagues33 investigated the role of amnion-derived cellular cytokine solution in the healing of transections of rat Achilles tendons, reporting improved mechanical properties of healing tendons at early time points compared to controls. Beredjiklian and colleagues34 compared the healing of transected extensor tendons of pregnant ewes and of their fetus in utero, reporting a reparative form of healing with scar formation in adult subjects and regenerative form of healing without scar formation or inflammation in fetal subjects.
Amniotic tissue has properties that prevent adhesion formation around tendons following injury and reconstruction.35 Ozgenel36 investigated the effects of hyaluronic acid and amniotic membrane alone and in combination on the presence of adhesions and the rate of healing following chicken flexor tendon repair. The study found amniotic membrane wrapped around the repaired tendon was superior in preventing adhesion formation. Kim and colleagues37 report a similar reduction in fibrosis and adhesion following application of a human amniotic membrane wrap to rabbit ulnar neurorrhaphy sites.
This barrier function of amniotic tissue has also been investigated in the prevention of surgical scarring and peridural fibrosis in animal models following spinal discectomy. A study in canine models showed a reduction of scarring following the application of cross-linked amniotic membrane compared to freeze dried amniotic membrane.38 Similar reductions in scarring in rat models with the application of freeze-dried amniotic membrane compared to negative controls have been reported.39
Human Studies
A randomized trial investigated the outcomes of prenatal vs postnatal repair of myelomeningocele in humans, finding a reduced need for implanted shunts and improved functional outcomes at 30 months of life in the prenatal intervention group compared to the postnatal group.40 This study was concluded early due to the efficacy of prenatal surgery and the benefit of nervous system repair in utero in the presence of amniotic growth factors.
Vines and colleagues41 performed a 6-patient feasibility study using amnion injections to treat symptomatic knee osteoarthritis. Each patient received a single intra-articular cryopreserved amniotic suspension allograft (ASA) injection and was followed for 1 year. No adverse outcomes were reported, with the only abnormal finding being a small increase in serum immunoglobulin G and immunoglobulin E levels. Intra-articular ASA injection was found to be safe, but a large-scale trial investigating symptomatic relief was recommended.41
Most of the human studies using amnion pertain to foot and ankle surgery. Its use as a treatment for diabetic foot ulcers and recalcitrant plantar fasciitis was one of the early-recognized successes.42-45 Zelen and colleagues46 investigated the applications of injectable micronized dehydrated human amniotic/chorionic membrane as an alternative to surgical intervention in the treatment of refractory plantar fasciitis. This prospective, randomized trial with 45 patients showed significant improvement in plantar fasciitis symptoms at 8 weeks compared to controls (saline injections). A similar study compared the use of cryopreserved human amniotic membrane (c-hAM) injections to corticosteroid injections in plantar fasciitis patients.47 The results indicated that c-hAM is safe and comparable to corticosteroids, with the authors noting that pain improvement was greatest in patients receiving 2 injections of c-hAM at 18 weeks.
Tendon wrapping, in which the amniotic membrane is laid over a tendon repair, has been reported with success. Amniotic membrane is superior to collagen for tendon wrapping as it actively contributes to healing while minimizing adhesions, which collagen alone cannot do.48 The membrane serves as a protective sheath around repaired tendons with anti-inflammatory, anti-adhesive, immunomodulatory, and antimicrobial benefits. A 124-patient study demonstrated the safety of using amnion in this manner, and the authors reported a decreased rate of complication compared to previously published data.49 Another study of 14 patients undergoing foot and ankle surgery with tendon wrapping reported clinical improvement with reduced pain and greater functional outcomes postoperatively compared to preoperative measurements.50
Conclusion
Amniotic membrane-derived tissues are safe and non-tumorigenic, producing an abundance of growth factors that have shown promise as tissue scaffolds and as aids in the regeneration of human bone and soft tissues. Amnion applications in orthopedic surgery may be numerous, but development is ongoing. Given the vast array of in vitro and in vivo animal data supporting the benefits of amnion in tissue regeneration, orthopedic surgeons and researchers should place emphasis on conducting clinical studies to validate the safety and efficacy of amniotic cells in the treatment of orthopedic conditions.
Am J Orthop. 2016;45(7):E421-E425. Copyright Frontline Medical Communications Inc. 2016. All rights reserved.
The amniotic membrane is a multilayer tissue forming the innermost layer of the amniotic sac that surrounds the developing fetus. It is comprised of 5 layers, from the inside out: a single layer of epithelial cells, a thick basement membrane, a compact layer, a fibroblast layer, and a spongy layer that abuts the surrounding chorion (Figure 1).1
Amniotic epithelial cells are derived from the pluripotent epiblast at approximately day 8 of gestation. This is well before gastrulation occurs at days 15 to 17, considered the “tipping point” when pluripotent cells differentiate into ectoderm, mesoderm, and endoderm.3 These cells express Oct-4 and Nanog, 2 molecular markers that are indicative of pluripotency.3 Two cell types have been identified in amniotic tissues that possess stem cell-like characteristics: human amniotic epithelial cells and human amniotic mesenchymal stromal cells.4 Both of these cell types have demonstrated the ability to differentiate into various cell lineages, including endothelial cells, adipocytes, myogenic cells, neurogenic cells, chondrocytes, tenocytes, and osteogenic cells.5-7 These previously reported findings indicate that amniotic cells and tissue have the capability to generate mesenchymal tissues.
FDA Classification and Available Forms
The US Food and Drug Administration (FDA) classifies amnion as an allograft tissue under Human Cells, Tissues, and Cellular and Tissue-Based Products (HCT/Ps) 361. To meet criteria, the tissue needs to be minimally manipulated. It is to be for homologous use and cannot be combined with other cells or tissues. There can be no systemic effect or dependence on the metabolic activity of living cells to achieve its primary function. The tissue has to have a localized effect in vivo. Therefore, amnion allograft tissue can be commercialized, provided it is not marketed as a stem cell product or to contain viable cells.
Amniotic tissue is commercially available in several forms.
Safety
Amniotic tissue has been used for over 100 years in burn, ophthalmology, and chronic wound patients with favorable outcomes and no adverse effects reported in the literature. Unlike embryonic stem cells, which may be tumorigenic,8 amniotic cells do not possess any known tumorigenicity.9 In one study, 50 immunodeficient mice were injected with 1 to 2 million amniotic epithelial cells and observed for a maximum of 516 days with no tumorigenicity observed in any of the animals.10 In another study, amniotic epithelial cells were implanted into the forearms of healthy volunteers and no immunologic response was observed in any of the recipients.11 Furthermore, viable amniotic cells were recovered via biopsy 7 weeks following transplantation, demonstrating viability of the transplanted cells.11 The lack of tumorigenicity and immunologic response in hosts is due in part to the fact that amniotic cells do not express human leukocyte antigen class II antigens and only express class I antigens in small amounts.3
Advantages of Amnion Tissue
Amniotic tissue is readily available, as it is often discarded after childbirth. The use of this tissue poses no added risk to the fetus or mother, eliminating the ethical concerns associated with obtaining embryonic stem cells. Amniotic tissue is comprised of an extracellular matrix, which acts as a natural scaffold for cellular attachment and structural support for cells as well as collagen types I, III, IV, V, and VI, hyaluronic acid, and a host of growth factors.12 In addition, it possesses antimicrobial properties, including beta-defensins.13
Amniotic tissue has been shown to exert an anti-inflammatory effect by inhibiting the inflammatory cascade. Specifically, it has been shown to inhibit cytokines such as tumor necrosis factor-alpha in the presence of dendritic cells,14 as well as inhibiting transforming growth factor-beta, interleukin-8, and fibroblast proliferation.15 These findings indicate that amniotic tissue has the ability to dampen the “cytokine storm” that occurs after an injury in an adult, which would lead to beneficial impacts on healing and scar formation in patients.16
Basic Science and Animal Studies
Several studies have demonstrated promising outcomes for orthopedic applications in vitro. A comparison of osteogenic potential found that amniotic fluid-derived cells were able to produce approximately 5 times more mineralized matrix than bone marrow-derived mesenchymal stem cells.17 More recently, Si and colleagues18 compared the osteogenic potential of human amniotic epithelial cells, amniotic cells, and human bone marrow-derived mesenchymal stem cells. They found that all 3 cell lines were osteogenic, though the amniotic epithelial cells had better immunomodulatory properties and marginally less osteogenic potential than the other 2 cell types. Furthermore, several in vivo animal studies have demonstrated the ability of human amniotic cells to stimulate bone growth in rats,19,20 rabbits,21 and sheep.22
Amniotic tissue also possesses potential for chondrogenesis. Cryopreserved human amniotic membrane cells used for in vitro human osteoarthritis tissue scaffolds did not differentiate in culture, and they integrated and repaired damaged articular cartilage.23 Various in vitro24,25 and animal in vivo26,27 studies have reported similar supportive findings. Kunisaki and colleagues28 used sheep amniotic fluid mesenchymal stem cells to reconstruct lamb tracheal cartilage in utero, concluding that cells obtained from the amniotic fluid possess chondrogenic capabilities. Further in utero lamb studies of cartilage artificial defects, given 7 days to settle before adding a hypocellular matrix as a scaffold, showed chondrocyte density and cell architecture was restored at the defect site after 28 days without the formation of an inflammatory response or scar tissue.29
Amniotic tissue has had similar success in tendon repair studies in vivo.9,30,31 Barboni and colleagues32 implanted amniotic epithelial cells (AECs) into artificially created sheep Achilles tendon defects in situ, inducing superior structural and mechanical recovery in the defects at a faster rate compared to controls not receiving AECs. Healing via AECs started at the healthy tissue around the borders of the defect and progressed centrally, suggesting recruitment of native progenitor cells to the lesion.32 Kueckelhaus and colleagues33 investigated the role of amnion-derived cellular cytokine solution in the healing of transections of rat Achilles tendons, reporting improved mechanical properties of healing tendons at early time points compared to controls. Beredjiklian and colleagues34 compared the healing of transected extensor tendons of pregnant ewes and of their fetus in utero, reporting a reparative form of healing with scar formation in adult subjects and regenerative form of healing without scar formation or inflammation in fetal subjects.
Amniotic tissue has properties that prevent adhesion formation around tendons following injury and reconstruction.35 Ozgenel36 investigated the effects of hyaluronic acid and amniotic membrane alone and in combination on the presence of adhesions and the rate of healing following chicken flexor tendon repair. The study found amniotic membrane wrapped around the repaired tendon was superior in preventing adhesion formation. Kim and colleagues37 report a similar reduction in fibrosis and adhesion following application of a human amniotic membrane wrap to rabbit ulnar neurorrhaphy sites.
This barrier function of amniotic tissue has also been investigated in the prevention of surgical scarring and peridural fibrosis in animal models following spinal discectomy. A study in canine models showed a reduction of scarring following the application of cross-linked amniotic membrane compared to freeze dried amniotic membrane.38 Similar reductions in scarring in rat models with the application of freeze-dried amniotic membrane compared to negative controls have been reported.39
Human Studies
A randomized trial investigated the outcomes of prenatal vs postnatal repair of myelomeningocele in humans, finding a reduced need for implanted shunts and improved functional outcomes at 30 months of life in the prenatal intervention group compared to the postnatal group.40 This study was concluded early due to the efficacy of prenatal surgery and the benefit of nervous system repair in utero in the presence of amniotic growth factors.
Vines and colleagues41 performed a 6-patient feasibility study using amnion injections to treat symptomatic knee osteoarthritis. Each patient received a single intra-articular cryopreserved amniotic suspension allograft (ASA) injection and was followed for 1 year. No adverse outcomes were reported, with the only abnormal finding being a small increase in serum immunoglobulin G and immunoglobulin E levels. Intra-articular ASA injection was found to be safe, but a large-scale trial investigating symptomatic relief was recommended.41
Most of the human studies using amnion pertain to foot and ankle surgery. Its use as a treatment for diabetic foot ulcers and recalcitrant plantar fasciitis was one of the early-recognized successes.42-45 Zelen and colleagues46 investigated the applications of injectable micronized dehydrated human amniotic/chorionic membrane as an alternative to surgical intervention in the treatment of refractory plantar fasciitis. This prospective, randomized trial with 45 patients showed significant improvement in plantar fasciitis symptoms at 8 weeks compared to controls (saline injections). A similar study compared the use of cryopreserved human amniotic membrane (c-hAM) injections to corticosteroid injections in plantar fasciitis patients.47 The results indicated that c-hAM is safe and comparable to corticosteroids, with the authors noting that pain improvement was greatest in patients receiving 2 injections of c-hAM at 18 weeks.
Tendon wrapping, in which the amniotic membrane is laid over a tendon repair, has been reported with success. Amniotic membrane is superior to collagen for tendon wrapping as it actively contributes to healing while minimizing adhesions, which collagen alone cannot do.48 The membrane serves as a protective sheath around repaired tendons with anti-inflammatory, anti-adhesive, immunomodulatory, and antimicrobial benefits. A 124-patient study demonstrated the safety of using amnion in this manner, and the authors reported a decreased rate of complication compared to previously published data.49 Another study of 14 patients undergoing foot and ankle surgery with tendon wrapping reported clinical improvement with reduced pain and greater functional outcomes postoperatively compared to preoperative measurements.50
Conclusion
Amniotic membrane-derived tissues are safe and non-tumorigenic, producing an abundance of growth factors that have shown promise as tissue scaffolds and as aids in the regeneration of human bone and soft tissues. Amnion applications in orthopedic surgery may be numerous, but development is ongoing. Given the vast array of in vitro and in vivo animal data supporting the benefits of amnion in tissue regeneration, orthopedic surgeons and researchers should place emphasis on conducting clinical studies to validate the safety and efficacy of amniotic cells in the treatment of orthopedic conditions.
Am J Orthop. 2016;45(7):E421-E425. Copyright Frontline Medical Communications Inc. 2016. All rights reserved.
1. Benirschke K, Kaufman P. Anatomy and pathology of the placental membranes. In: Pathology of the Human Placent., 4th ed. New York, NY: Springer-Verlag; 2000:281-334.
2. Mamede AC, Carvalho MJ, Abrantes AM, Laranjo M, Maia CJ, Botelho MF. Amniotic membrane: from structure and functions to clinical applications. Cell Tissue Res. 2012;349(2):447-458.
3. Miki T, Strom SC. Amnion-derived pluripotent/multipotent stem cells. Stem Cell Rev. 2006;2(2):133-142.
4. Parolini O, Alviano F, Bagnara GP, et al. Concise review: isolation and characterization of cells from human term placenta: outcome of the first international workshop on placenta derived stem cells. Stem Cells. 2008;26(2):300-311.
5. Ilancheran S, Michalska A, Peh G, Wallace EM, Pera M, Manuelpillai U. Stem cells derived from human fetal membranes display multilineage differentiation potential. Biol Reprod. 2007;77(3):577-588.
6. Alviano F, Fossati V, Marchionni C, et al. Term amniotic membrane is a high throughput source for multipotent mesenchymal stem cells with the ability to differentiate into endothelial cells in vitro. BMC Dev Biol. 2007;7:11.
7. Barboni B, Curini V, Russo V, et al. Indirect co-culture with tendons or tenocytes can program amniotic epithelial cells towards stepwise tenogenic differentiation. PLoS One. 2012;7(2):e30974.
8. Ben-David U, Benvenisty N. The tumorigenicity of human embryonic and induced pluripotent stem cells. Nature Reviews Cancer. 2011;11(4):268-277.
9. Lange-Consiglio A, Rossi D, Tassan S, Perego R, Cremonesi F, Parolini O. Conditioned medium from horse amniotic membrane-derived multipotent progenitor cells: immunomodulatory activity in vitro and first clinical application in tendon and ligament injuries in vivo. Stem Cells Dev. 2013;22(22):3015-3024.
10. Miki T. Amnion-derived stem cells: in quest of clinical applications. Stem Cell Res Ther. 2011;2(3):25.
11. Akle CA, Adinolfi M, Welsh KI, Leibowitz S, McColl I. Immunogenicity of human amniotic epithelial cells after transplantation into volunteers. Lancet. 1981;2(8254):1003-1035.
12. Gupta A, Kedige SD, Jain K. Amnion and chorion membranes: potential stem cell reservoir with wide applications in periodontics. Int J Biomater. 2015;2015:274082.
13. Buhimschi IA, Jabr M, Buhimschi CS, Petkova AP, Weiner CP, Saed GM. The novel antimicrobial peptide beta3-defensin is produced by the amnion: a possible role of the fetal membranes in innate immunity of the amniotic cavity. Am J Obstet Gynecol. 2004;191(5):1678-1687.
14. Magatti M, De Munari S, Vertua E, et al. Amniotic mesenchymal tissue cells inhibit dendritic cell differentiation of peripheral blood and amnion resident monocytes. Cell Transplant. 2009;18(8):899-914.
15. Solomon A, Wajngarten M, Alviano F, et al. Suppression of inflammatory and fibrotic responses in allergic inflammation by the amniotic membrane stromal matrix. Clin Exp Allergy. 2005;35(7):941-948.
16. Silini A, Parolini O, Huppertz B, Lang I. Soluble factors of amnion-derived cells in treatment of inflammatory and fibrotic pathologies. Curr Stem Cell Res Ther. 2013;8(1):6-14.
17. Peister A, Woodruff MA, Prince JJ, Gray DP, Hutmacher DW, Guldberg RE. Cell sourcing for bone tissue engineering: amniotic fluid stem cells have a delayed, robust differentiation compared to mesenchymal stem cells. Stem Cell Res. 2011;7(1):17-27.
18. Si J, Dai J, Zhang J, et al. Comparative investigation of human amniotic epithelial cells and mesenchymal stem cells for application in bone tissue engineering. Stem Cells Int. 2015;2015:565732.
19. Starecki M, Schwartz JA, Grande DA. Evaluation of amniotic-derived membrane biomaterial as an adjunct for repair of critical sized bone defects. Advances in Orthopedic Surgery. 2014;2014:572586.
20. Kerimoglu S, Livaoglu M, Sönmez B, et al. Effects of human amniotic fluid on fracture healing in rat tibia. J Surg Res. 2009;152(2):281-287.
21. Karaçal N, Kocucu P, Cobanglu U, Kutlu N. Effect of human amniotic fluid on bone healing. J Surg Res. 2005;129(2):283-287.
22. Barboni B, Mangano C, Valbonetti L, et al. Synthetic bone substitute engineered with amniotic epithelial cells enhances bone regeneration after maxillary sinus augmentation. PLoS One. 2013;8(5):e63256.
23. Díaz-Prado S, Rendal-Vázquez ME, Muiños-Lopez E, et al. Potential use of the human amniotic membrane as a scaffold in human articular cartilage repair. Cell Tissue Bank. 2010;11(2):183-195.
24. Krishnamurithy G, Shilpa PN, Ahmad RE, Sulaiman S, Ng CL, Kamarul T. Human amniotic membrane as a chondrocyte carrier vehicle/substrate: in vitro study. J Biomed Mater Res A. 2011;99(3):500-506.
25. Tan SL, Sulaiman S, Pingguan-Murphy B, Selvaratnam L, Tai CC, Kamarul T. Human amnion as a novel cell delivery vehicle for chondrogenic mesenchymal stem cells. Cell Tissue Bank. 2011;12(1):59-70.
26. Jin CZ, Park SR, Choi BH, Lee KY, Kang CK, Min BH. Human amniotic membrane as a delivery matrix for articular cartilage repair. Tissue Eng. 2007;13(4):693-702.
27. Garcia D, Longo UG, Vaquero J, et al. Amniotic membrane transplant for articular cartilage repair: an experimental study in sheep. Curr Stem Cell Res Ther. 2014;10(1):77-83.
28. Kunisaki SM, Freedman DA, Fauza DO. Fetal tracheal reconstruction with cartilaginous grafts engineered from mesenchymal amniocytes. J Pediatr Surg. 2006;41(4):675-682.
29. Namba RS, Meuli M, Sullivan KM, Le AX, Adzick NS. Spontaneous repair of superficial defects in articular cartilage in a fetal lamb model. J Bone Joint Surg Am. 1998;80(1):4-10.
30. Philip J, Hackl F, Canseco JA, et al. Amnion-derived multipotent progenitor cells improve achilles tendon repair in rats. Eplasty. 2013;13:e31.
31. Lange-Consiglio A, Tassan S, Corradetti B, et al. Investigating the efficacy of amnion-derived compared with bone marrow–derived mesenchymal stromal cells in equine tendon and ligament injuries. Cytotherapy. 2013;15(8):1011-1020.
32. Barboni B, Russo V, Curini V, et al. Achilles tendon regeneration can be improved by amniotic epithelial cell allotransplantation. Cell Transplant. 2012;21(11):2377-2395.
33. Kueckelhaus M, Philip J, Kamel RA, et al. Sustained release of amnion-derived cellular cytokine solution facilitates achilles tendon healing in rats. Eplasty. 2014;14:e29.
34. Beredjiklian PK, Favata M, Cartmell JS, Flanagan CL, Crombleholme TM, Soslowski LJ. Regenerative versus reparative healing in tendon: a study of biomechanical and histological properties in fetal sheep. Ann Biomed Eng. 2003;31(10):1143-1152.
35. Demirkan F, Colakoglu N, Herek O, Erkula G. The use of amniotic membrane in flexor tendon repair: an experimental model. Arch Orthop Trauma Surg. 2002;122(7):396-369.
36. Ozgenel GY. The effects of a combination of hyaluronic and amniotic membrane on the formation of peritendinous adhesions after flexor tendon surgery in chickens. J Bone Joint Surg Br. 2004;86(2):301-307.
37. Kim SS, Sohn SK, Lee KY, Lee MJ, Roh MS, Kim CH. Use of human amniotic membrane wrap in reducing perineural adhesions in a rabbit model of ulnar nerve neurorrhaphy. J Hand Surg Eur Vol. 2010;35(3):214-219.
38. Tao H, Fan H. Implantation of amniotic membrane to reduce postlaminectomy epidural adhesions. Eur Spine J. 2009;18(8):1202-1212.
39. Choi HJ, Kim KB, Kwon YM. Effect of amniotic membrane to reduce postlaminectomy epidural adhesion on a rat model. J Korean Neurosurg Soc. 2011;49(6):323-328.
40. Adzick NS, Thom EA, Spong CY, et al. A randomized trial of prenatal versus postnatal repair of myelomeningocele. N Engl J Med. 2011;364(11):993-1004.
41. Vines JB, Aliprantis AO, Gomoll AH, Farr J. Cryopreserved amniotic suspension for the treatment of knee osteoarthritis. J Knee Surg. 2016;29(6):443-450.
42. Zelen CM. An evaluation of dehydrated human amniotic membrane allografts in patients with DFUs. J Wound Care. 2013;22(7):347-348,350-351.
43. Zelen CM, Serena TE, Denoziere G, Fetterolf DE. A prospective randomised comparative parallel study of amniotic membrane wound graft in the management of diabetic foot ulcers. Int Wound J. 2013;10(5):502-507.
44. Zelen CM, Serena TE, Snyder RJ. A prospective, randomised comparative study of weekly versus biweekly application of dehydrated human amnion/chorion membrane allograft in the management of diabetic foot ulcers. Int Wound J. 2014;11(2):122-128.
45. Zelen CM, Snyder RJ, Serena TE, Li WW. The use of human amnion/chorion membrane in the clinical setting for lower extremity repair: a review. Clin Podiatr Med Surg. 2015;32(1):135-146.
46. Zelen CM, Poka A, Andrews J. Prospective, randomized, blinded, comparative study of injectable micronized dehydrated amniotic/chorionic membrane allograft for plantar fasciitis: a feasibility study. Foot Ankle Int. 2013;34(10):1332-1339.
47. Hanselman AE, Tidwell JE, Santrock RD. Cryopreserved human amniotic membrane injection for plantar fasciitis: a randomized, controlled, double-blind pilot study. Foot Ankle Int. 2015;36(2):151-158.
48. Jay RM. Initial clinical experience with the use of human amniotic membrane tissue during repair of posterior tibial and achilles tendons. 2009. http://encompassbiologics.com/wp-content/uploads/2015/07/DrJayClinicalExperience.pdf. Accessed September 29, 2016.
49. DeMill SL, Granata JD, McAlister JE, Berlet GC, Hyer CF. Safety analysis of cryopreserved amniotic membrane/umbilical cord tissue in foot and ankle surgery: a consecutive case series of 124 patients. Surg Technol Int. 2014;25:257-261.
50. Warner M, Lasyone L. An open-label, single-center, retrospective study of cryopreserved amniotic membrane and umbilical cord tissue as an adjunct for foot and ankle surgery. Surg Technol Int. 2014;25:251-255.
1. Benirschke K, Kaufman P. Anatomy and pathology of the placental membranes. In: Pathology of the Human Placent., 4th ed. New York, NY: Springer-Verlag; 2000:281-334.
2. Mamede AC, Carvalho MJ, Abrantes AM, Laranjo M, Maia CJ, Botelho MF. Amniotic membrane: from structure and functions to clinical applications. Cell Tissue Res. 2012;349(2):447-458.
3. Miki T, Strom SC. Amnion-derived pluripotent/multipotent stem cells. Stem Cell Rev. 2006;2(2):133-142.
4. Parolini O, Alviano F, Bagnara GP, et al. Concise review: isolation and characterization of cells from human term placenta: outcome of the first international workshop on placenta derived stem cells. Stem Cells. 2008;26(2):300-311.
5. Ilancheran S, Michalska A, Peh G, Wallace EM, Pera M, Manuelpillai U. Stem cells derived from human fetal membranes display multilineage differentiation potential. Biol Reprod. 2007;77(3):577-588.
6. Alviano F, Fossati V, Marchionni C, et al. Term amniotic membrane is a high throughput source for multipotent mesenchymal stem cells with the ability to differentiate into endothelial cells in vitro. BMC Dev Biol. 2007;7:11.
7. Barboni B, Curini V, Russo V, et al. Indirect co-culture with tendons or tenocytes can program amniotic epithelial cells towards stepwise tenogenic differentiation. PLoS One. 2012;7(2):e30974.
8. Ben-David U, Benvenisty N. The tumorigenicity of human embryonic and induced pluripotent stem cells. Nature Reviews Cancer. 2011;11(4):268-277.
9. Lange-Consiglio A, Rossi D, Tassan S, Perego R, Cremonesi F, Parolini O. Conditioned medium from horse amniotic membrane-derived multipotent progenitor cells: immunomodulatory activity in vitro and first clinical application in tendon and ligament injuries in vivo. Stem Cells Dev. 2013;22(22):3015-3024.
10. Miki T. Amnion-derived stem cells: in quest of clinical applications. Stem Cell Res Ther. 2011;2(3):25.
11. Akle CA, Adinolfi M, Welsh KI, Leibowitz S, McColl I. Immunogenicity of human amniotic epithelial cells after transplantation into volunteers. Lancet. 1981;2(8254):1003-1035.
12. Gupta A, Kedige SD, Jain K. Amnion and chorion membranes: potential stem cell reservoir with wide applications in periodontics. Int J Biomater. 2015;2015:274082.
13. Buhimschi IA, Jabr M, Buhimschi CS, Petkova AP, Weiner CP, Saed GM. The novel antimicrobial peptide beta3-defensin is produced by the amnion: a possible role of the fetal membranes in innate immunity of the amniotic cavity. Am J Obstet Gynecol. 2004;191(5):1678-1687.
14. Magatti M, De Munari S, Vertua E, et al. Amniotic mesenchymal tissue cells inhibit dendritic cell differentiation of peripheral blood and amnion resident monocytes. Cell Transplant. 2009;18(8):899-914.
15. Solomon A, Wajngarten M, Alviano F, et al. Suppression of inflammatory and fibrotic responses in allergic inflammation by the amniotic membrane stromal matrix. Clin Exp Allergy. 2005;35(7):941-948.
16. Silini A, Parolini O, Huppertz B, Lang I. Soluble factors of amnion-derived cells in treatment of inflammatory and fibrotic pathologies. Curr Stem Cell Res Ther. 2013;8(1):6-14.
17. Peister A, Woodruff MA, Prince JJ, Gray DP, Hutmacher DW, Guldberg RE. Cell sourcing for bone tissue engineering: amniotic fluid stem cells have a delayed, robust differentiation compared to mesenchymal stem cells. Stem Cell Res. 2011;7(1):17-27.
18. Si J, Dai J, Zhang J, et al. Comparative investigation of human amniotic epithelial cells and mesenchymal stem cells for application in bone tissue engineering. Stem Cells Int. 2015;2015:565732.
19. Starecki M, Schwartz JA, Grande DA. Evaluation of amniotic-derived membrane biomaterial as an adjunct for repair of critical sized bone defects. Advances in Orthopedic Surgery. 2014;2014:572586.
20. Kerimoglu S, Livaoglu M, Sönmez B, et al. Effects of human amniotic fluid on fracture healing in rat tibia. J Surg Res. 2009;152(2):281-287.
21. Karaçal N, Kocucu P, Cobanglu U, Kutlu N. Effect of human amniotic fluid on bone healing. J Surg Res. 2005;129(2):283-287.
22. Barboni B, Mangano C, Valbonetti L, et al. Synthetic bone substitute engineered with amniotic epithelial cells enhances bone regeneration after maxillary sinus augmentation. PLoS One. 2013;8(5):e63256.
23. Díaz-Prado S, Rendal-Vázquez ME, Muiños-Lopez E, et al. Potential use of the human amniotic membrane as a scaffold in human articular cartilage repair. Cell Tissue Bank. 2010;11(2):183-195.
24. Krishnamurithy G, Shilpa PN, Ahmad RE, Sulaiman S, Ng CL, Kamarul T. Human amniotic membrane as a chondrocyte carrier vehicle/substrate: in vitro study. J Biomed Mater Res A. 2011;99(3):500-506.
25. Tan SL, Sulaiman S, Pingguan-Murphy B, Selvaratnam L, Tai CC, Kamarul T. Human amnion as a novel cell delivery vehicle for chondrogenic mesenchymal stem cells. Cell Tissue Bank. 2011;12(1):59-70.
26. Jin CZ, Park SR, Choi BH, Lee KY, Kang CK, Min BH. Human amniotic membrane as a delivery matrix for articular cartilage repair. Tissue Eng. 2007;13(4):693-702.
27. Garcia D, Longo UG, Vaquero J, et al. Amniotic membrane transplant for articular cartilage repair: an experimental study in sheep. Curr Stem Cell Res Ther. 2014;10(1):77-83.
28. Kunisaki SM, Freedman DA, Fauza DO. Fetal tracheal reconstruction with cartilaginous grafts engineered from mesenchymal amniocytes. J Pediatr Surg. 2006;41(4):675-682.
29. Namba RS, Meuli M, Sullivan KM, Le AX, Adzick NS. Spontaneous repair of superficial defects in articular cartilage in a fetal lamb model. J Bone Joint Surg Am. 1998;80(1):4-10.
30. Philip J, Hackl F, Canseco JA, et al. Amnion-derived multipotent progenitor cells improve achilles tendon repair in rats. Eplasty. 2013;13:e31.
31. Lange-Consiglio A, Tassan S, Corradetti B, et al. Investigating the efficacy of amnion-derived compared with bone marrow–derived mesenchymal stromal cells in equine tendon and ligament injuries. Cytotherapy. 2013;15(8):1011-1020.
32. Barboni B, Russo V, Curini V, et al. Achilles tendon regeneration can be improved by amniotic epithelial cell allotransplantation. Cell Transplant. 2012;21(11):2377-2395.
33. Kueckelhaus M, Philip J, Kamel RA, et al. Sustained release of amnion-derived cellular cytokine solution facilitates achilles tendon healing in rats. Eplasty. 2014;14:e29.
34. Beredjiklian PK, Favata M, Cartmell JS, Flanagan CL, Crombleholme TM, Soslowski LJ. Regenerative versus reparative healing in tendon: a study of biomechanical and histological properties in fetal sheep. Ann Biomed Eng. 2003;31(10):1143-1152.
35. Demirkan F, Colakoglu N, Herek O, Erkula G. The use of amniotic membrane in flexor tendon repair: an experimental model. Arch Orthop Trauma Surg. 2002;122(7):396-369.
36. Ozgenel GY. The effects of a combination of hyaluronic and amniotic membrane on the formation of peritendinous adhesions after flexor tendon surgery in chickens. J Bone Joint Surg Br. 2004;86(2):301-307.
37. Kim SS, Sohn SK, Lee KY, Lee MJ, Roh MS, Kim CH. Use of human amniotic membrane wrap in reducing perineural adhesions in a rabbit model of ulnar nerve neurorrhaphy. J Hand Surg Eur Vol. 2010;35(3):214-219.
38. Tao H, Fan H. Implantation of amniotic membrane to reduce postlaminectomy epidural adhesions. Eur Spine J. 2009;18(8):1202-1212.
39. Choi HJ, Kim KB, Kwon YM. Effect of amniotic membrane to reduce postlaminectomy epidural adhesion on a rat model. J Korean Neurosurg Soc. 2011;49(6):323-328.
40. Adzick NS, Thom EA, Spong CY, et al. A randomized trial of prenatal versus postnatal repair of myelomeningocele. N Engl J Med. 2011;364(11):993-1004.
41. Vines JB, Aliprantis AO, Gomoll AH, Farr J. Cryopreserved amniotic suspension for the treatment of knee osteoarthritis. J Knee Surg. 2016;29(6):443-450.
42. Zelen CM. An evaluation of dehydrated human amniotic membrane allografts in patients with DFUs. J Wound Care. 2013;22(7):347-348,350-351.
43. Zelen CM, Serena TE, Denoziere G, Fetterolf DE. A prospective randomised comparative parallel study of amniotic membrane wound graft in the management of diabetic foot ulcers. Int Wound J. 2013;10(5):502-507.
44. Zelen CM, Serena TE, Snyder RJ. A prospective, randomised comparative study of weekly versus biweekly application of dehydrated human amnion/chorion membrane allograft in the management of diabetic foot ulcers. Int Wound J. 2014;11(2):122-128.
45. Zelen CM, Snyder RJ, Serena TE, Li WW. The use of human amnion/chorion membrane in the clinical setting for lower extremity repair: a review. Clin Podiatr Med Surg. 2015;32(1):135-146.
46. Zelen CM, Poka A, Andrews J. Prospective, randomized, blinded, comparative study of injectable micronized dehydrated amniotic/chorionic membrane allograft for plantar fasciitis: a feasibility study. Foot Ankle Int. 2013;34(10):1332-1339.
47. Hanselman AE, Tidwell JE, Santrock RD. Cryopreserved human amniotic membrane injection for plantar fasciitis: a randomized, controlled, double-blind pilot study. Foot Ankle Int. 2015;36(2):151-158.
48. Jay RM. Initial clinical experience with the use of human amniotic membrane tissue during repair of posterior tibial and achilles tendons. 2009. http://encompassbiologics.com/wp-content/uploads/2015/07/DrJayClinicalExperience.pdf. Accessed September 29, 2016.
49. DeMill SL, Granata JD, McAlister JE, Berlet GC, Hyer CF. Safety analysis of cryopreserved amniotic membrane/umbilical cord tissue in foot and ankle surgery: a consecutive case series of 124 patients. Surg Technol Int. 2014;25:257-261.
50. Warner M, Lasyone L. An open-label, single-center, retrospective study of cryopreserved amniotic membrane and umbilical cord tissue as an adjunct for foot and ankle surgery. Surg Technol Int. 2014;25:251-255.
Allografts for Ligament Reconstruction: Where Are We Now?
Musculoskeletal allografts are becoming increasingly accepted as a viable alternative to autografts in a variety of orthopedic procedures. A 2006 American Orthopaedic Society for Sports Medicine (AOSSM) survey indicated that 86% of the participating 365 orthopedic surgeons use allografts in their practice.1 Although the overwhelming majority of orthopedic surgeons use allografts, they share common concerns, including safety, tissue integrity, and biologic incorporation. It is essential for the orthopedic surgeon to understand the current standards of tissue banking, risks and benefits related to the use of allografts, and common indications for safe use in clinical practice. This article reviews the current status of musculoskeletal allografts, including tissue procurement and processing, infections, complications, and specific uses tailored to ligament reconstruction.
Donor Bank, Processing, Sterilization, and Regulation
In the United States, the American Association of Tissue Banks (AATB) is responsible for establishing the standards for more than 100 accredited tissue banks. These tissue banks recover tissue from approximately 30,000 donors annually and account for an estimated 90% of the available musculoskeletal allografts used in the United States. While not all tissue banks are accredited by the AATB, all are required to register with the Food and Drug Administration (FDA), which allows for unannounced inspections of any facility. Facilities are required to abide by the FDA-implemented Current Good Tissue Practices (CGTP), which encompasses regulations on all donor tissue collected after May 2005 to help prevent the transmission of communicable diseases. The FDA released an updated draft in January 2009 that emphasizes safe practices and regulations spanning from environmental control to specific equipment.2
The safety of a transplanted allograft tissue begins within the tissue bank. Donor screening and testing is the first step in reducing the risk of transmission. Screening consists of collecting medical and social history from the family and any healthcare resources to assess the eligibility of the donor. If prior blood donations or autopsy information is available, that information is scrutinized. Donor tissue undergoes nucleic acid testing (NAT), which is required by both the AATB and FDA. All donor tissue must be screened for both types of human immunodeficiency virus (HIV), hepatitis B virus (HBV), hepatitis C virus (HCV), treponema pallidum, and human transmissible spongiform encephalopathies.3 NAT of donor tissue effectively reduces the risk of viral transmission. Additionally, routine preprocessing swabs for bacterial and fungal cultures are performed, although the sensitivity of these cultures ranges from 78% to 92%.4
After donor screening and testing, allograft tissues are usually obtained under aseptic conditions, though this is not FDA-required.5 Once procured, the tissue undergoes sterilization. Currently, there is no standard method ubiquitous to all tissue banks, nor does the FDA require a specific method. Rather, the FDA and AATB require tissue banks to validate their sterilization process and provide supporting data. The goal of sterilization is to inactivate viruses and eradicate bacteria while maintaining the biological and mechanical properties of the tissue. The AATB requires a Sterility Assurance Level (SAL) of 10-6, meaning there is no more than one in a million chance that a nonviral viable microbe exists on or within the tissue. Sterilization techniques may include both radiation and a variety of chemical reagents. Gamma irradiation is a commonly used method of sterilizing soft tissue allografts, although some studies indicate that it is detrimental to tissue biology.6 Newer methods of sterilization are being tested, one of which includes carbon dioxide in combination with antioxidants and irradiation. Bui and colleagues7 directly compared the biomechanical and histological properties of allograft tissue after either the standard 25 kGy gamma irradiation or supercritical carbon dioxide techniques. Although there is no histological difference, the samples treated with supercritical carbon dioxide had less biomechanical damage.7 Finally, the terminally sterilized allograft tissue is frozen to temperatures between -40°C and -80°C.5
Infections
One major concern of allografts is the risk of disease transmission. While numerous studies have investigated the incidence of bacterial infection following transplantation of allograft tissue, there are challenges associated with differentiating common postoperative infections from ones directly associated with the transmission of bacteria within the graft. There is a wide array of reported incidences of infection in the literature, from the Tomford and colleagues8 1981 study that reported a 6.9% rateto the 2001 study by Munting and colleagues,9 who reported 0% in their series. Multiple confounding variables exist, such as possible contamination during handling of an otherwise noncontaminated or properly sterilized allograft with inappropriate inclusion of all postoperative infections. In contrast, recognizing viral transmission has been somewhat easier, although reporting of these incidences has been variable in the past. In either case, there is no accredited reporting system for infections related to allografts.
Bacterial Transmission
Clostridium species. Clostridium species are commonly found among intestinal flora. There is a general consensus that between 24 to 48 hours after death intestinal flora transmigrates into the surrounding tissue and blood. Therefore, a commonly accepted recommendation is that cadaveric tissue needs to be excised prior to 24 hours postmortem.10
In 2001, a 23-year-old man underwent reconstructive knee surgery with a femoral condyle allograft. A few days after surgery, he became septic and ultimately died from the infection. Clostridium sordellii was cultured from the tissue. Several days later, a 17-year-old boy underwent reconstructive knee surgery with a fresh femoral condyle and frozen meniscus from the same donor. Twenty-four hours after surgery, he developed a fever and was readmitted a week later for presumed infection and treated effectively with penicillin and ampicillin/sulbactam. Tissue from the same cadaveric donor had been transplanted into 7 other patients without reports of infection. In a 2002 Centers for Disease Control and Prevention (CDC) update report,11 there were 26 total bacterial cases from allografts and 13 cases were attributed to Clostridium. Malinin and colleagues10 reviewed 795 consecutive cadaveric donors and found that 64 (8.1%) had positive cultures for Clostridia. Of all the positive cultures for Clostridia, 81.3% had positive blood cultures, 57.8% had positive bone marrow aspirate cultures, and 46.9% had positive tissue cultures. They concluded that multiple cultures are required for cadaveric tissue donors in order to reach a higher sensitivity for Clostridial contamination, and these should be done routinely to guide the sterilization process.
Strep species. In 2003, a 17-year-old boy underwent anterior cruciate ligament (ACL) reconstruction with a patellar tendon allograft.12 About 1 week later, he was admitted for signs of infection and received intravenous antibiotics. He required surgical debridement, and intraoperative cultures grew Group A Streptococcus (GAS) that was also identified in the postmortem donor cultures. The tissues underwent processing in an antimicrobial solution and postprocessing cultures were negative for bacteria, but they were not sterilized. Tissues from this donor had been implanted in 5 other patients without report of infection. Following this event, recommendations have been made for prompt rejection of tissue with cultures positive for GAS, unless a sterilizing procedure is used.
Other bacteria. According to the 2002 CDC update, 11 of the 26 cases of bacterial infection reported to the agency were a combination of gram-negative bacilli, polymicrobial flora, or culture negative.11
Viral Transmission
The most effective way to prevent transmission of a viral disease from allografts is thorough donor screening. Since the AATB implemented NAT in 2005 for HIV and HCV, there have been no reported cases of transmission.3 Even prior to this, regular blood screening along with social questionnaires completed by donors or donor families eliminated high-risk donors and significantly decreased the rate of transmission.
Human Immunodeficiency Virus. The first reported case of HIV transmission via implantation of allograft was in 1988. Further investigation revealed that there were 8 transmissions between 1984 and 1986, when routine screening of donors had not yet been implemented. The last reported case of HIV transmission occurred in 1996 with an untested donor.13Hepatitis C Virus. There are several reported cases of HCV transmission that occurred where the donors initially tested negative for HCV. In one case, 40 allografts from the same donor were transplanted over a period of nearly 2 years. This resulted in at least 8 patients being infected with HCV.14 Another case of HCV transmission was reported in 2005 after a patient developed acute HCV 6 weeks after transplantation of a patellar tendon allograft. Further investigation revealed that there had been 3 additional cases over a year from the same donor. Researchers determined that if the initial case had been reported, at least 3 transmissions could have been prevented.15Human T-cell Lymphotropic Virus (HTLV).The first reported transmission of HTLV was in 1991. This was reported in an asymptomatic patient who received a femoral head allograft from a donor who had been previously infected via a blood transfusion.16Zika virus. With recent outbreaks of the Zika virus, the FDA recently released recommendations regarding the screening and deferral of donors, mainly for blood transfusion. Orthopedists should take into consideration the potential for transmission through allografts. The FDA states that all potential donors should be screened for Zika virus using questionnaires and whole blood tests. Symptomatic donors are deferred at least 4 weeks following resolution of symptoms. While this is a recent recommendation from the FDA, orthopedists must be cognizant of the potential harms from this unfamiliar and evolving situation.17
Graft Specifics
Anterior Cruciate Ligament
ACL reconstruction is one of the most commonly performed surgeries by orthopedic surgeons, with an estimated 200,000 reconstructions per year.18Despite the popularity of this surgery, controversies remain regarding the optimal graft for reconstruction.19,20 One would provide adequate strength, be readily available, not elicit an immunologic response from the host, rapidly incorporate, elicit low morbidity, and vascularize early. Current options include both autografts and allografts. Common autograft options include patellar bone-tendon-bone (PBTB), hamstrings tendon, quadriceps tendon, and iliotibial band. PBTB autograft remains a common choice among orthopedic surgeons, as it allows early incorporation of the graft into bone and eliminates immune rejection. However, donor site morbidity, including anterior knee pain, weakness of knee extension, joint stiffness, increased postoperative pain, and iatrogenic patella fractures, have been reported in the literature.21 Commonly used allograft options include donor bone-patellar tendon-bone, quadriceps tendon, Achilles tendon, anterior and posterior tibialis tendons, hamstring tendons, and iliotibial band. Allografts provide the advantage of avoiding donor site morbidity, being readily available, allowing for shorter operative times, and providing lower postoperative pain compared to autografts, although they carry the risk of disease transmission, rejection, and slower incorporation into bone.22-27
Autograft donor site morbidities. One of the general disadvantages of autografts is the donor site morbidity associated with harvesting the grafts. In specific, PBTB grafts allow for bony blocks on both ends of the graft to incorporate into the host bone. However, this technique comes with the risk of disrupting the extensor mechanism.28,29 Milankov and colleagues30 published a retrospective review of over 2000 ACLs using autologous PBTB graft. They noted a 0.45% incidence of patella fracture and 0.18% patellar tendon rupture.30 Others have reported that intraoperative repair of the patellar tendon after tendon harvesting can increase infrapatellar fibrosis, thus increasing the risk for stiffness.31-33
Hamstring autografts include the semitendinosus and the gracilis tendons. The harvesting process is technically demanding and can be complicated by inadvertent amputation of the tendons, making the graft unsuitable for reconstructive purposes.34 Additionally, several reports have identified persistent numbness and hyperesthesia following hamstring harvesting due to iatrogenic injury to the prepatellar branches of the saphenous nerve.35,36A comprehensive review by Slone and colleagues37 reported comparable functional outcomes with quadriceps tendon autograft compared to PBTB; however, this comes with the risk of postoperative hematoma formation and the potential for thigh compartment syndrome.
Biology and Biomechanics of Allografts
One of the major disadvantages of allografts is the reduced ability to incorporate into the host tissue. Several in vitro and animal studies have suggested that allografts incorporate in the host slower than autografts.24,26,38 Early studies by Jackson and colleagues24 on goat models demonstrated that allografts and autografts have similar structural and biological properties initially, but allografts display significantly slower incorporation into the host tissue at 6 months. Histologically, allografts demonstrated lower revascularization, a smaller cross-sectional area, and a prolonged inflammatory response at 6 months postoperatively.24,39,40 Muramatsu and colleagues41 further showed through the use of magnetic resonance imaging a slower rate of revascularization of allografts over 2 years post-reconstruction.
Acknowledging these limitations, one should use caution when choosing to use an allograft or starting aggressive early rehabilitation after an allograft reconstruction, especially in athletes and young patients.
Clinical Outcomes
Although in vitro studies demonstrate inferior strength and delayed incorporation of allografts in the early postoperative period, there is still controversy surrounding the clinical and functional outcomes. Numerous studies have identified allografts as a viable option for ACL reconstruction, with similar reported patient satisfaction scores compared to autografts.43,44
The MOON Consortium recently published a prospective study of nearly 2500 subjects looking to identify risk factors for failure of ACL reconstruction. The study found that allografts had an odds ratio for failure 5.2 times that of PBTB autografts, correlating this factor to an increased re-tear rate of 6.9% in the allograft group compared to 3.2% in the PBTB group (P < .01).45 The elevated risk is more prevalent in younger patients, especially athletic teenagers. This issue has been reiterated in multiple studies.45-50A meta-analysis by Hu and colleagues23 identified 9 studies, either randomized control trials or prospective cohort studies, that looked at clinical outcomes between the different graft choices. They showed there was no significant difference between graft options in terms of instrumental laxity (P = .59), Lachman test (P = .41), pivot shift test (P = .88), and multiple functional outcome scores, including the International Knee Documentation Committee (IKDC), Lysholm, and Tegner scores.23,51-59Processing and sterilization techniques are thought to play a role in allograft failure. Guo and other researchers have demonstrated a significantly higher rate of failure for patients who received gamma-irradiated allografts compared to fresh frozen allografts.23,58-64 With improved sterilization techniques and a strict selection process of donors, gamma radiation has fallen out of favor to protect the biological characteristics of the tissue graft.5,65,66Several factors need to be considered when selecting between allograft or autograft tissue for ligamentous reconstruction. The selection must be balanced between the surgeon’s experience, patient and surgeon preferences, age of the patient, level of physical activity, primary or revision surgical setting, multiligamentous failure, geographical availability of donor grafts, and economical factors.
Medial Patellofemoral Ligament Reconstruction
Another relatively recent application for allografts has been described for the reconstruction of the medial patellofemoral ligament (MPFL) in recurrent lateral patellar dislocations.67-74
Typically, MPFL reconstructions make use of autografts, including quadriceps tendon, patellar tendon, and hamstring ligaments. However, allografts have the potential to limit postoperative donor site morbidity and to allow a faster rehabilitation.75,76 Allografts include semitendinosus, gracilis, anterior tibialis, posterior tibialis, and quadriceps tendons.
Calvo Rodríguez and colleagues76 performed a retrospective review in 2015 comparing allografts to autografts for MPFL reconstruction with respect to postoperative knee function and re-dislocation rates. Among the collective 28 patients, there was no difference in overall functional scores or dislocation rates between the grafts. Although this was a retrospective review and had a small number of subjects, the findings identify allografts as a reliable graft option for MPFL reconstruction.76While there has been a surge of interest in techniques for MPFL reconstruction, there is limited research available regarding the superiority of allografts compared to autografts. For this specific application, it seems that clinical outcomes correlate more to adequate stabilization of the patellofemoral joint than to the type of graft used.77,78 Future research should be dedicated to prospective randomized control trials to delineate any disadvantages to using allografts for MPFL reconstruction.
Discussion
Musculoskeletal allografts are gaining popularity for ligamentous reconstruction as their safety and efficacy continue to improve. With the great majority of tissue banks being accredited by the AATB and specific regulations such as NAT screening becoming common practice, infection rates and transmission of diseases have become incredibly rare. However, a thorough consideration needs to be taken into account when choosing between autograft and allograft on a case-by-case basis (Table).
Am J Orthop. 2016;45(7):446-453. Copyright Frontline Medical Communications Inc. 2016. All rights reserved.
1. The American Orthopaedic Society for Sports Medicine. Allografts for ACL Reconstruction Survey Report. 2013. http://www.sportsmed.org/AOSSMIMIS/members/downloads/research/AllograftACLReconstructionSurveyReport.pdf. Accessed October 21, 2016.
2. US Department of Health and Human Services, Food and Drug Administration. Guidance for industry: Current good tissue practice (CGTP) and additional requirements for manufacturers of human cells, tissues, and cellular and tissue-based products (HCT/Ps). http://www.fda.gov/downloads/BiologicsBloodVaccines/GuidanceComplianceRegulatoryInformation/Guidances/Tissue/UCM285223.pdf. Published December 2011. Accessed August 17, 2015.
3. Vaishnav S, Thomas Vangsness C Jr, Dellamaggiora R. New techniques in allograft tissue processing. Clin Sports Med. 2009;28(1):127-141.
4. Veen MR, Bloem RM, Petit PL. Sensitivity and negative predictive value of swab cultures in musculoskeletal allograft procurement. Clin Orthop Relat Res. 1994;(300):259-263.
5. McAllister DR, Joyce MJ, Mann BJ, Vangsness CT Jr. Allograft update: the current status of tissue regulation, procurement, processing, and sterilization. Am J Sports Med. 2007;35(12):2148-2158.
6. Mickiewicz P, Binkowski M, Bursig H, Wróbel Z. Preservation and sterilization methods of the meniscal allografts: literature review. Cell Tissue Bank. 2014;15(3):307-317.
7. Bui D, Lovric V, Oliver R, Bertollo N, Broe D, Walsh WR. Meniscal allograft sterilisation: effect on biomechanical and histological properties. Cell Tissue Bank. 2015;16(3):467-475.
8. Tomford WW, Starkweather RJ, Goldman MH. A study of the clinical incidence of infection in the use of banked allograft bone. J Bone Joint Surg Am. 1981;63(2):244-248.
9. Munting E, Faundez A, Manche E. Vertebral reconstruction with cortical allograft: long-term evaluation. Eur Spine J. 2001;10 Suppl 2:S153-S157.
10. Malinin TI, Buck BE, Temple HT, Martinez OV, Fox WP. Incidence of clostridial contamination in donors’ musculoskeletal tissue. J Bone Joint Surg Br. 2003;85(7):1051-1054.
11. Centers for Disease Control and Prevention (CDC). Update: allograft-associated bacterial infections--United States, 2002. MMWR Morb Mortal Wkly Rep. 2002;51(10):207-210.
12. Centers for Disease Control and Prevention (CDC). Invasive Streptococcus pyogenes after allograft implantation--Colorado, 2003. MMWR Morb Mortal Wkly Rep. 2003;52(48):1174-1176.
13. Hinsenkamp M, Muylle L, Eastlund T, Fehily D, Noël L, Strong DM. Adverse reactions and events related to musculoskeletal allografts: reviewed by the World Health Organisation project NOTIFY. Int Orthop. 2012;36(3):633-641.
14. Schratt HE, Regel G, Kiesewetter B, Tscherne H. HIV infection caused by cold preserved bone transplants. Unfallchirurg. 1996;99(9):679-684.
15. Tugwell BD, Patel PR, Williams IT, et al. Transmission of hepatitis C virus to several organ and tissue recipients from an antibody-negative donor. Ann Intern Med. 2005;143(9):648-654.
16. Sanzén L, Carlsson A. Transmission of human T-cell lymphotrophic virus type 1 by a deep-frozen bone allograft. Acta Orthop Scand. 1997;68(1):72-74.
17. US Department of Health and Human Services, Food and Drug Administration. Recommendations for donor screening, deferral, and product management to reduce the risk of transfusion-transmission of Zika virus. Guidance for industry. http://www.fda.gov/downloads/BiologicsBloodVaccines/GuidanceComplianceRegulatoryInformation/Guidances/Blood/UCM486360.pdf. Published February 2016. Accessed August 10, 2016.
18. Gottlob CA, Baker CL Jr, Pellissier JM, Colvin L. Cost effectiveness of anterior cruciate ligament reconstruction in young adults. Clin Orthop Relat Res. 1999;(367):272-282.
19. Fu F, Christel P, Miller MD, Johnson DL. Graft selection for anterior cruciate ligament reconstruction. Instr Course Lect. 2009;58:337-354.
20. Chechik O, Amar E, Khashan M, Lador R, Eyal G, Gold A. An international survey on anterior cruciate ligament reconstruction practices. Int Orthop. 2013;37(2):201-206.
21. Spindler KP, Kuhn JE, Freedman KB, Matthews CE, Dittus RS, Harrell FE Jr. Anterior cruciate ligament reconstruction autograft choice: bone-tendon-bone versus hamstring: does it really matter? A systematic review. Am J Sports Med. 2004;32(8):1986-1995.
22. Harner CD, Irrgang JJ, Paul J, Dearwater S, Fu FH. Loss of motion after anterior cruciate ligament reconstruction. Am J Sports Med. 1992;20(5):499-506.
23. Hu J, Qu J, Xu D, Zhou J, Lu H. Allograft versus autograft for anterior cruciate ligament reconstruction: an up-to-date meta-analysis of prospective studies. Int Orthop. 2013;37(2):311-320.
24. Jackson DW, Grood ES, Goldstein JD, et al. A comparison of patellar tendon autograft and allograft used for anterior cruciate ligament reconstruction in the goat model. Am J Sports Med. 1993;21(2):176-185.
25. Mroz TE, Joyce MJ, Steinmetz MP, Lieberman IH, Wang JC. Musculoskeletal allograft risks and recalls in the United States. J Am Acad Orthop Surg. 2008;16(10):559-565.
26. Malinin TI, Levitt RL, Bashore C, Temple HT, Mnaymneh W. A study of retrieved allografts used to replace anterior cruciate ligaments. Arthroscopy. 2002;18(2):163-170.
27. Foster TE, Wolfe BL, Ryan S, Silvestri L, Kaye EK. Does the graft source really matter in the outcome of patients undergoing anterior cruciate ligament reconstruction? An evaluation of autograft versus allograft reconstruction results: a systematic review. Am J Sports Med. 2010;38(1):189-199.
28. Lee GH, McCulloch P, Cole BJ, Bush-Joseph CA, Bach BR Jr. The incidence of acute patellar tendon harvest complications for anterior cruciate ligament reconstruction. Arthroscopy. 2008;24(2):162-166.
29. Marumoto JM, Mitsunaga MM, Richardson AB, Medoff RJ, Mayfield GW. Late patellar tendon ruptures after removal of the central third for anterior cruciate ligament reconstruction. A report of two cases. Am J Sports Med. 1996;24(5):698-701.
30 Milankov M, Kecojević V, Rasović P, Kovacević N, Gvozdenović N, Obradović M. Disruption of the knee extensor apparatus complicating anterior cruciate ligament reconstruction. Acta Chir Iugosl. 2013;60(2):13-21.
31. Atkinson TS, Atkinson PJ, Mendenhall HV, Haut RC. Patellar tendon and infrapatellar fat pad healing after harvest of an ACL graft. J Surg Res. 1998;79(1):25-30.
32. Tang G, Niitsu M, Ikeda K, Endo H, Itai Y. Fibrous scar in the infrapatellar fat pad after arthroscopy: MR imaging. Radiat Med. 2000;18(1):1-5.
33. Unterhauser FN, Bosch U, Zeichen J, Weiler A. Alpha-smooth muscle actin containing contractile fibroblastic cells in human knee arthrofibrosis tissue. Winner of the AGA-DonJoy Award 2003. Arch Orthop Trauma Surg. 2004;124(9):585-591.
34. Prodromos CC, Fu FH, Howell SM, Johnson DH, Lawhorn K. Controversies in soft-tissue anterior cruciate ligament reconstruction: grafts, bundles, tunnels, fixation, and harvest. J Am Acad Orthop Surg. 2008;16(7):376-384.
35. Sabat D, Kumar V. Nerve injury during hamstring graft harvest: a prospective comparative study of three different incisions. Knee Surg Sports Traumatol Arthrosc. 2013;21(9):2089-2095.
36. Kjaergaard J, Faunø LZ, Faunø P. Sensibility loss after ACL reconstruction with hamstring graft. Int J Sports Med. 2008;29(6):507-511.
37. Slone HS, Romine SE, Premkumar A, Xerogeanes JW. Quadriceps tendon autograft for anterior cruciate ligament reconstruction: a comprehensive review of current literature and systematic review of clinical results. Arthroscopy. 2015;31(3):541-554.
38. Nikolaou PK, Seaber AV, Glisson RR, Ribbeck BM, Bassett FH 3rd. Anterior cruciate ligament allograft transplantation. Long-term function, histology, revascularization, and operative technique. Am J Sports Med. 1986;14(5):348-360.
39. Arnoczky SP, Warren RF, Ashlock MA. Replacement of the anterior cruciate ligament using a patellar tendon allograft. An experimental study. J Bone Joint Surg Am. 1986;68(3):376-385.
40. Scheffler SU, Schmidt T, Gangéy I, Dustmann M, Unterhauser F, Weiler A. Fresh-frozen free-tendon allografts versus autografts in anterior cruciate ligament reconstruction: delayed remodeling and inferior mechanical function during long-term healing in sheep. Arthroscopy. 2008;24(4):448-458.
41. Muramatsu K, Hachiya Y, Izawa H. Serial evaluation of human anterior cruciate ligament grafts by contrast-enhanced magnetic resonance imaging: comparison of allografts and autografts. Arthroscopy. 2008;24(9):1038-1044.
42. Jackson DW, Grood ES, Arnoczky SP, Butler DL, Simon TM. Freeze dried anterior cruciate ligament allografts. Preliminary studies in a goat model. Am J Sports Med. 1987;15(4):295-303.
43. Chang SK, Egami DK, Shaieb MD, Kan DM, Richardson AB. Anterior cruciate ligament reconstruction: allograft versus autograft. Arthroscopy. 2003;19(5):453-462.
44. Poehling GG, Curl WW, Lee CA, et al. Analysis of outcomes of anterior cruciate ligament repair with 5-year follow-up: allograft versus autograft. Arthroscopy. 2005;21(7):774-785.
45. Kaeding CC, Pedroza AD, Reinke EK, Huston LJ; MOON Consortium, Spindler KP. Risk factors and predictors of subsequent ACL injury in either knee after ACL reconstruction: prospective analysis of 2488 primary ACL reconstructions from the MOON cohort. Am J Sports Med. 2015;43(7):1583-1590.
46. Kaeding CC, Aros B, Pedroza A, et al. Allograft versus autograft anterior cruciate ligament reconstruction: predictors of failure from a MOON prospective longitudinal cohort. Sports Health. 2011;3(1):73-81.
47. Lynch TS, Parker RD, Patel RM, et al. The impact of the Multicenter Orthopaedic Outcomes Network (MOON) research on anterior cruciate ligament reconstruction and orthopaedic practice. J Am Acad Orthop Surg. 2015;23(3):154-163.
48. Hettrich CM, Dunn WR, Reinke EK; MOON Group, Spindler KP. The rate of subsequent surgery and predictors after anterior cruciate ligament reconstruction: two- and 6-year follow-up results from a multicenter cohort. Am J Sports Med. 2013;41(7):1534-1540.
49. Steadman JR, Matheny LM, Hurst JM, Briggs KK. Patient-centered outcomes and revision rate in patients undergoing ACL reconstruction using bone-patellar tendon-bone autograft compared with bone-patellar tendon-bone allograft: a matched case-control study. Arthroscopy. 2015;31(12):2320-2326.
50. Lenehan EA, Payne WB, Askam BM, Grana WA, Farrow LD. Long-term outcomes of allograft reconstruction of the anterior cruciate ligament. Am J Orthop. 2015;44(5):217-222.
51. Noh JH, Yi SR, Song SJ, Kim SW, Kim W. Comparison between hamstring autograft and free tendon achilles allograft: minimum 2-year follow-up after anterior cruciate ligament reconstruction using EndoButton and Intrafix. Knee Surg Sports Traumatol Arthrosc. 2011;19(5):816-822.
52. Victor J, Bellemans J, Witvrouw E, Govaers K, Fabry G. Graft selection in anterior cruciate ligament reconstruction--prospective analysis of patellar tendon autografts compared with allografts. Int Orthop. 1997;21(2):93-97.
53. Kleipool AE, Zijl JA, Willems WJ. Arthroscopic anterior cruciate ligament reconstruction with bone-patellar tendon-bone allograft or autograft. A prospective study with an average follow up of 4 years. Knee Surg Sports Traumatol Arthrosc. 1998;6(4):224-230.
54. Peterson RK, Shelton WR, Bomboy AL. Allograft versus autograft patellar tendon anterior cruciate ligament reconstruction: a 5-year follow-up. Arthroscopy. 2001;17(1):9-13.
55. Edgar CM, Zimmer S, Kakar S, Jones H, Schepsis AA. Prospective comparison of auto and allograft hamstring tendon constructs for ACL reconstruction. Clin Orthop Relat Res. 2008;466(9):2238-2246.
56. Sun K, Tian S, Zhang J, Xia C, Zhang C, Yu T. Anterior cruciate ligament reconstruction with BPTB autograft, irradiated versus non-irradiated allograft: a prospective randomized clinical study. Knee Surg Sports Traumatol Arthrosc. 2009;17(5):464-474.
57. Leal-Blanquet J, Alentorn-Geli E, Tuneu J, Valentí JR, Maestro A. Anterior cruciate ligament reconstruction: a multicenter prospective cohort study evaluating 3 different grafts using same bone drilling method. Clin J Sport Med. 2011;21(4):294-300.
58. Sun K, Zhang J, Wang Y, et al. Arthroscopic reconstruction of the anterior cruciate ligament with hamstring tendon autograft and fresh-frozen allograft: a prospective, randomized controlled study. Am J Sports Med. 2011;39(7):1430-1438.
59. Lawhorn KW, Howell SM, Traina SM, Gottlieb JE, Meade TD, Freedberg HI. The effect of graft tissue on anterior cruciate ligament outcomes: a multicenter, prospective, randomized controlled trial comparing autograft hamstrings with fresh-frozen anterior tibialis allograft. Arthroscopy. 2012;28(8):1079-1086.
60. Guo L, Yang L, Duan XJ, et al. Anterior cruciate ligament reconstruction with bone-patellar tendon-bone graft: comparison of autograft, fresh-frozen allograft, and γ-irradiated allograft. Arthroscopy. 2012;28(2):211-217.
61. Lamblin CJ, Waterman BR, Lubowitz JH. Anterior cruciate ligament reconstruction with autografts compared with non-irradiated, non-chemically treated allografts. Arthroscopy. 2013;29(6):1113-1122.
62. Mayr HO, Willkomm D, Stoehr A, et al. Revision of anterior cruciate ligament reconstruction with patellar tendon allograft and autograft: 2- and 5-year results. Arch Orthop Trauma Surg. 2012;132(6):867-874.
63. Mariscalco MW, Magnussen RA, Mehta D, Hewett TE, Flanigan DC, Kaeding CC. Autograft versus nonirradiated allograft tissue for anterior cruciate ligament reconstruction: a systematic review. Am J Sports Med. 2014;42(2):492-499.
64. Mehta VM, Mandala C, Foster D, Petsche TS. Comparison of revision rates in bone-patella tendon-bone autograft and allograft anterior cruciate ligament reconstruction. Orthopedics. 2010;33(1):12.
65. Vangsness CT Jr, Garcia IA, Mills CR, Kainer MA, Roberts MR, Moore TM. Allograft transplantation in the knee: tissue regulation, procurement, processing, and sterilization. Am J Sports Med. 2003;31(3):474-481.
66. Barrett GR, Luber K, Replogle WH, Manley JL. Allograft anterior cruciate ligament reconstruction in the young, active patient: tegner activity level and failure rate. Arthroscopy. 2010;26(12):1593-1601.
67. Reagan J, Kullar R, Burks R. MPFL reconstruction: technique and results. Clin Sports Med. 2014;33(3):501-516.
68. Christiansen SE, Jacobsen BW, Lund B, Lind M. Reconstruction of the medial patellofemoral ligament with gracilis tendon autograft in transverse patellar drill holes. Arthroscopy. 2008;24(1):82-87.
69. Schöttle PB, Fucentese SF, Romero J. Clinical and radiological outcome of medial patellofemoral ligament reconstruction with a semitendinosus autograft for patella instability. Knee Surg Sports Traumatol Arthrosc. 2005;13(7):516-521.
70. Deie M, Ochi M, Sumen Y, Adachi N, Kobayashi K, Yasumoto M. A long-term follow-up study after medial patellofemoral ligament reconstruction using the transferred semitendinosus tendon for patellar dislocation. Knee Surg Sports Traumatol Arthrosc. 2005;13(7):522-528.
71. Nomura E, Inoue M. Hybrid medial patellofemoral ligament reconstruction using the semitendinous tendon for recurrent patellar dislocation: minimum 3 years’ follow-up. Arthroscopy. 2006;22(7):787-793.
72. Nomura E, Inoue M. Surgical technique and rationale for medial patellofemoral ligament reconstruction for recurrent patellar dislocation. Arthroscopy. 2003;19(5):E47.
73. Hautamaa PV, Fithian DC, Kaufman KR, Daniel DM, Pohlmeyer AM. Medial soft tissue restraints in lateral patellar instability and repair. Clin Orthop Relat Res. 1998;(349):174-182.
74. Drez D Jr, Edwards TB, Williams CS. Results of medial patellofemoral ligament reconstruction in the treatment of patellar dislocation. Arthroscopy. 2001;17(3):298-306.
75. Fink C, Veselko M, Herbort M, Hoser C. MPFL reconstruction using a quadriceps tendon graft: part 2: operative technique and short term clinical results. Knee. 2014;21(6):1175-1179.
76. Calvo Rodríguez R, Figueroa Poblete D, Anastasiadis Le Roy Z, Etchegaray Bascur F, Vaisman Burucker A, Calvo Mena R. Reconstruction of the medial patellofemoral ligament: evaluation of the clinical results of autografts versus allografts. Rev Esp Cir Ortop Traumatol. 2015;59(5):348-353.
77. Becher C, Kley K, Lobenhoffer P, Ezechieli M, Smith T, Ostermeier S. Dynamic versus static reconstruction of the medial patellofemoral ligament for recurrent lateral patellar dislocation. Knee Surg Sports Traumatol Arthrosc. 2014;22(10):2452-2457.
78. Gomes JE. Comparison between a static and a dynamic technique for medial patellofemoral ligament reconstruction. Arthroscopy. 2008;24(4):430-435.
Musculoskeletal allografts are becoming increasingly accepted as a viable alternative to autografts in a variety of orthopedic procedures. A 2006 American Orthopaedic Society for Sports Medicine (AOSSM) survey indicated that 86% of the participating 365 orthopedic surgeons use allografts in their practice.1 Although the overwhelming majority of orthopedic surgeons use allografts, they share common concerns, including safety, tissue integrity, and biologic incorporation. It is essential for the orthopedic surgeon to understand the current standards of tissue banking, risks and benefits related to the use of allografts, and common indications for safe use in clinical practice. This article reviews the current status of musculoskeletal allografts, including tissue procurement and processing, infections, complications, and specific uses tailored to ligament reconstruction.
Donor Bank, Processing, Sterilization, and Regulation
In the United States, the American Association of Tissue Banks (AATB) is responsible for establishing the standards for more than 100 accredited tissue banks. These tissue banks recover tissue from approximately 30,000 donors annually and account for an estimated 90% of the available musculoskeletal allografts used in the United States. While not all tissue banks are accredited by the AATB, all are required to register with the Food and Drug Administration (FDA), which allows for unannounced inspections of any facility. Facilities are required to abide by the FDA-implemented Current Good Tissue Practices (CGTP), which encompasses regulations on all donor tissue collected after May 2005 to help prevent the transmission of communicable diseases. The FDA released an updated draft in January 2009 that emphasizes safe practices and regulations spanning from environmental control to specific equipment.2
The safety of a transplanted allograft tissue begins within the tissue bank. Donor screening and testing is the first step in reducing the risk of transmission. Screening consists of collecting medical and social history from the family and any healthcare resources to assess the eligibility of the donor. If prior blood donations or autopsy information is available, that information is scrutinized. Donor tissue undergoes nucleic acid testing (NAT), which is required by both the AATB and FDA. All donor tissue must be screened for both types of human immunodeficiency virus (HIV), hepatitis B virus (HBV), hepatitis C virus (HCV), treponema pallidum, and human transmissible spongiform encephalopathies.3 NAT of donor tissue effectively reduces the risk of viral transmission. Additionally, routine preprocessing swabs for bacterial and fungal cultures are performed, although the sensitivity of these cultures ranges from 78% to 92%.4
After donor screening and testing, allograft tissues are usually obtained under aseptic conditions, though this is not FDA-required.5 Once procured, the tissue undergoes sterilization. Currently, there is no standard method ubiquitous to all tissue banks, nor does the FDA require a specific method. Rather, the FDA and AATB require tissue banks to validate their sterilization process and provide supporting data. The goal of sterilization is to inactivate viruses and eradicate bacteria while maintaining the biological and mechanical properties of the tissue. The AATB requires a Sterility Assurance Level (SAL) of 10-6, meaning there is no more than one in a million chance that a nonviral viable microbe exists on or within the tissue. Sterilization techniques may include both radiation and a variety of chemical reagents. Gamma irradiation is a commonly used method of sterilizing soft tissue allografts, although some studies indicate that it is detrimental to tissue biology.6 Newer methods of sterilization are being tested, one of which includes carbon dioxide in combination with antioxidants and irradiation. Bui and colleagues7 directly compared the biomechanical and histological properties of allograft tissue after either the standard 25 kGy gamma irradiation or supercritical carbon dioxide techniques. Although there is no histological difference, the samples treated with supercritical carbon dioxide had less biomechanical damage.7 Finally, the terminally sterilized allograft tissue is frozen to temperatures between -40°C and -80°C.5
Infections
One major concern of allografts is the risk of disease transmission. While numerous studies have investigated the incidence of bacterial infection following transplantation of allograft tissue, there are challenges associated with differentiating common postoperative infections from ones directly associated with the transmission of bacteria within the graft. There is a wide array of reported incidences of infection in the literature, from the Tomford and colleagues8 1981 study that reported a 6.9% rateto the 2001 study by Munting and colleagues,9 who reported 0% in their series. Multiple confounding variables exist, such as possible contamination during handling of an otherwise noncontaminated or properly sterilized allograft with inappropriate inclusion of all postoperative infections. In contrast, recognizing viral transmission has been somewhat easier, although reporting of these incidences has been variable in the past. In either case, there is no accredited reporting system for infections related to allografts.
Bacterial Transmission
Clostridium species. Clostridium species are commonly found among intestinal flora. There is a general consensus that between 24 to 48 hours after death intestinal flora transmigrates into the surrounding tissue and blood. Therefore, a commonly accepted recommendation is that cadaveric tissue needs to be excised prior to 24 hours postmortem.10
In 2001, a 23-year-old man underwent reconstructive knee surgery with a femoral condyle allograft. A few days after surgery, he became septic and ultimately died from the infection. Clostridium sordellii was cultured from the tissue. Several days later, a 17-year-old boy underwent reconstructive knee surgery with a fresh femoral condyle and frozen meniscus from the same donor. Twenty-four hours after surgery, he developed a fever and was readmitted a week later for presumed infection and treated effectively with penicillin and ampicillin/sulbactam. Tissue from the same cadaveric donor had been transplanted into 7 other patients without reports of infection. In a 2002 Centers for Disease Control and Prevention (CDC) update report,11 there were 26 total bacterial cases from allografts and 13 cases were attributed to Clostridium. Malinin and colleagues10 reviewed 795 consecutive cadaveric donors and found that 64 (8.1%) had positive cultures for Clostridia. Of all the positive cultures for Clostridia, 81.3% had positive blood cultures, 57.8% had positive bone marrow aspirate cultures, and 46.9% had positive tissue cultures. They concluded that multiple cultures are required for cadaveric tissue donors in order to reach a higher sensitivity for Clostridial contamination, and these should be done routinely to guide the sterilization process.
Strep species. In 2003, a 17-year-old boy underwent anterior cruciate ligament (ACL) reconstruction with a patellar tendon allograft.12 About 1 week later, he was admitted for signs of infection and received intravenous antibiotics. He required surgical debridement, and intraoperative cultures grew Group A Streptococcus (GAS) that was also identified in the postmortem donor cultures. The tissues underwent processing in an antimicrobial solution and postprocessing cultures were negative for bacteria, but they were not sterilized. Tissues from this donor had been implanted in 5 other patients without report of infection. Following this event, recommendations have been made for prompt rejection of tissue with cultures positive for GAS, unless a sterilizing procedure is used.
Other bacteria. According to the 2002 CDC update, 11 of the 26 cases of bacterial infection reported to the agency were a combination of gram-negative bacilli, polymicrobial flora, or culture negative.11
Viral Transmission
The most effective way to prevent transmission of a viral disease from allografts is thorough donor screening. Since the AATB implemented NAT in 2005 for HIV and HCV, there have been no reported cases of transmission.3 Even prior to this, regular blood screening along with social questionnaires completed by donors or donor families eliminated high-risk donors and significantly decreased the rate of transmission.
Human Immunodeficiency Virus. The first reported case of HIV transmission via implantation of allograft was in 1988. Further investigation revealed that there were 8 transmissions between 1984 and 1986, when routine screening of donors had not yet been implemented. The last reported case of HIV transmission occurred in 1996 with an untested donor.13Hepatitis C Virus. There are several reported cases of HCV transmission that occurred where the donors initially tested negative for HCV. In one case, 40 allografts from the same donor were transplanted over a period of nearly 2 years. This resulted in at least 8 patients being infected with HCV.14 Another case of HCV transmission was reported in 2005 after a patient developed acute HCV 6 weeks after transplantation of a patellar tendon allograft. Further investigation revealed that there had been 3 additional cases over a year from the same donor. Researchers determined that if the initial case had been reported, at least 3 transmissions could have been prevented.15Human T-cell Lymphotropic Virus (HTLV).The first reported transmission of HTLV was in 1991. This was reported in an asymptomatic patient who received a femoral head allograft from a donor who had been previously infected via a blood transfusion.16Zika virus. With recent outbreaks of the Zika virus, the FDA recently released recommendations regarding the screening and deferral of donors, mainly for blood transfusion. Orthopedists should take into consideration the potential for transmission through allografts. The FDA states that all potential donors should be screened for Zika virus using questionnaires and whole blood tests. Symptomatic donors are deferred at least 4 weeks following resolution of symptoms. While this is a recent recommendation from the FDA, orthopedists must be cognizant of the potential harms from this unfamiliar and evolving situation.17
Graft Specifics
Anterior Cruciate Ligament
ACL reconstruction is one of the most commonly performed surgeries by orthopedic surgeons, with an estimated 200,000 reconstructions per year.18Despite the popularity of this surgery, controversies remain regarding the optimal graft for reconstruction.19,20 One would provide adequate strength, be readily available, not elicit an immunologic response from the host, rapidly incorporate, elicit low morbidity, and vascularize early. Current options include both autografts and allografts. Common autograft options include patellar bone-tendon-bone (PBTB), hamstrings tendon, quadriceps tendon, and iliotibial band. PBTB autograft remains a common choice among orthopedic surgeons, as it allows early incorporation of the graft into bone and eliminates immune rejection. However, donor site morbidity, including anterior knee pain, weakness of knee extension, joint stiffness, increased postoperative pain, and iatrogenic patella fractures, have been reported in the literature.21 Commonly used allograft options include donor bone-patellar tendon-bone, quadriceps tendon, Achilles tendon, anterior and posterior tibialis tendons, hamstring tendons, and iliotibial band. Allografts provide the advantage of avoiding donor site morbidity, being readily available, allowing for shorter operative times, and providing lower postoperative pain compared to autografts, although they carry the risk of disease transmission, rejection, and slower incorporation into bone.22-27
Autograft donor site morbidities. One of the general disadvantages of autografts is the donor site morbidity associated with harvesting the grafts. In specific, PBTB grafts allow for bony blocks on both ends of the graft to incorporate into the host bone. However, this technique comes with the risk of disrupting the extensor mechanism.28,29 Milankov and colleagues30 published a retrospective review of over 2000 ACLs using autologous PBTB graft. They noted a 0.45% incidence of patella fracture and 0.18% patellar tendon rupture.30 Others have reported that intraoperative repair of the patellar tendon after tendon harvesting can increase infrapatellar fibrosis, thus increasing the risk for stiffness.31-33
Hamstring autografts include the semitendinosus and the gracilis tendons. The harvesting process is technically demanding and can be complicated by inadvertent amputation of the tendons, making the graft unsuitable for reconstructive purposes.34 Additionally, several reports have identified persistent numbness and hyperesthesia following hamstring harvesting due to iatrogenic injury to the prepatellar branches of the saphenous nerve.35,36A comprehensive review by Slone and colleagues37 reported comparable functional outcomes with quadriceps tendon autograft compared to PBTB; however, this comes with the risk of postoperative hematoma formation and the potential for thigh compartment syndrome.
Biology and Biomechanics of Allografts
One of the major disadvantages of allografts is the reduced ability to incorporate into the host tissue. Several in vitro and animal studies have suggested that allografts incorporate in the host slower than autografts.24,26,38 Early studies by Jackson and colleagues24 on goat models demonstrated that allografts and autografts have similar structural and biological properties initially, but allografts display significantly slower incorporation into the host tissue at 6 months. Histologically, allografts demonstrated lower revascularization, a smaller cross-sectional area, and a prolonged inflammatory response at 6 months postoperatively.24,39,40 Muramatsu and colleagues41 further showed through the use of magnetic resonance imaging a slower rate of revascularization of allografts over 2 years post-reconstruction.
Acknowledging these limitations, one should use caution when choosing to use an allograft or starting aggressive early rehabilitation after an allograft reconstruction, especially in athletes and young patients.
Clinical Outcomes
Although in vitro studies demonstrate inferior strength and delayed incorporation of allografts in the early postoperative period, there is still controversy surrounding the clinical and functional outcomes. Numerous studies have identified allografts as a viable option for ACL reconstruction, with similar reported patient satisfaction scores compared to autografts.43,44
The MOON Consortium recently published a prospective study of nearly 2500 subjects looking to identify risk factors for failure of ACL reconstruction. The study found that allografts had an odds ratio for failure 5.2 times that of PBTB autografts, correlating this factor to an increased re-tear rate of 6.9% in the allograft group compared to 3.2% in the PBTB group (P < .01).45 The elevated risk is more prevalent in younger patients, especially athletic teenagers. This issue has been reiterated in multiple studies.45-50A meta-analysis by Hu and colleagues23 identified 9 studies, either randomized control trials or prospective cohort studies, that looked at clinical outcomes between the different graft choices. They showed there was no significant difference between graft options in terms of instrumental laxity (P = .59), Lachman test (P = .41), pivot shift test (P = .88), and multiple functional outcome scores, including the International Knee Documentation Committee (IKDC), Lysholm, and Tegner scores.23,51-59Processing and sterilization techniques are thought to play a role in allograft failure. Guo and other researchers have demonstrated a significantly higher rate of failure for patients who received gamma-irradiated allografts compared to fresh frozen allografts.23,58-64 With improved sterilization techniques and a strict selection process of donors, gamma radiation has fallen out of favor to protect the biological characteristics of the tissue graft.5,65,66Several factors need to be considered when selecting between allograft or autograft tissue for ligamentous reconstruction. The selection must be balanced between the surgeon’s experience, patient and surgeon preferences, age of the patient, level of physical activity, primary or revision surgical setting, multiligamentous failure, geographical availability of donor grafts, and economical factors.
Medial Patellofemoral Ligament Reconstruction
Another relatively recent application for allografts has been described for the reconstruction of the medial patellofemoral ligament (MPFL) in recurrent lateral patellar dislocations.67-74
Typically, MPFL reconstructions make use of autografts, including quadriceps tendon, patellar tendon, and hamstring ligaments. However, allografts have the potential to limit postoperative donor site morbidity and to allow a faster rehabilitation.75,76 Allografts include semitendinosus, gracilis, anterior tibialis, posterior tibialis, and quadriceps tendons.
Calvo Rodríguez and colleagues76 performed a retrospective review in 2015 comparing allografts to autografts for MPFL reconstruction with respect to postoperative knee function and re-dislocation rates. Among the collective 28 patients, there was no difference in overall functional scores or dislocation rates between the grafts. Although this was a retrospective review and had a small number of subjects, the findings identify allografts as a reliable graft option for MPFL reconstruction.76While there has been a surge of interest in techniques for MPFL reconstruction, there is limited research available regarding the superiority of allografts compared to autografts. For this specific application, it seems that clinical outcomes correlate more to adequate stabilization of the patellofemoral joint than to the type of graft used.77,78 Future research should be dedicated to prospective randomized control trials to delineate any disadvantages to using allografts for MPFL reconstruction.
Discussion
Musculoskeletal allografts are gaining popularity for ligamentous reconstruction as their safety and efficacy continue to improve. With the great majority of tissue banks being accredited by the AATB and specific regulations such as NAT screening becoming common practice, infection rates and transmission of diseases have become incredibly rare. However, a thorough consideration needs to be taken into account when choosing between autograft and allograft on a case-by-case basis (Table).
Am J Orthop. 2016;45(7):446-453. Copyright Frontline Medical Communications Inc. 2016. All rights reserved.
Musculoskeletal allografts are becoming increasingly accepted as a viable alternative to autografts in a variety of orthopedic procedures. A 2006 American Orthopaedic Society for Sports Medicine (AOSSM) survey indicated that 86% of the participating 365 orthopedic surgeons use allografts in their practice.1 Although the overwhelming majority of orthopedic surgeons use allografts, they share common concerns, including safety, tissue integrity, and biologic incorporation. It is essential for the orthopedic surgeon to understand the current standards of tissue banking, risks and benefits related to the use of allografts, and common indications for safe use in clinical practice. This article reviews the current status of musculoskeletal allografts, including tissue procurement and processing, infections, complications, and specific uses tailored to ligament reconstruction.
Donor Bank, Processing, Sterilization, and Regulation
In the United States, the American Association of Tissue Banks (AATB) is responsible for establishing the standards for more than 100 accredited tissue banks. These tissue banks recover tissue from approximately 30,000 donors annually and account for an estimated 90% of the available musculoskeletal allografts used in the United States. While not all tissue banks are accredited by the AATB, all are required to register with the Food and Drug Administration (FDA), which allows for unannounced inspections of any facility. Facilities are required to abide by the FDA-implemented Current Good Tissue Practices (CGTP), which encompasses regulations on all donor tissue collected after May 2005 to help prevent the transmission of communicable diseases. The FDA released an updated draft in January 2009 that emphasizes safe practices and regulations spanning from environmental control to specific equipment.2
The safety of a transplanted allograft tissue begins within the tissue bank. Donor screening and testing is the first step in reducing the risk of transmission. Screening consists of collecting medical and social history from the family and any healthcare resources to assess the eligibility of the donor. If prior blood donations or autopsy information is available, that information is scrutinized. Donor tissue undergoes nucleic acid testing (NAT), which is required by both the AATB and FDA. All donor tissue must be screened for both types of human immunodeficiency virus (HIV), hepatitis B virus (HBV), hepatitis C virus (HCV), treponema pallidum, and human transmissible spongiform encephalopathies.3 NAT of donor tissue effectively reduces the risk of viral transmission. Additionally, routine preprocessing swabs for bacterial and fungal cultures are performed, although the sensitivity of these cultures ranges from 78% to 92%.4
After donor screening and testing, allograft tissues are usually obtained under aseptic conditions, though this is not FDA-required.5 Once procured, the tissue undergoes sterilization. Currently, there is no standard method ubiquitous to all tissue banks, nor does the FDA require a specific method. Rather, the FDA and AATB require tissue banks to validate their sterilization process and provide supporting data. The goal of sterilization is to inactivate viruses and eradicate bacteria while maintaining the biological and mechanical properties of the tissue. The AATB requires a Sterility Assurance Level (SAL) of 10-6, meaning there is no more than one in a million chance that a nonviral viable microbe exists on or within the tissue. Sterilization techniques may include both radiation and a variety of chemical reagents. Gamma irradiation is a commonly used method of sterilizing soft tissue allografts, although some studies indicate that it is detrimental to tissue biology.6 Newer methods of sterilization are being tested, one of which includes carbon dioxide in combination with antioxidants and irradiation. Bui and colleagues7 directly compared the biomechanical and histological properties of allograft tissue after either the standard 25 kGy gamma irradiation or supercritical carbon dioxide techniques. Although there is no histological difference, the samples treated with supercritical carbon dioxide had less biomechanical damage.7 Finally, the terminally sterilized allograft tissue is frozen to temperatures between -40°C and -80°C.5
Infections
One major concern of allografts is the risk of disease transmission. While numerous studies have investigated the incidence of bacterial infection following transplantation of allograft tissue, there are challenges associated with differentiating common postoperative infections from ones directly associated with the transmission of bacteria within the graft. There is a wide array of reported incidences of infection in the literature, from the Tomford and colleagues8 1981 study that reported a 6.9% rateto the 2001 study by Munting and colleagues,9 who reported 0% in their series. Multiple confounding variables exist, such as possible contamination during handling of an otherwise noncontaminated or properly sterilized allograft with inappropriate inclusion of all postoperative infections. In contrast, recognizing viral transmission has been somewhat easier, although reporting of these incidences has been variable in the past. In either case, there is no accredited reporting system for infections related to allografts.
Bacterial Transmission
Clostridium species. Clostridium species are commonly found among intestinal flora. There is a general consensus that between 24 to 48 hours after death intestinal flora transmigrates into the surrounding tissue and blood. Therefore, a commonly accepted recommendation is that cadaveric tissue needs to be excised prior to 24 hours postmortem.10
In 2001, a 23-year-old man underwent reconstructive knee surgery with a femoral condyle allograft. A few days after surgery, he became septic and ultimately died from the infection. Clostridium sordellii was cultured from the tissue. Several days later, a 17-year-old boy underwent reconstructive knee surgery with a fresh femoral condyle and frozen meniscus from the same donor. Twenty-four hours after surgery, he developed a fever and was readmitted a week later for presumed infection and treated effectively with penicillin and ampicillin/sulbactam. Tissue from the same cadaveric donor had been transplanted into 7 other patients without reports of infection. In a 2002 Centers for Disease Control and Prevention (CDC) update report,11 there were 26 total bacterial cases from allografts and 13 cases were attributed to Clostridium. Malinin and colleagues10 reviewed 795 consecutive cadaveric donors and found that 64 (8.1%) had positive cultures for Clostridia. Of all the positive cultures for Clostridia, 81.3% had positive blood cultures, 57.8% had positive bone marrow aspirate cultures, and 46.9% had positive tissue cultures. They concluded that multiple cultures are required for cadaveric tissue donors in order to reach a higher sensitivity for Clostridial contamination, and these should be done routinely to guide the sterilization process.
Strep species. In 2003, a 17-year-old boy underwent anterior cruciate ligament (ACL) reconstruction with a patellar tendon allograft.12 About 1 week later, he was admitted for signs of infection and received intravenous antibiotics. He required surgical debridement, and intraoperative cultures grew Group A Streptococcus (GAS) that was also identified in the postmortem donor cultures. The tissues underwent processing in an antimicrobial solution and postprocessing cultures were negative for bacteria, but they were not sterilized. Tissues from this donor had been implanted in 5 other patients without report of infection. Following this event, recommendations have been made for prompt rejection of tissue with cultures positive for GAS, unless a sterilizing procedure is used.
Other bacteria. According to the 2002 CDC update, 11 of the 26 cases of bacterial infection reported to the agency were a combination of gram-negative bacilli, polymicrobial flora, or culture negative.11
Viral Transmission
The most effective way to prevent transmission of a viral disease from allografts is thorough donor screening. Since the AATB implemented NAT in 2005 for HIV and HCV, there have been no reported cases of transmission.3 Even prior to this, regular blood screening along with social questionnaires completed by donors or donor families eliminated high-risk donors and significantly decreased the rate of transmission.
Human Immunodeficiency Virus. The first reported case of HIV transmission via implantation of allograft was in 1988. Further investigation revealed that there were 8 transmissions between 1984 and 1986, when routine screening of donors had not yet been implemented. The last reported case of HIV transmission occurred in 1996 with an untested donor.13Hepatitis C Virus. There are several reported cases of HCV transmission that occurred where the donors initially tested negative for HCV. In one case, 40 allografts from the same donor were transplanted over a period of nearly 2 years. This resulted in at least 8 patients being infected with HCV.14 Another case of HCV transmission was reported in 2005 after a patient developed acute HCV 6 weeks after transplantation of a patellar tendon allograft. Further investigation revealed that there had been 3 additional cases over a year from the same donor. Researchers determined that if the initial case had been reported, at least 3 transmissions could have been prevented.15Human T-cell Lymphotropic Virus (HTLV).The first reported transmission of HTLV was in 1991. This was reported in an asymptomatic patient who received a femoral head allograft from a donor who had been previously infected via a blood transfusion.16Zika virus. With recent outbreaks of the Zika virus, the FDA recently released recommendations regarding the screening and deferral of donors, mainly for blood transfusion. Orthopedists should take into consideration the potential for transmission through allografts. The FDA states that all potential donors should be screened for Zika virus using questionnaires and whole blood tests. Symptomatic donors are deferred at least 4 weeks following resolution of symptoms. While this is a recent recommendation from the FDA, orthopedists must be cognizant of the potential harms from this unfamiliar and evolving situation.17
Graft Specifics
Anterior Cruciate Ligament
ACL reconstruction is one of the most commonly performed surgeries by orthopedic surgeons, with an estimated 200,000 reconstructions per year.18Despite the popularity of this surgery, controversies remain regarding the optimal graft for reconstruction.19,20 One would provide adequate strength, be readily available, not elicit an immunologic response from the host, rapidly incorporate, elicit low morbidity, and vascularize early. Current options include both autografts and allografts. Common autograft options include patellar bone-tendon-bone (PBTB), hamstrings tendon, quadriceps tendon, and iliotibial band. PBTB autograft remains a common choice among orthopedic surgeons, as it allows early incorporation of the graft into bone and eliminates immune rejection. However, donor site morbidity, including anterior knee pain, weakness of knee extension, joint stiffness, increased postoperative pain, and iatrogenic patella fractures, have been reported in the literature.21 Commonly used allograft options include donor bone-patellar tendon-bone, quadriceps tendon, Achilles tendon, anterior and posterior tibialis tendons, hamstring tendons, and iliotibial band. Allografts provide the advantage of avoiding donor site morbidity, being readily available, allowing for shorter operative times, and providing lower postoperative pain compared to autografts, although they carry the risk of disease transmission, rejection, and slower incorporation into bone.22-27
Autograft donor site morbidities. One of the general disadvantages of autografts is the donor site morbidity associated with harvesting the grafts. In specific, PBTB grafts allow for bony blocks on both ends of the graft to incorporate into the host bone. However, this technique comes with the risk of disrupting the extensor mechanism.28,29 Milankov and colleagues30 published a retrospective review of over 2000 ACLs using autologous PBTB graft. They noted a 0.45% incidence of patella fracture and 0.18% patellar tendon rupture.30 Others have reported that intraoperative repair of the patellar tendon after tendon harvesting can increase infrapatellar fibrosis, thus increasing the risk for stiffness.31-33
Hamstring autografts include the semitendinosus and the gracilis tendons. The harvesting process is technically demanding and can be complicated by inadvertent amputation of the tendons, making the graft unsuitable for reconstructive purposes.34 Additionally, several reports have identified persistent numbness and hyperesthesia following hamstring harvesting due to iatrogenic injury to the prepatellar branches of the saphenous nerve.35,36A comprehensive review by Slone and colleagues37 reported comparable functional outcomes with quadriceps tendon autograft compared to PBTB; however, this comes with the risk of postoperative hematoma formation and the potential for thigh compartment syndrome.
Biology and Biomechanics of Allografts
One of the major disadvantages of allografts is the reduced ability to incorporate into the host tissue. Several in vitro and animal studies have suggested that allografts incorporate in the host slower than autografts.24,26,38 Early studies by Jackson and colleagues24 on goat models demonstrated that allografts and autografts have similar structural and biological properties initially, but allografts display significantly slower incorporation into the host tissue at 6 months. Histologically, allografts demonstrated lower revascularization, a smaller cross-sectional area, and a prolonged inflammatory response at 6 months postoperatively.24,39,40 Muramatsu and colleagues41 further showed through the use of magnetic resonance imaging a slower rate of revascularization of allografts over 2 years post-reconstruction.
Acknowledging these limitations, one should use caution when choosing to use an allograft or starting aggressive early rehabilitation after an allograft reconstruction, especially in athletes and young patients.
Clinical Outcomes
Although in vitro studies demonstrate inferior strength and delayed incorporation of allografts in the early postoperative period, there is still controversy surrounding the clinical and functional outcomes. Numerous studies have identified allografts as a viable option for ACL reconstruction, with similar reported patient satisfaction scores compared to autografts.43,44
The MOON Consortium recently published a prospective study of nearly 2500 subjects looking to identify risk factors for failure of ACL reconstruction. The study found that allografts had an odds ratio for failure 5.2 times that of PBTB autografts, correlating this factor to an increased re-tear rate of 6.9% in the allograft group compared to 3.2% in the PBTB group (P < .01).45 The elevated risk is more prevalent in younger patients, especially athletic teenagers. This issue has been reiterated in multiple studies.45-50A meta-analysis by Hu and colleagues23 identified 9 studies, either randomized control trials or prospective cohort studies, that looked at clinical outcomes between the different graft choices. They showed there was no significant difference between graft options in terms of instrumental laxity (P = .59), Lachman test (P = .41), pivot shift test (P = .88), and multiple functional outcome scores, including the International Knee Documentation Committee (IKDC), Lysholm, and Tegner scores.23,51-59Processing and sterilization techniques are thought to play a role in allograft failure. Guo and other researchers have demonstrated a significantly higher rate of failure for patients who received gamma-irradiated allografts compared to fresh frozen allografts.23,58-64 With improved sterilization techniques and a strict selection process of donors, gamma radiation has fallen out of favor to protect the biological characteristics of the tissue graft.5,65,66Several factors need to be considered when selecting between allograft or autograft tissue for ligamentous reconstruction. The selection must be balanced between the surgeon’s experience, patient and surgeon preferences, age of the patient, level of physical activity, primary or revision surgical setting, multiligamentous failure, geographical availability of donor grafts, and economical factors.
Medial Patellofemoral Ligament Reconstruction
Another relatively recent application for allografts has been described for the reconstruction of the medial patellofemoral ligament (MPFL) in recurrent lateral patellar dislocations.67-74
Typically, MPFL reconstructions make use of autografts, including quadriceps tendon, patellar tendon, and hamstring ligaments. However, allografts have the potential to limit postoperative donor site morbidity and to allow a faster rehabilitation.75,76 Allografts include semitendinosus, gracilis, anterior tibialis, posterior tibialis, and quadriceps tendons.
Calvo Rodríguez and colleagues76 performed a retrospective review in 2015 comparing allografts to autografts for MPFL reconstruction with respect to postoperative knee function and re-dislocation rates. Among the collective 28 patients, there was no difference in overall functional scores or dislocation rates between the grafts. Although this was a retrospective review and had a small number of subjects, the findings identify allografts as a reliable graft option for MPFL reconstruction.76While there has been a surge of interest in techniques for MPFL reconstruction, there is limited research available regarding the superiority of allografts compared to autografts. For this specific application, it seems that clinical outcomes correlate more to adequate stabilization of the patellofemoral joint than to the type of graft used.77,78 Future research should be dedicated to prospective randomized control trials to delineate any disadvantages to using allografts for MPFL reconstruction.
Discussion
Musculoskeletal allografts are gaining popularity for ligamentous reconstruction as their safety and efficacy continue to improve. With the great majority of tissue banks being accredited by the AATB and specific regulations such as NAT screening becoming common practice, infection rates and transmission of diseases have become incredibly rare. However, a thorough consideration needs to be taken into account when choosing between autograft and allograft on a case-by-case basis (Table).
Am J Orthop. 2016;45(7):446-453. Copyright Frontline Medical Communications Inc. 2016. All rights reserved.
1. The American Orthopaedic Society for Sports Medicine. Allografts for ACL Reconstruction Survey Report. 2013. http://www.sportsmed.org/AOSSMIMIS/members/downloads/research/AllograftACLReconstructionSurveyReport.pdf. Accessed October 21, 2016.
2. US Department of Health and Human Services, Food and Drug Administration. Guidance for industry: Current good tissue practice (CGTP) and additional requirements for manufacturers of human cells, tissues, and cellular and tissue-based products (HCT/Ps). http://www.fda.gov/downloads/BiologicsBloodVaccines/GuidanceComplianceRegulatoryInformation/Guidances/Tissue/UCM285223.pdf. Published December 2011. Accessed August 17, 2015.
3. Vaishnav S, Thomas Vangsness C Jr, Dellamaggiora R. New techniques in allograft tissue processing. Clin Sports Med. 2009;28(1):127-141.
4. Veen MR, Bloem RM, Petit PL. Sensitivity and negative predictive value of swab cultures in musculoskeletal allograft procurement. Clin Orthop Relat Res. 1994;(300):259-263.
5. McAllister DR, Joyce MJ, Mann BJ, Vangsness CT Jr. Allograft update: the current status of tissue regulation, procurement, processing, and sterilization. Am J Sports Med. 2007;35(12):2148-2158.
6. Mickiewicz P, Binkowski M, Bursig H, Wróbel Z. Preservation and sterilization methods of the meniscal allografts: literature review. Cell Tissue Bank. 2014;15(3):307-317.
7. Bui D, Lovric V, Oliver R, Bertollo N, Broe D, Walsh WR. Meniscal allograft sterilisation: effect on biomechanical and histological properties. Cell Tissue Bank. 2015;16(3):467-475.
8. Tomford WW, Starkweather RJ, Goldman MH. A study of the clinical incidence of infection in the use of banked allograft bone. J Bone Joint Surg Am. 1981;63(2):244-248.
9. Munting E, Faundez A, Manche E. Vertebral reconstruction with cortical allograft: long-term evaluation. Eur Spine J. 2001;10 Suppl 2:S153-S157.
10. Malinin TI, Buck BE, Temple HT, Martinez OV, Fox WP. Incidence of clostridial contamination in donors’ musculoskeletal tissue. J Bone Joint Surg Br. 2003;85(7):1051-1054.
11. Centers for Disease Control and Prevention (CDC). Update: allograft-associated bacterial infections--United States, 2002. MMWR Morb Mortal Wkly Rep. 2002;51(10):207-210.
12. Centers for Disease Control and Prevention (CDC). Invasive Streptococcus pyogenes after allograft implantation--Colorado, 2003. MMWR Morb Mortal Wkly Rep. 2003;52(48):1174-1176.
13. Hinsenkamp M, Muylle L, Eastlund T, Fehily D, Noël L, Strong DM. Adverse reactions and events related to musculoskeletal allografts: reviewed by the World Health Organisation project NOTIFY. Int Orthop. 2012;36(3):633-641.
14. Schratt HE, Regel G, Kiesewetter B, Tscherne H. HIV infection caused by cold preserved bone transplants. Unfallchirurg. 1996;99(9):679-684.
15. Tugwell BD, Patel PR, Williams IT, et al. Transmission of hepatitis C virus to several organ and tissue recipients from an antibody-negative donor. Ann Intern Med. 2005;143(9):648-654.
16. Sanzén L, Carlsson A. Transmission of human T-cell lymphotrophic virus type 1 by a deep-frozen bone allograft. Acta Orthop Scand. 1997;68(1):72-74.
17. US Department of Health and Human Services, Food and Drug Administration. Recommendations for donor screening, deferral, and product management to reduce the risk of transfusion-transmission of Zika virus. Guidance for industry. http://www.fda.gov/downloads/BiologicsBloodVaccines/GuidanceComplianceRegulatoryInformation/Guidances/Blood/UCM486360.pdf. Published February 2016. Accessed August 10, 2016.
18. Gottlob CA, Baker CL Jr, Pellissier JM, Colvin L. Cost effectiveness of anterior cruciate ligament reconstruction in young adults. Clin Orthop Relat Res. 1999;(367):272-282.
19. Fu F, Christel P, Miller MD, Johnson DL. Graft selection for anterior cruciate ligament reconstruction. Instr Course Lect. 2009;58:337-354.
20. Chechik O, Amar E, Khashan M, Lador R, Eyal G, Gold A. An international survey on anterior cruciate ligament reconstruction practices. Int Orthop. 2013;37(2):201-206.
21. Spindler KP, Kuhn JE, Freedman KB, Matthews CE, Dittus RS, Harrell FE Jr. Anterior cruciate ligament reconstruction autograft choice: bone-tendon-bone versus hamstring: does it really matter? A systematic review. Am J Sports Med. 2004;32(8):1986-1995.
22. Harner CD, Irrgang JJ, Paul J, Dearwater S, Fu FH. Loss of motion after anterior cruciate ligament reconstruction. Am J Sports Med. 1992;20(5):499-506.
23. Hu J, Qu J, Xu D, Zhou J, Lu H. Allograft versus autograft for anterior cruciate ligament reconstruction: an up-to-date meta-analysis of prospective studies. Int Orthop. 2013;37(2):311-320.
24. Jackson DW, Grood ES, Goldstein JD, et al. A comparison of patellar tendon autograft and allograft used for anterior cruciate ligament reconstruction in the goat model. Am J Sports Med. 1993;21(2):176-185.
25. Mroz TE, Joyce MJ, Steinmetz MP, Lieberman IH, Wang JC. Musculoskeletal allograft risks and recalls in the United States. J Am Acad Orthop Surg. 2008;16(10):559-565.
26. Malinin TI, Levitt RL, Bashore C, Temple HT, Mnaymneh W. A study of retrieved allografts used to replace anterior cruciate ligaments. Arthroscopy. 2002;18(2):163-170.
27. Foster TE, Wolfe BL, Ryan S, Silvestri L, Kaye EK. Does the graft source really matter in the outcome of patients undergoing anterior cruciate ligament reconstruction? An evaluation of autograft versus allograft reconstruction results: a systematic review. Am J Sports Med. 2010;38(1):189-199.
28. Lee GH, McCulloch P, Cole BJ, Bush-Joseph CA, Bach BR Jr. The incidence of acute patellar tendon harvest complications for anterior cruciate ligament reconstruction. Arthroscopy. 2008;24(2):162-166.
29. Marumoto JM, Mitsunaga MM, Richardson AB, Medoff RJ, Mayfield GW. Late patellar tendon ruptures after removal of the central third for anterior cruciate ligament reconstruction. A report of two cases. Am J Sports Med. 1996;24(5):698-701.
30 Milankov M, Kecojević V, Rasović P, Kovacević N, Gvozdenović N, Obradović M. Disruption of the knee extensor apparatus complicating anterior cruciate ligament reconstruction. Acta Chir Iugosl. 2013;60(2):13-21.
31. Atkinson TS, Atkinson PJ, Mendenhall HV, Haut RC. Patellar tendon and infrapatellar fat pad healing after harvest of an ACL graft. J Surg Res. 1998;79(1):25-30.
32. Tang G, Niitsu M, Ikeda K, Endo H, Itai Y. Fibrous scar in the infrapatellar fat pad after arthroscopy: MR imaging. Radiat Med. 2000;18(1):1-5.
33. Unterhauser FN, Bosch U, Zeichen J, Weiler A. Alpha-smooth muscle actin containing contractile fibroblastic cells in human knee arthrofibrosis tissue. Winner of the AGA-DonJoy Award 2003. Arch Orthop Trauma Surg. 2004;124(9):585-591.
34. Prodromos CC, Fu FH, Howell SM, Johnson DH, Lawhorn K. Controversies in soft-tissue anterior cruciate ligament reconstruction: grafts, bundles, tunnels, fixation, and harvest. J Am Acad Orthop Surg. 2008;16(7):376-384.
35. Sabat D, Kumar V. Nerve injury during hamstring graft harvest: a prospective comparative study of three different incisions. Knee Surg Sports Traumatol Arthrosc. 2013;21(9):2089-2095.
36. Kjaergaard J, Faunø LZ, Faunø P. Sensibility loss after ACL reconstruction with hamstring graft. Int J Sports Med. 2008;29(6):507-511.
37. Slone HS, Romine SE, Premkumar A, Xerogeanes JW. Quadriceps tendon autograft for anterior cruciate ligament reconstruction: a comprehensive review of current literature and systematic review of clinical results. Arthroscopy. 2015;31(3):541-554.
38. Nikolaou PK, Seaber AV, Glisson RR, Ribbeck BM, Bassett FH 3rd. Anterior cruciate ligament allograft transplantation. Long-term function, histology, revascularization, and operative technique. Am J Sports Med. 1986;14(5):348-360.
39. Arnoczky SP, Warren RF, Ashlock MA. Replacement of the anterior cruciate ligament using a patellar tendon allograft. An experimental study. J Bone Joint Surg Am. 1986;68(3):376-385.
40. Scheffler SU, Schmidt T, Gangéy I, Dustmann M, Unterhauser F, Weiler A. Fresh-frozen free-tendon allografts versus autografts in anterior cruciate ligament reconstruction: delayed remodeling and inferior mechanical function during long-term healing in sheep. Arthroscopy. 2008;24(4):448-458.
41. Muramatsu K, Hachiya Y, Izawa H. Serial evaluation of human anterior cruciate ligament grafts by contrast-enhanced magnetic resonance imaging: comparison of allografts and autografts. Arthroscopy. 2008;24(9):1038-1044.
42. Jackson DW, Grood ES, Arnoczky SP, Butler DL, Simon TM. Freeze dried anterior cruciate ligament allografts. Preliminary studies in a goat model. Am J Sports Med. 1987;15(4):295-303.
43. Chang SK, Egami DK, Shaieb MD, Kan DM, Richardson AB. Anterior cruciate ligament reconstruction: allograft versus autograft. Arthroscopy. 2003;19(5):453-462.
44. Poehling GG, Curl WW, Lee CA, et al. Analysis of outcomes of anterior cruciate ligament repair with 5-year follow-up: allograft versus autograft. Arthroscopy. 2005;21(7):774-785.
45. Kaeding CC, Pedroza AD, Reinke EK, Huston LJ; MOON Consortium, Spindler KP. Risk factors and predictors of subsequent ACL injury in either knee after ACL reconstruction: prospective analysis of 2488 primary ACL reconstructions from the MOON cohort. Am J Sports Med. 2015;43(7):1583-1590.
46. Kaeding CC, Aros B, Pedroza A, et al. Allograft versus autograft anterior cruciate ligament reconstruction: predictors of failure from a MOON prospective longitudinal cohort. Sports Health. 2011;3(1):73-81.
47. Lynch TS, Parker RD, Patel RM, et al. The impact of the Multicenter Orthopaedic Outcomes Network (MOON) research on anterior cruciate ligament reconstruction and orthopaedic practice. J Am Acad Orthop Surg. 2015;23(3):154-163.
48. Hettrich CM, Dunn WR, Reinke EK; MOON Group, Spindler KP. The rate of subsequent surgery and predictors after anterior cruciate ligament reconstruction: two- and 6-year follow-up results from a multicenter cohort. Am J Sports Med. 2013;41(7):1534-1540.
49. Steadman JR, Matheny LM, Hurst JM, Briggs KK. Patient-centered outcomes and revision rate in patients undergoing ACL reconstruction using bone-patellar tendon-bone autograft compared with bone-patellar tendon-bone allograft: a matched case-control study. Arthroscopy. 2015;31(12):2320-2326.
50. Lenehan EA, Payne WB, Askam BM, Grana WA, Farrow LD. Long-term outcomes of allograft reconstruction of the anterior cruciate ligament. Am J Orthop. 2015;44(5):217-222.
51. Noh JH, Yi SR, Song SJ, Kim SW, Kim W. Comparison between hamstring autograft and free tendon achilles allograft: minimum 2-year follow-up after anterior cruciate ligament reconstruction using EndoButton and Intrafix. Knee Surg Sports Traumatol Arthrosc. 2011;19(5):816-822.
52. Victor J, Bellemans J, Witvrouw E, Govaers K, Fabry G. Graft selection in anterior cruciate ligament reconstruction--prospective analysis of patellar tendon autografts compared with allografts. Int Orthop. 1997;21(2):93-97.
53. Kleipool AE, Zijl JA, Willems WJ. Arthroscopic anterior cruciate ligament reconstruction with bone-patellar tendon-bone allograft or autograft. A prospective study with an average follow up of 4 years. Knee Surg Sports Traumatol Arthrosc. 1998;6(4):224-230.
54. Peterson RK, Shelton WR, Bomboy AL. Allograft versus autograft patellar tendon anterior cruciate ligament reconstruction: a 5-year follow-up. Arthroscopy. 2001;17(1):9-13.
55. Edgar CM, Zimmer S, Kakar S, Jones H, Schepsis AA. Prospective comparison of auto and allograft hamstring tendon constructs for ACL reconstruction. Clin Orthop Relat Res. 2008;466(9):2238-2246.
56. Sun K, Tian S, Zhang J, Xia C, Zhang C, Yu T. Anterior cruciate ligament reconstruction with BPTB autograft, irradiated versus non-irradiated allograft: a prospective randomized clinical study. Knee Surg Sports Traumatol Arthrosc. 2009;17(5):464-474.
57. Leal-Blanquet J, Alentorn-Geli E, Tuneu J, Valentí JR, Maestro A. Anterior cruciate ligament reconstruction: a multicenter prospective cohort study evaluating 3 different grafts using same bone drilling method. Clin J Sport Med. 2011;21(4):294-300.
58. Sun K, Zhang J, Wang Y, et al. Arthroscopic reconstruction of the anterior cruciate ligament with hamstring tendon autograft and fresh-frozen allograft: a prospective, randomized controlled study. Am J Sports Med. 2011;39(7):1430-1438.
59. Lawhorn KW, Howell SM, Traina SM, Gottlieb JE, Meade TD, Freedberg HI. The effect of graft tissue on anterior cruciate ligament outcomes: a multicenter, prospective, randomized controlled trial comparing autograft hamstrings with fresh-frozen anterior tibialis allograft. Arthroscopy. 2012;28(8):1079-1086.
60. Guo L, Yang L, Duan XJ, et al. Anterior cruciate ligament reconstruction with bone-patellar tendon-bone graft: comparison of autograft, fresh-frozen allograft, and γ-irradiated allograft. Arthroscopy. 2012;28(2):211-217.
61. Lamblin CJ, Waterman BR, Lubowitz JH. Anterior cruciate ligament reconstruction with autografts compared with non-irradiated, non-chemically treated allografts. Arthroscopy. 2013;29(6):1113-1122.
62. Mayr HO, Willkomm D, Stoehr A, et al. Revision of anterior cruciate ligament reconstruction with patellar tendon allograft and autograft: 2- and 5-year results. Arch Orthop Trauma Surg. 2012;132(6):867-874.
63. Mariscalco MW, Magnussen RA, Mehta D, Hewett TE, Flanigan DC, Kaeding CC. Autograft versus nonirradiated allograft tissue for anterior cruciate ligament reconstruction: a systematic review. Am J Sports Med. 2014;42(2):492-499.
64. Mehta VM, Mandala C, Foster D, Petsche TS. Comparison of revision rates in bone-patella tendon-bone autograft and allograft anterior cruciate ligament reconstruction. Orthopedics. 2010;33(1):12.
65. Vangsness CT Jr, Garcia IA, Mills CR, Kainer MA, Roberts MR, Moore TM. Allograft transplantation in the knee: tissue regulation, procurement, processing, and sterilization. Am J Sports Med. 2003;31(3):474-481.
66. Barrett GR, Luber K, Replogle WH, Manley JL. Allograft anterior cruciate ligament reconstruction in the young, active patient: tegner activity level and failure rate. Arthroscopy. 2010;26(12):1593-1601.
67. Reagan J, Kullar R, Burks R. MPFL reconstruction: technique and results. Clin Sports Med. 2014;33(3):501-516.
68. Christiansen SE, Jacobsen BW, Lund B, Lind M. Reconstruction of the medial patellofemoral ligament with gracilis tendon autograft in transverse patellar drill holes. Arthroscopy. 2008;24(1):82-87.
69. Schöttle PB, Fucentese SF, Romero J. Clinical and radiological outcome of medial patellofemoral ligament reconstruction with a semitendinosus autograft for patella instability. Knee Surg Sports Traumatol Arthrosc. 2005;13(7):516-521.
70. Deie M, Ochi M, Sumen Y, Adachi N, Kobayashi K, Yasumoto M. A long-term follow-up study after medial patellofemoral ligament reconstruction using the transferred semitendinosus tendon for patellar dislocation. Knee Surg Sports Traumatol Arthrosc. 2005;13(7):522-528.
71. Nomura E, Inoue M. Hybrid medial patellofemoral ligament reconstruction using the semitendinous tendon for recurrent patellar dislocation: minimum 3 years’ follow-up. Arthroscopy. 2006;22(7):787-793.
72. Nomura E, Inoue M. Surgical technique and rationale for medial patellofemoral ligament reconstruction for recurrent patellar dislocation. Arthroscopy. 2003;19(5):E47.
73. Hautamaa PV, Fithian DC, Kaufman KR, Daniel DM, Pohlmeyer AM. Medial soft tissue restraints in lateral patellar instability and repair. Clin Orthop Relat Res. 1998;(349):174-182.
74. Drez D Jr, Edwards TB, Williams CS. Results of medial patellofemoral ligament reconstruction in the treatment of patellar dislocation. Arthroscopy. 2001;17(3):298-306.
75. Fink C, Veselko M, Herbort M, Hoser C. MPFL reconstruction using a quadriceps tendon graft: part 2: operative technique and short term clinical results. Knee. 2014;21(6):1175-1179.
76. Calvo Rodríguez R, Figueroa Poblete D, Anastasiadis Le Roy Z, Etchegaray Bascur F, Vaisman Burucker A, Calvo Mena R. Reconstruction of the medial patellofemoral ligament: evaluation of the clinical results of autografts versus allografts. Rev Esp Cir Ortop Traumatol. 2015;59(5):348-353.
77. Becher C, Kley K, Lobenhoffer P, Ezechieli M, Smith T, Ostermeier S. Dynamic versus static reconstruction of the medial patellofemoral ligament for recurrent lateral patellar dislocation. Knee Surg Sports Traumatol Arthrosc. 2014;22(10):2452-2457.
78. Gomes JE. Comparison between a static and a dynamic technique for medial patellofemoral ligament reconstruction. Arthroscopy. 2008;24(4):430-435.
1. The American Orthopaedic Society for Sports Medicine. Allografts for ACL Reconstruction Survey Report. 2013. http://www.sportsmed.org/AOSSMIMIS/members/downloads/research/AllograftACLReconstructionSurveyReport.pdf. Accessed October 21, 2016.
2. US Department of Health and Human Services, Food and Drug Administration. Guidance for industry: Current good tissue practice (CGTP) and additional requirements for manufacturers of human cells, tissues, and cellular and tissue-based products (HCT/Ps). http://www.fda.gov/downloads/BiologicsBloodVaccines/GuidanceComplianceRegulatoryInformation/Guidances/Tissue/UCM285223.pdf. Published December 2011. Accessed August 17, 2015.
3. Vaishnav S, Thomas Vangsness C Jr, Dellamaggiora R. New techniques in allograft tissue processing. Clin Sports Med. 2009;28(1):127-141.
4. Veen MR, Bloem RM, Petit PL. Sensitivity and negative predictive value of swab cultures in musculoskeletal allograft procurement. Clin Orthop Relat Res. 1994;(300):259-263.
5. McAllister DR, Joyce MJ, Mann BJ, Vangsness CT Jr. Allograft update: the current status of tissue regulation, procurement, processing, and sterilization. Am J Sports Med. 2007;35(12):2148-2158.
6. Mickiewicz P, Binkowski M, Bursig H, Wróbel Z. Preservation and sterilization methods of the meniscal allografts: literature review. Cell Tissue Bank. 2014;15(3):307-317.
7. Bui D, Lovric V, Oliver R, Bertollo N, Broe D, Walsh WR. Meniscal allograft sterilisation: effect on biomechanical and histological properties. Cell Tissue Bank. 2015;16(3):467-475.
8. Tomford WW, Starkweather RJ, Goldman MH. A study of the clinical incidence of infection in the use of banked allograft bone. J Bone Joint Surg Am. 1981;63(2):244-248.
9. Munting E, Faundez A, Manche E. Vertebral reconstruction with cortical allograft: long-term evaluation. Eur Spine J. 2001;10 Suppl 2:S153-S157.
10. Malinin TI, Buck BE, Temple HT, Martinez OV, Fox WP. Incidence of clostridial contamination in donors’ musculoskeletal tissue. J Bone Joint Surg Br. 2003;85(7):1051-1054.
11. Centers for Disease Control and Prevention (CDC). Update: allograft-associated bacterial infections--United States, 2002. MMWR Morb Mortal Wkly Rep. 2002;51(10):207-210.
12. Centers for Disease Control and Prevention (CDC). Invasive Streptococcus pyogenes after allograft implantation--Colorado, 2003. MMWR Morb Mortal Wkly Rep. 2003;52(48):1174-1176.
13. Hinsenkamp M, Muylle L, Eastlund T, Fehily D, Noël L, Strong DM. Adverse reactions and events related to musculoskeletal allografts: reviewed by the World Health Organisation project NOTIFY. Int Orthop. 2012;36(3):633-641.
14. Schratt HE, Regel G, Kiesewetter B, Tscherne H. HIV infection caused by cold preserved bone transplants. Unfallchirurg. 1996;99(9):679-684.
15. Tugwell BD, Patel PR, Williams IT, et al. Transmission of hepatitis C virus to several organ and tissue recipients from an antibody-negative donor. Ann Intern Med. 2005;143(9):648-654.
16. Sanzén L, Carlsson A. Transmission of human T-cell lymphotrophic virus type 1 by a deep-frozen bone allograft. Acta Orthop Scand. 1997;68(1):72-74.
17. US Department of Health and Human Services, Food and Drug Administration. Recommendations for donor screening, deferral, and product management to reduce the risk of transfusion-transmission of Zika virus. Guidance for industry. http://www.fda.gov/downloads/BiologicsBloodVaccines/GuidanceComplianceRegulatoryInformation/Guidances/Blood/UCM486360.pdf. Published February 2016. Accessed August 10, 2016.
18. Gottlob CA, Baker CL Jr, Pellissier JM, Colvin L. Cost effectiveness of anterior cruciate ligament reconstruction in young adults. Clin Orthop Relat Res. 1999;(367):272-282.
19. Fu F, Christel P, Miller MD, Johnson DL. Graft selection for anterior cruciate ligament reconstruction. Instr Course Lect. 2009;58:337-354.
20. Chechik O, Amar E, Khashan M, Lador R, Eyal G, Gold A. An international survey on anterior cruciate ligament reconstruction practices. Int Orthop. 2013;37(2):201-206.
21. Spindler KP, Kuhn JE, Freedman KB, Matthews CE, Dittus RS, Harrell FE Jr. Anterior cruciate ligament reconstruction autograft choice: bone-tendon-bone versus hamstring: does it really matter? A systematic review. Am J Sports Med. 2004;32(8):1986-1995.
22. Harner CD, Irrgang JJ, Paul J, Dearwater S, Fu FH. Loss of motion after anterior cruciate ligament reconstruction. Am J Sports Med. 1992;20(5):499-506.
23. Hu J, Qu J, Xu D, Zhou J, Lu H. Allograft versus autograft for anterior cruciate ligament reconstruction: an up-to-date meta-analysis of prospective studies. Int Orthop. 2013;37(2):311-320.
24. Jackson DW, Grood ES, Goldstein JD, et al. A comparison of patellar tendon autograft and allograft used for anterior cruciate ligament reconstruction in the goat model. Am J Sports Med. 1993;21(2):176-185.
25. Mroz TE, Joyce MJ, Steinmetz MP, Lieberman IH, Wang JC. Musculoskeletal allograft risks and recalls in the United States. J Am Acad Orthop Surg. 2008;16(10):559-565.
26. Malinin TI, Levitt RL, Bashore C, Temple HT, Mnaymneh W. A study of retrieved allografts used to replace anterior cruciate ligaments. Arthroscopy. 2002;18(2):163-170.
27. Foster TE, Wolfe BL, Ryan S, Silvestri L, Kaye EK. Does the graft source really matter in the outcome of patients undergoing anterior cruciate ligament reconstruction? An evaluation of autograft versus allograft reconstruction results: a systematic review. Am J Sports Med. 2010;38(1):189-199.
28. Lee GH, McCulloch P, Cole BJ, Bush-Joseph CA, Bach BR Jr. The incidence of acute patellar tendon harvest complications for anterior cruciate ligament reconstruction. Arthroscopy. 2008;24(2):162-166.
29. Marumoto JM, Mitsunaga MM, Richardson AB, Medoff RJ, Mayfield GW. Late patellar tendon ruptures after removal of the central third for anterior cruciate ligament reconstruction. A report of two cases. Am J Sports Med. 1996;24(5):698-701.
30 Milankov M, Kecojević V, Rasović P, Kovacević N, Gvozdenović N, Obradović M. Disruption of the knee extensor apparatus complicating anterior cruciate ligament reconstruction. Acta Chir Iugosl. 2013;60(2):13-21.
31. Atkinson TS, Atkinson PJ, Mendenhall HV, Haut RC. Patellar tendon and infrapatellar fat pad healing after harvest of an ACL graft. J Surg Res. 1998;79(1):25-30.
32. Tang G, Niitsu M, Ikeda K, Endo H, Itai Y. Fibrous scar in the infrapatellar fat pad after arthroscopy: MR imaging. Radiat Med. 2000;18(1):1-5.
33. Unterhauser FN, Bosch U, Zeichen J, Weiler A. Alpha-smooth muscle actin containing contractile fibroblastic cells in human knee arthrofibrosis tissue. Winner of the AGA-DonJoy Award 2003. Arch Orthop Trauma Surg. 2004;124(9):585-591.
34. Prodromos CC, Fu FH, Howell SM, Johnson DH, Lawhorn K. Controversies in soft-tissue anterior cruciate ligament reconstruction: grafts, bundles, tunnels, fixation, and harvest. J Am Acad Orthop Surg. 2008;16(7):376-384.
35. Sabat D, Kumar V. Nerve injury during hamstring graft harvest: a prospective comparative study of three different incisions. Knee Surg Sports Traumatol Arthrosc. 2013;21(9):2089-2095.
36. Kjaergaard J, Faunø LZ, Faunø P. Sensibility loss after ACL reconstruction with hamstring graft. Int J Sports Med. 2008;29(6):507-511.
37. Slone HS, Romine SE, Premkumar A, Xerogeanes JW. Quadriceps tendon autograft for anterior cruciate ligament reconstruction: a comprehensive review of current literature and systematic review of clinical results. Arthroscopy. 2015;31(3):541-554.
38. Nikolaou PK, Seaber AV, Glisson RR, Ribbeck BM, Bassett FH 3rd. Anterior cruciate ligament allograft transplantation. Long-term function, histology, revascularization, and operative technique. Am J Sports Med. 1986;14(5):348-360.
39. Arnoczky SP, Warren RF, Ashlock MA. Replacement of the anterior cruciate ligament using a patellar tendon allograft. An experimental study. J Bone Joint Surg Am. 1986;68(3):376-385.
40. Scheffler SU, Schmidt T, Gangéy I, Dustmann M, Unterhauser F, Weiler A. Fresh-frozen free-tendon allografts versus autografts in anterior cruciate ligament reconstruction: delayed remodeling and inferior mechanical function during long-term healing in sheep. Arthroscopy. 2008;24(4):448-458.
41. Muramatsu K, Hachiya Y, Izawa H. Serial evaluation of human anterior cruciate ligament grafts by contrast-enhanced magnetic resonance imaging: comparison of allografts and autografts. Arthroscopy. 2008;24(9):1038-1044.
42. Jackson DW, Grood ES, Arnoczky SP, Butler DL, Simon TM. Freeze dried anterior cruciate ligament allografts. Preliminary studies in a goat model. Am J Sports Med. 1987;15(4):295-303.
43. Chang SK, Egami DK, Shaieb MD, Kan DM, Richardson AB. Anterior cruciate ligament reconstruction: allograft versus autograft. Arthroscopy. 2003;19(5):453-462.
44. Poehling GG, Curl WW, Lee CA, et al. Analysis of outcomes of anterior cruciate ligament repair with 5-year follow-up: allograft versus autograft. Arthroscopy. 2005;21(7):774-785.
45. Kaeding CC, Pedroza AD, Reinke EK, Huston LJ; MOON Consortium, Spindler KP. Risk factors and predictors of subsequent ACL injury in either knee after ACL reconstruction: prospective analysis of 2488 primary ACL reconstructions from the MOON cohort. Am J Sports Med. 2015;43(7):1583-1590.
46. Kaeding CC, Aros B, Pedroza A, et al. Allograft versus autograft anterior cruciate ligament reconstruction: predictors of failure from a MOON prospective longitudinal cohort. Sports Health. 2011;3(1):73-81.
47. Lynch TS, Parker RD, Patel RM, et al. The impact of the Multicenter Orthopaedic Outcomes Network (MOON) research on anterior cruciate ligament reconstruction and orthopaedic practice. J Am Acad Orthop Surg. 2015;23(3):154-163.
48. Hettrich CM, Dunn WR, Reinke EK; MOON Group, Spindler KP. The rate of subsequent surgery and predictors after anterior cruciate ligament reconstruction: two- and 6-year follow-up results from a multicenter cohort. Am J Sports Med. 2013;41(7):1534-1540.
49. Steadman JR, Matheny LM, Hurst JM, Briggs KK. Patient-centered outcomes and revision rate in patients undergoing ACL reconstruction using bone-patellar tendon-bone autograft compared with bone-patellar tendon-bone allograft: a matched case-control study. Arthroscopy. 2015;31(12):2320-2326.
50. Lenehan EA, Payne WB, Askam BM, Grana WA, Farrow LD. Long-term outcomes of allograft reconstruction of the anterior cruciate ligament. Am J Orthop. 2015;44(5):217-222.
51. Noh JH, Yi SR, Song SJ, Kim SW, Kim W. Comparison between hamstring autograft and free tendon achilles allograft: minimum 2-year follow-up after anterior cruciate ligament reconstruction using EndoButton and Intrafix. Knee Surg Sports Traumatol Arthrosc. 2011;19(5):816-822.
52. Victor J, Bellemans J, Witvrouw E, Govaers K, Fabry G. Graft selection in anterior cruciate ligament reconstruction--prospective analysis of patellar tendon autografts compared with allografts. Int Orthop. 1997;21(2):93-97.
53. Kleipool AE, Zijl JA, Willems WJ. Arthroscopic anterior cruciate ligament reconstruction with bone-patellar tendon-bone allograft or autograft. A prospective study with an average follow up of 4 years. Knee Surg Sports Traumatol Arthrosc. 1998;6(4):224-230.
54. Peterson RK, Shelton WR, Bomboy AL. Allograft versus autograft patellar tendon anterior cruciate ligament reconstruction: a 5-year follow-up. Arthroscopy. 2001;17(1):9-13.
55. Edgar CM, Zimmer S, Kakar S, Jones H, Schepsis AA. Prospective comparison of auto and allograft hamstring tendon constructs for ACL reconstruction. Clin Orthop Relat Res. 2008;466(9):2238-2246.
56. Sun K, Tian S, Zhang J, Xia C, Zhang C, Yu T. Anterior cruciate ligament reconstruction with BPTB autograft, irradiated versus non-irradiated allograft: a prospective randomized clinical study. Knee Surg Sports Traumatol Arthrosc. 2009;17(5):464-474.
57. Leal-Blanquet J, Alentorn-Geli E, Tuneu J, Valentí JR, Maestro A. Anterior cruciate ligament reconstruction: a multicenter prospective cohort study evaluating 3 different grafts using same bone drilling method. Clin J Sport Med. 2011;21(4):294-300.
58. Sun K, Zhang J, Wang Y, et al. Arthroscopic reconstruction of the anterior cruciate ligament with hamstring tendon autograft and fresh-frozen allograft: a prospective, randomized controlled study. Am J Sports Med. 2011;39(7):1430-1438.
59. Lawhorn KW, Howell SM, Traina SM, Gottlieb JE, Meade TD, Freedberg HI. The effect of graft tissue on anterior cruciate ligament outcomes: a multicenter, prospective, randomized controlled trial comparing autograft hamstrings with fresh-frozen anterior tibialis allograft. Arthroscopy. 2012;28(8):1079-1086.
60. Guo L, Yang L, Duan XJ, et al. Anterior cruciate ligament reconstruction with bone-patellar tendon-bone graft: comparison of autograft, fresh-frozen allograft, and γ-irradiated allograft. Arthroscopy. 2012;28(2):211-217.
61. Lamblin CJ, Waterman BR, Lubowitz JH. Anterior cruciate ligament reconstruction with autografts compared with non-irradiated, non-chemically treated allografts. Arthroscopy. 2013;29(6):1113-1122.
62. Mayr HO, Willkomm D, Stoehr A, et al. Revision of anterior cruciate ligament reconstruction with patellar tendon allograft and autograft: 2- and 5-year results. Arch Orthop Trauma Surg. 2012;132(6):867-874.
63. Mariscalco MW, Magnussen RA, Mehta D, Hewett TE, Flanigan DC, Kaeding CC. Autograft versus nonirradiated allograft tissue for anterior cruciate ligament reconstruction: a systematic review. Am J Sports Med. 2014;42(2):492-499.
64. Mehta VM, Mandala C, Foster D, Petsche TS. Comparison of revision rates in bone-patella tendon-bone autograft and allograft anterior cruciate ligament reconstruction. Orthopedics. 2010;33(1):12.
65. Vangsness CT Jr, Garcia IA, Mills CR, Kainer MA, Roberts MR, Moore TM. Allograft transplantation in the knee: tissue regulation, procurement, processing, and sterilization. Am J Sports Med. 2003;31(3):474-481.
66. Barrett GR, Luber K, Replogle WH, Manley JL. Allograft anterior cruciate ligament reconstruction in the young, active patient: tegner activity level and failure rate. Arthroscopy. 2010;26(12):1593-1601.
67. Reagan J, Kullar R, Burks R. MPFL reconstruction: technique and results. Clin Sports Med. 2014;33(3):501-516.
68. Christiansen SE, Jacobsen BW, Lund B, Lind M. Reconstruction of the medial patellofemoral ligament with gracilis tendon autograft in transverse patellar drill holes. Arthroscopy. 2008;24(1):82-87.
69. Schöttle PB, Fucentese SF, Romero J. Clinical and radiological outcome of medial patellofemoral ligament reconstruction with a semitendinosus autograft for patella instability. Knee Surg Sports Traumatol Arthrosc. 2005;13(7):516-521.
70. Deie M, Ochi M, Sumen Y, Adachi N, Kobayashi K, Yasumoto M. A long-term follow-up study after medial patellofemoral ligament reconstruction using the transferred semitendinosus tendon for patellar dislocation. Knee Surg Sports Traumatol Arthrosc. 2005;13(7):522-528.
71. Nomura E, Inoue M. Hybrid medial patellofemoral ligament reconstruction using the semitendinous tendon for recurrent patellar dislocation: minimum 3 years’ follow-up. Arthroscopy. 2006;22(7):787-793.
72. Nomura E, Inoue M. Surgical technique and rationale for medial patellofemoral ligament reconstruction for recurrent patellar dislocation. Arthroscopy. 2003;19(5):E47.
73. Hautamaa PV, Fithian DC, Kaufman KR, Daniel DM, Pohlmeyer AM. Medial soft tissue restraints in lateral patellar instability and repair. Clin Orthop Relat Res. 1998;(349):174-182.
74. Drez D Jr, Edwards TB, Williams CS. Results of medial patellofemoral ligament reconstruction in the treatment of patellar dislocation. Arthroscopy. 2001;17(3):298-306.
75. Fink C, Veselko M, Herbort M, Hoser C. MPFL reconstruction using a quadriceps tendon graft: part 2: operative technique and short term clinical results. Knee. 2014;21(6):1175-1179.
76. Calvo Rodríguez R, Figueroa Poblete D, Anastasiadis Le Roy Z, Etchegaray Bascur F, Vaisman Burucker A, Calvo Mena R. Reconstruction of the medial patellofemoral ligament: evaluation of the clinical results of autografts versus allografts. Rev Esp Cir Ortop Traumatol. 2015;59(5):348-353.
77. Becher C, Kley K, Lobenhoffer P, Ezechieli M, Smith T, Ostermeier S. Dynamic versus static reconstruction of the medial patellofemoral ligament for recurrent lateral patellar dislocation. Knee Surg Sports Traumatol Arthrosc. 2014;22(10):2452-2457.
78. Gomes JE. Comparison between a static and a dynamic technique for medial patellofemoral ligament reconstruction. Arthroscopy. 2008;24(4):430-435.
Platelet-Rich Plasma Can Be Used to Successfully Treat Elbow Ulnar Collateral Ligament Insufficiency in High-Level Throwers
For overhead athletes, elbow ulnar collateral ligament (UCL) insufficiency is a potential career-ending injury. Baseball players with UCL insufficiency typically complain of medial-sided elbow pain that affects their ability to throw. Loss of velocity, loss of control, difficulty warming up, and pain while throwing are all symptoms of UCL injury.
Classically, nonoperative treatment of UCL injuries involves activity modification, use of anti-inflammatory medication, and a structured physical therapy program. Asymptomatic players can return to throwing after a structured interval throwing program. Rettig and colleagues1 found a 42% rate of success in conservatively treating UCL injuries in throwing athletes. UCL reconstruction is reserved for players with complete tears of the UCL or with partial tears after failed conservative treatment. Several techniques have been used to reconstruct the ligament, but successful outcomes depend on a long rehabilitation process. According to most published series, 85% to 90% of athletes who had UCL reconstruction returned to their previous level of play, but it took, on average, 9 to 12 months.2,3 This prolonged recovery period is one reason that some older professional baseball players, as well as casual high school and college players, elect to forgo surgery.
Over the past few years, platelet-rich plasma (PRP) has garnered attention as a bridge between conservative treatment and surgery. PRP refers to a sample of autologous blood that contains a platelet concentration higher than baseline levels. This sample often has a 3 to 5 times increase in growth factor concentration.4-6 Initial studies focused on its ability to successfully treat lateral epicondylitis.7-9 More recent clinical work has shown that PRP can potentially enhance healing after anterior cruciate ligament reconstruction,10-14 rotator cuff repair,15-17 and subacromial decompression.11,18-23 If PRP could be used to successfully treat UCL insufficiency that is refractory to conservative treatment, then year-long recovery periods could be avoided. This could potentially prolong certain athletes’ careers or, at the very least, allow them to return to play much sooner. In the present case series, we hypothesized that PRP injections could be used to successfully treat partial UCL tears in high-level throwing athletes, obviating the need for surgery and its associated prolonged recovery period.
Materials and Methods
Institutional Review Board approval was obtained for this retrospective study of 44 baseball players treated with PRP injections for partial-thickness UCL tears.
Patients provided written informed consent. They were diagnosed with UCL insufficiency by physical examination, and findings were confirmed by magnetic resonance imaging (MRI). After diagnosis, all throwers underwent a trial of conservative treatment that included rest, activity modification, use of anti-inflammatory medication, and physical therapy followed by an attempt to return to throwing using an interval throwing program.
Study inclusion criteria were physical examinations and MRI results consistent with UCL insufficiency, and failure of the conservative treatment plan described.
Patients were injected using the Autologous Conditioned Plasma system (Arthrex). PRP solutions were prepared according to manufacturer guidelines. After the elbow was prepared sterilely, the UCL was injected at the location of the tear. Typically, 3 mL of PRP was injected into the elbow. Sixteen patients had 1 injection, 6 had 2, and 22 had 3. Repeat injections were considered for recalcitrant pain after 3 weeks.
After injection, patients used acetaminophen and ice for pain control. Anti-inflammatory medications were avoided for a minimum of 2 weeks after injection. Typical postinjection therapy protocol consisted of rest followed by progressive stretching and strengthening for about 4 to 6 weeks before the start of an interval throwing program. Although there is no well-defined postinjection recovery protocol, as a general rule rest was prescribed for the first 2 weeks, followed by a progressive stretching and strengthening program for the next month. Patients who were asymptomatic subjectively and clinically—negative moving valgus stress test, negative milking maneuver, no pain with valgus stress—were started on an interval throwing program.
Final follow-up involved a physical examination. Results were classified according to a modified version of the Conway Scale12,24-26: excellent (return to preinjury level of competition or performance), good (return to play at a lower level of competition or performance or, specifically for baseball players, ability to throw in daily batting practice), fair (able to play recreationally), and poor (unable to return to previous sport at any level).
By final follow-up, all patients had completed their postoperative rehabilitation protocol, and all had at least tried to return to their previous activities. No patients were lost to follow-up.
Results
Of the 44 baseball players, 6 were professional, 14 were in college, and 24 were in high school. There were 36 pitchers and 8 position players. Mean age was 17.3 years (range, 16-28 years). All patients were available for follow-up after injection (mean, 11 months). Fifteen of the 44 players had an excellent outcome (34%), 17 had a good outcome, 2 had a fair outcome, and 10 had a poor outcome. After injection, 4 (67%) of the 6 professional baseball players returned to professional play. Five (36%) of the 14 college players had an excellent outcome, and 4 (17%) of the 24 high school players had an excellent outcome. Of the 8 position players, 4 had an excellent outcome, 3 had a good outcome, and 1 had a poor outcome.
Before treatment, all patients had medial-sided elbow pain over the UCL inhibiting their ability to throw. Mean duration of symptoms before injection was 8.8 months (range, 1-36 months). There was no correlation between symptom duration and any outcome measure. On MRI, 29 patients showed partial tears: 22 proximally based and 7 distally based. The other 15 patients had diffuse signal without partial tear. All 7 patients with distally based partial tears and 3 of the patients with proximally based partial tears had a poor outcome. Overall, there were 6 excellent, 7 good, and 2 fair outcomes in the partial-tear group. In the patients with diffuse signal without partial tear, there were 9 excellent and 10 good outcomes.
Mean time from injection to return to throwing was 5 weeks, and mean time to return to competition was 12 weeks (range, 5-24 weeks). The 1 player who returned at 5 weeks was a professional relief pitcher whose team was in the playoffs. He has now pitched for an additional 2 baseball seasons without elbow difficulty.
There were no injection-related complications.
Discussion
To our knowledge, this is the first report documenting successful PRP treatment of UCL insufficiency. In this study, 73% of players who had failed a course of conservative treatment had good to excellent outcomes with PRP injection.
Data on successful nonoperative treatment of UCL injuries are limited. Rettig and colleagues1 treated 31 throwing athletes’ UCL injuries with a supervised rehabilitation program. Treatment included rest, use of anti-inflammatory medication, progressive strengthening, and an interval throwing program. Only 41% of the athletes returned to their previous level of play, and it took, on average, 24.5 weeks. There was no significant difference in age or in duration or acuity of symptoms between those who returned to play and those whose conservative treatment failed.
Surgical reconstruction of UCL injuries has been very successful, with upward of 90% of athletes returning to previous level of play.3,27The procedure, however, is not without associated complications, including retear of the ligament, stiffness, ulnar nerve injury, and fracture.27-29 In addition, even when successful, the procedure requires that athletes take 9 to 12 months to recover before returning to competition at their previous level.
Savoie and colleagues,30 in their recent study on UCL repairs, highlighted an important fact that is often overlooked when reviewing the literature on UCL tears. Most of the literature on these injuries focuses on college and professional baseball players in whom ligament damage is often extensive, precluding repair. In contrast to prior reports, Savoie and colleagues30 found excellent results in 93% of their young athletes who underwent UCL repair. It is possible that their results can be attributed to the fact that many of their athletes had tears isolated to one area of the ligament, as opposed to generalized ligament incompetence. Our improved results vis-à-vis other reports on conservative management may be attributable to the same phenomenon.
PRP has garnered much attention in the literature and media because of its potential to enhance healing of tendons and ligaments; in some cases, it can obviate the need for surgery. After failure of other nonoperative measures in 15 patients with elbow epicondylitis, Mishra and Pavelko8 treated each patient with a single PRP injection. They prepared the PRP using the GPS III system (Biomet). At final follow-up, 93% improvement was seen. Clearly, their experiment had design flaws: It was nonblinded, and 3 of the 5 patients in the control group treated with bupivacaine injection withdrew from the experiment. Despite its shortcomings, their study became the impetus for several other studies.
A larger, double-blinded, randomized controlled trial comparing PRP and cortisone injections for lateral epicondylitis in 100 patients is under way, and preliminary results have been published.9 A minimum of 6 months after injection, patients who received PRP showed more improvement in visual analog scale (VAS) pain scores and Disabilities of the Arm, Shoulder, and Hand (DASH) questionnaire scores. In another large, double-blinded, randomized controlled trial, patients with chronic lateral epicondylitis had significant improvements in VAS pain scores and DASH scores relative to patients injected with corticosteroids with a 2-year follow-up.31 Similarly, Thanasas and colleagues32 found significantly reduced VAS pain scores in patients injected with PRP versus autologous whole blood. Another study demonstrated improved tendon morphology using ultrasound imaging 6 months after PRP injection.33
Contrary to these positive results, Krogh and colleagues34 found that a single injection of PRP or glucocorticoid was not significantly superior to a saline injection for reducing pain and disability over a 3-month period in patients with lateral epicondylitis. Their study, however, had major flaws. Its original design called for a 12-month follow-up, but there was massive dropout in all 3 treatment arms, necessitating reporting of only 3-month data. In addition, 60% of the patients in the glucocorticoid group were not naïve to this treatment, so definitive conclusions about the efficacy of glucocorticoids could not be made.
In the present study, we successfully treated partial ligament tears with PRP injections. Sixty-seven percent of our baseball players returned to play at a mean of 4 months, much earlier than the 9 to 12 months typically required after ligament reconstruction. Many athletes, such as high school baseball players or aging veteran professional baseball players, do not have the luxury of 12 months for recovery. Therefore, this select group of patients clearly has a limited window of opportunity to return to play. In fact, these patients might be ideal candidates for PRP injections for UCL injuries. Return-to-play rates, however, differed significantly among professional players and nonprofessional players. The difference may be attributable to professional players’ conditioning, quality of physical therapy, extrinsic motivation, and other intangible factors. Four (67%) of our 6 professional baseball players returned to professional play after injection, whereas only 36% of college players and 17% of high school players had excellent outcomes.
Limitations
The present study had several weaknesses, several of which are inherent to PRP studies conducted so far. It was not a prospective, randomized controlled trial. It is important to note that PRP treatment in diseased tissue may have some drawbacks, as its success depends on the ability of healing tissue to use concentrated growth factors and cytokines to proliferate.35 Thus, a chronically injured ligament with depleted active cells may have a diminished response to PRP. Another limitation of this study is that we evaluated outcomes based on return to play using the Conway Scale, which is well reported but not validated. Despite the potential weaknesses of this outcome scale, it has become the benchmark for measuring the success of outcomes of UCL reconstruction. Furthermore, we did not measure patients’ satisfaction with the treatment. Players who could not return to their preinjury level of play may have considered the treatment a failure regardless of their ability to continue throwing. Last, MRI was not repeated to document ligament healing. We did not routinely perform a second MRI because we thought it would not affect treatment. Several series have found a high incidence of abnormal signal in baseball players’ UCLs. In this group of patients, the most important outcome is return to previous level of competition.
This study raised several questions. Is one PRP brand better than another? Should more than 1 injection be given? What is the ideal postinjection protocol? Clearly, larger, prospective, randomized controlled studies are needed to truly elucidate the potential role of PRP in the treatment algorithm for UCL injury. Nevertheless, in certain cases in which traditional conservative measures have failed and patients do not have the luxury of rehabilitating for 9 to 12 months after surgery, PRP may be a viable treatment option.
Conclusion
In this study, use of PRP in the treatment of UCL insufficiency produced outcomes much better than earlier reported outcomes of conservative treatment of these injuries. PRP injections may be particularly beneficial in young athletes who have sustained acute damage to an isolated part of the ligament and in athletes unwilling or unable to undergo the extended rehabilitation required after surgical reconstruction of the ligament.
1. Rettig AC, Sherrill C, Snead DS, Mendler JC, Mieling P. Nonoperative treatment of ulnar collateral ligament injuries in throwing athletes. Am J Sports Med. 2001;29(1):15-17.
2. Eygendaal D, Rahussen FT, Diercks RL. Biomechanics of the elbow joint in tennis players and relation to pathology. Br J Sports Med. 2007;41(11):820-823.
3. Bowers AL, Dines JS, Dines DM, Altchek DW. Elbow medial ulnar collateral ligament reconstruction: clinical relevance and the docking technique. J Shoulder Elbow Surg. 2010;19(2):110-117.
5. Kibler WB. Biomechanical analysis of the shoulder during tennis activities. Clin Sports Med. 1995;14(1):79-85.
5. Marx RE. Platelet-rich plasma: evidence to support its use. J Oral Maxillofac Surg. 2004;62(4):489-496.
6. Marx RE. Platelet-rich plasma (PRP): what is PRP and what is not PRP? Implant Dent. 2001;10(4):225-228.
7. Elliott B, Fleisig G, Nicholls R, Escamilia R. Technique effects on upper limb loading in the tennis serve. J Sci Med Sport. 2003;6(1):76-87.
8. Mishra A, Pavelko T. Treatment of chronic elbow tendinosis with buffered platelet-rich plasma. Am J Sports Med. 2006;34(11):1774-1778.
9. Mishra A, Woodall J Jr, Vieira A. Treatment of tendon and muscle using platelet-rich plasma. Clin Sports Med. 2009;28(1):113-125.
10. Kovacs MS. Applied physiology of tennis performance. Br J Sports Med. 2006;40(5):381-386.
11. Xie X, Wu H, Zhao S, Xie G, Huangfu X, Zhao J. The effect of platelet-rich plasma on patterns of gene expression in a dog model of anterior cruciate ligament reconstruction. J Surg Res. 2013;180(1):80-88.
12. Pluim BM, Staal JB, Windler GE, Jayanthi N. Tennis injuries: occurrence, aetiology, and prevention. Br J Sports Med. 2006;40(5):415-423.
13. Xie X, Zhao S, Wu H, et al. Platelet-rich plasma enhances autograft revascularization and reinnervation in a dog model of anterior cruciate ligament reconstruction. J Surg Res. 2013;183(1):214-222.
14. Lopez-Vidriero E, Goulding KA, Simon DA, Sanchez M, Johnson DH. The use of platelet-rich plasma in arthroscopy and sports medicine: optimizing the healing environment. Arthroscopy. 2010;26(2):269-278.
15. Jo CH, Shin JS, Shin WH, Lee SY, Yoon KS, Shin S. Platelet-rich plasma for arthroscopic repair of medium to large rotator cuff tears: a randomized controlled trial. Am J Sports Med. 2015;43(9):2102-2110.
16. Jo CH, Shin JS, Lee YG, et al. Platelet-rich plasma for arthroscopic repair of large to massive rotator cuff tears: a randomized, single-blinded, parallel-group trial. Am J Sports Med. 2013;41(10):2240-2248.
17. Randelli P, Arrigoni P, Ragone V, Aliprandi A, Cabitza P. Platelet-rich plasma in arthroscopic rotator cuff repair: a prospective RCT study, 2-year follow-up. J Shoulder Elbow Surg. 2011;20(4):518-528.
18. Randelli P, Arrigoni P, Ragone V, Aliprandi A, Cabitza P. Platelet rich plasma in arthroscopic rotator cuff repair: a prospective RCT study, 2-year follow-up. J Shoulder Elbow Surg. 2011;20(4):518-528.
19. Barber FA, Hrnack SA, Snyder SJ, Hapa O. Rotator cuff repair healing influenced by platelet-rich plasma construct augmentation. Arthroscopy. 2011;27(8):1029-1035.
20. Jo CH, Kim JE, Yoon KS, et al. Does platelet-rich plasma accelerate recovery after rotator cuff repair? A prospective cohort study. Am J Sports Med. 2011;39(10):2082-2090.
21. Jo CH, Kim JE, Yoon KS, Shin S. Platelet-rich plasma stimulates cell proliferation and enhances matrix gene expression and synthesis in tenocytes from human rotator cuff tendons with degenerative tears. Am J Sports Med. 2012;40(5):1035-1045.
22. Chahal J, Van Thiel GS, Mall N, et al. The role of platelet-rich plasma in arthroscopic rotator cuff repair: a systematic review with quantitative synthesis. Arthroscopy. 2012;28(11):1718-1727.
23. Mei-Dan O, Carmont MR. The role of platelet-rich plasma in rotator cuff repair. Sports Med Arthrosc Rev. 2011;19(3):244-250.
24. Dines JS, ElAttrache NS, Conway JE, Smith W, Ahmad CS. Clinical outcomes of the DANE TJ technique to treat ulnar collateral ligament insufficiency of the elbow. Am J Sports Med. 2007;35(12):2039-2044.
25. Hutchinson MR, Laprade RF, Burnett QM 2nd, Moss R, Terpstra J. Injury surveillance at the USTA boys’ tennis championships: a 6-yr study. Med Sci Sports Exerc. 1995;27(6):826-830.
26. Winge S, Jørgensen U, Nielsen A. Epidemiology of injuries in Danish championship tennis. Int J Sports Med. 1989;10(5):368-371.
27. Safran MR, Hutchinson MR, Moss R, Albrandt J. A comparison of injuries in elite boys and girls tennis players. Paper presented at: 9th Annual Meeting of the Society of Tennis Medicine and Science; March 1999; Indian Wells, CA.
28. Cain EL, Andrews JR, Dugas JR, et al. Outcome of ulnar collateral ligament reconstruction of the elbow in 1281 athletes: results in 743 athletes with minimum 2-year follow-up. Am J Sports Med. 2010;38(12):2426-2434.
29. Dines JS, Yocum LA, Frank JB, ElAttrache NS, Gambardella RA, Jobe FW. Revision surgery for failed elbow medial collateral ligament reconstruction. Am J Sports Med. 2008;36(6):1061-1065.
30. Savoie FH, Trenhaile SW, Roberts J, Field LD, Ramsey JR. Primary repair of ulnar collateral ligament injuries of the elbow in young athletes: a case series of injuries to the proximal and distal ends of the ligament. Am J Sports Med. 2008;36(6):1066-1072.
31. Gosens T, Peerbooms JC, van Laar W, Oudsten den BL. Ongoing positive effect of platelet-rich plasma versus corticosteroid injection in lateral epicondylitis: a double-blind randomized controlled trial with 2-year follow-up. Am J Sports Med. 2011;39(6):1200-1208.
32. Thanasas C, Papadimitriou G, Charalambidis C, Paraskevopoulos I, Papanikolaou A. Platelet-rich plasma versus autologous whole blood for the treatment of chronic lateral elbow epicondylitis: a randomized controlled clinical trial. Am J Sports Med. 2011;39(10):2130-2134.
33. Chaudhury S, La Lama de M, Adler RS, et al. Platelet-rich plasma for the treatment of lateral epicondylitis: sonographic assessment of tendon morphology and vascularity (pilot study). Skeletal Radiol. 2013;42(1):91-97.
34. Krogh TP, Fredberg U, Stengaard-Pedersen K, Christensen R, Jensen P, Ellingsen T. Treatment of lateral epicondylitis with platelet-rich plasma, glucocorticoid, or saline: a randomized, double-blind, placebo-controlled trial. Am J Sports Med. 2013;41(3):625-635.
35. Anz AW, Hackel JG, Nilssen EC, Andrews JR. Application of biologics in the treatment of the rotator cuff, meniscus, cartilage, and osteoarthritis. J Am Acad Orthop Surg. 2014;22(2):68-79.
For overhead athletes, elbow ulnar collateral ligament (UCL) insufficiency is a potential career-ending injury. Baseball players with UCL insufficiency typically complain of medial-sided elbow pain that affects their ability to throw. Loss of velocity, loss of control, difficulty warming up, and pain while throwing are all symptoms of UCL injury.
Classically, nonoperative treatment of UCL injuries involves activity modification, use of anti-inflammatory medication, and a structured physical therapy program. Asymptomatic players can return to throwing after a structured interval throwing program. Rettig and colleagues1 found a 42% rate of success in conservatively treating UCL injuries in throwing athletes. UCL reconstruction is reserved for players with complete tears of the UCL or with partial tears after failed conservative treatment. Several techniques have been used to reconstruct the ligament, but successful outcomes depend on a long rehabilitation process. According to most published series, 85% to 90% of athletes who had UCL reconstruction returned to their previous level of play, but it took, on average, 9 to 12 months.2,3 This prolonged recovery period is one reason that some older professional baseball players, as well as casual high school and college players, elect to forgo surgery.
Over the past few years, platelet-rich plasma (PRP) has garnered attention as a bridge between conservative treatment and surgery. PRP refers to a sample of autologous blood that contains a platelet concentration higher than baseline levels. This sample often has a 3 to 5 times increase in growth factor concentration.4-6 Initial studies focused on its ability to successfully treat lateral epicondylitis.7-9 More recent clinical work has shown that PRP can potentially enhance healing after anterior cruciate ligament reconstruction,10-14 rotator cuff repair,15-17 and subacromial decompression.11,18-23 If PRP could be used to successfully treat UCL insufficiency that is refractory to conservative treatment, then year-long recovery periods could be avoided. This could potentially prolong certain athletes’ careers or, at the very least, allow them to return to play much sooner. In the present case series, we hypothesized that PRP injections could be used to successfully treat partial UCL tears in high-level throwing athletes, obviating the need for surgery and its associated prolonged recovery period.
Materials and Methods
Institutional Review Board approval was obtained for this retrospective study of 44 baseball players treated with PRP injections for partial-thickness UCL tears.
Patients provided written informed consent. They were diagnosed with UCL insufficiency by physical examination, and findings were confirmed by magnetic resonance imaging (MRI). After diagnosis, all throwers underwent a trial of conservative treatment that included rest, activity modification, use of anti-inflammatory medication, and physical therapy followed by an attempt to return to throwing using an interval throwing program.
Study inclusion criteria were physical examinations and MRI results consistent with UCL insufficiency, and failure of the conservative treatment plan described.
Patients were injected using the Autologous Conditioned Plasma system (Arthrex). PRP solutions were prepared according to manufacturer guidelines. After the elbow was prepared sterilely, the UCL was injected at the location of the tear. Typically, 3 mL of PRP was injected into the elbow. Sixteen patients had 1 injection, 6 had 2, and 22 had 3. Repeat injections were considered for recalcitrant pain after 3 weeks.
After injection, patients used acetaminophen and ice for pain control. Anti-inflammatory medications were avoided for a minimum of 2 weeks after injection. Typical postinjection therapy protocol consisted of rest followed by progressive stretching and strengthening for about 4 to 6 weeks before the start of an interval throwing program. Although there is no well-defined postinjection recovery protocol, as a general rule rest was prescribed for the first 2 weeks, followed by a progressive stretching and strengthening program for the next month. Patients who were asymptomatic subjectively and clinically—negative moving valgus stress test, negative milking maneuver, no pain with valgus stress—were started on an interval throwing program.
Final follow-up involved a physical examination. Results were classified according to a modified version of the Conway Scale12,24-26: excellent (return to preinjury level of competition or performance), good (return to play at a lower level of competition or performance or, specifically for baseball players, ability to throw in daily batting practice), fair (able to play recreationally), and poor (unable to return to previous sport at any level).
By final follow-up, all patients had completed their postoperative rehabilitation protocol, and all had at least tried to return to their previous activities. No patients were lost to follow-up.
Results
Of the 44 baseball players, 6 were professional, 14 were in college, and 24 were in high school. There were 36 pitchers and 8 position players. Mean age was 17.3 years (range, 16-28 years). All patients were available for follow-up after injection (mean, 11 months). Fifteen of the 44 players had an excellent outcome (34%), 17 had a good outcome, 2 had a fair outcome, and 10 had a poor outcome. After injection, 4 (67%) of the 6 professional baseball players returned to professional play. Five (36%) of the 14 college players had an excellent outcome, and 4 (17%) of the 24 high school players had an excellent outcome. Of the 8 position players, 4 had an excellent outcome, 3 had a good outcome, and 1 had a poor outcome.
Before treatment, all patients had medial-sided elbow pain over the UCL inhibiting their ability to throw. Mean duration of symptoms before injection was 8.8 months (range, 1-36 months). There was no correlation between symptom duration and any outcome measure. On MRI, 29 patients showed partial tears: 22 proximally based and 7 distally based. The other 15 patients had diffuse signal without partial tear. All 7 patients with distally based partial tears and 3 of the patients with proximally based partial tears had a poor outcome. Overall, there were 6 excellent, 7 good, and 2 fair outcomes in the partial-tear group. In the patients with diffuse signal without partial tear, there were 9 excellent and 10 good outcomes.
Mean time from injection to return to throwing was 5 weeks, and mean time to return to competition was 12 weeks (range, 5-24 weeks). The 1 player who returned at 5 weeks was a professional relief pitcher whose team was in the playoffs. He has now pitched for an additional 2 baseball seasons without elbow difficulty.
There were no injection-related complications.
Discussion
To our knowledge, this is the first report documenting successful PRP treatment of UCL insufficiency. In this study, 73% of players who had failed a course of conservative treatment had good to excellent outcomes with PRP injection.
Data on successful nonoperative treatment of UCL injuries are limited. Rettig and colleagues1 treated 31 throwing athletes’ UCL injuries with a supervised rehabilitation program. Treatment included rest, use of anti-inflammatory medication, progressive strengthening, and an interval throwing program. Only 41% of the athletes returned to their previous level of play, and it took, on average, 24.5 weeks. There was no significant difference in age or in duration or acuity of symptoms between those who returned to play and those whose conservative treatment failed.
Surgical reconstruction of UCL injuries has been very successful, with upward of 90% of athletes returning to previous level of play.3,27The procedure, however, is not without associated complications, including retear of the ligament, stiffness, ulnar nerve injury, and fracture.27-29 In addition, even when successful, the procedure requires that athletes take 9 to 12 months to recover before returning to competition at their previous level.
Savoie and colleagues,30 in their recent study on UCL repairs, highlighted an important fact that is often overlooked when reviewing the literature on UCL tears. Most of the literature on these injuries focuses on college and professional baseball players in whom ligament damage is often extensive, precluding repair. In contrast to prior reports, Savoie and colleagues30 found excellent results in 93% of their young athletes who underwent UCL repair. It is possible that their results can be attributed to the fact that many of their athletes had tears isolated to one area of the ligament, as opposed to generalized ligament incompetence. Our improved results vis-à-vis other reports on conservative management may be attributable to the same phenomenon.
PRP has garnered much attention in the literature and media because of its potential to enhance healing of tendons and ligaments; in some cases, it can obviate the need for surgery. After failure of other nonoperative measures in 15 patients with elbow epicondylitis, Mishra and Pavelko8 treated each patient with a single PRP injection. They prepared the PRP using the GPS III system (Biomet). At final follow-up, 93% improvement was seen. Clearly, their experiment had design flaws: It was nonblinded, and 3 of the 5 patients in the control group treated with bupivacaine injection withdrew from the experiment. Despite its shortcomings, their study became the impetus for several other studies.
A larger, double-blinded, randomized controlled trial comparing PRP and cortisone injections for lateral epicondylitis in 100 patients is under way, and preliminary results have been published.9 A minimum of 6 months after injection, patients who received PRP showed more improvement in visual analog scale (VAS) pain scores and Disabilities of the Arm, Shoulder, and Hand (DASH) questionnaire scores. In another large, double-blinded, randomized controlled trial, patients with chronic lateral epicondylitis had significant improvements in VAS pain scores and DASH scores relative to patients injected with corticosteroids with a 2-year follow-up.31 Similarly, Thanasas and colleagues32 found significantly reduced VAS pain scores in patients injected with PRP versus autologous whole blood. Another study demonstrated improved tendon morphology using ultrasound imaging 6 months after PRP injection.33
Contrary to these positive results, Krogh and colleagues34 found that a single injection of PRP or glucocorticoid was not significantly superior to a saline injection for reducing pain and disability over a 3-month period in patients with lateral epicondylitis. Their study, however, had major flaws. Its original design called for a 12-month follow-up, but there was massive dropout in all 3 treatment arms, necessitating reporting of only 3-month data. In addition, 60% of the patients in the glucocorticoid group were not naïve to this treatment, so definitive conclusions about the efficacy of glucocorticoids could not be made.
In the present study, we successfully treated partial ligament tears with PRP injections. Sixty-seven percent of our baseball players returned to play at a mean of 4 months, much earlier than the 9 to 12 months typically required after ligament reconstruction. Many athletes, such as high school baseball players or aging veteran professional baseball players, do not have the luxury of 12 months for recovery. Therefore, this select group of patients clearly has a limited window of opportunity to return to play. In fact, these patients might be ideal candidates for PRP injections for UCL injuries. Return-to-play rates, however, differed significantly among professional players and nonprofessional players. The difference may be attributable to professional players’ conditioning, quality of physical therapy, extrinsic motivation, and other intangible factors. Four (67%) of our 6 professional baseball players returned to professional play after injection, whereas only 36% of college players and 17% of high school players had excellent outcomes.
Limitations
The present study had several weaknesses, several of which are inherent to PRP studies conducted so far. It was not a prospective, randomized controlled trial. It is important to note that PRP treatment in diseased tissue may have some drawbacks, as its success depends on the ability of healing tissue to use concentrated growth factors and cytokines to proliferate.35 Thus, a chronically injured ligament with depleted active cells may have a diminished response to PRP. Another limitation of this study is that we evaluated outcomes based on return to play using the Conway Scale, which is well reported but not validated. Despite the potential weaknesses of this outcome scale, it has become the benchmark for measuring the success of outcomes of UCL reconstruction. Furthermore, we did not measure patients’ satisfaction with the treatment. Players who could not return to their preinjury level of play may have considered the treatment a failure regardless of their ability to continue throwing. Last, MRI was not repeated to document ligament healing. We did not routinely perform a second MRI because we thought it would not affect treatment. Several series have found a high incidence of abnormal signal in baseball players’ UCLs. In this group of patients, the most important outcome is return to previous level of competition.
This study raised several questions. Is one PRP brand better than another? Should more than 1 injection be given? What is the ideal postinjection protocol? Clearly, larger, prospective, randomized controlled studies are needed to truly elucidate the potential role of PRP in the treatment algorithm for UCL injury. Nevertheless, in certain cases in which traditional conservative measures have failed and patients do not have the luxury of rehabilitating for 9 to 12 months after surgery, PRP may be a viable treatment option.
Conclusion
In this study, use of PRP in the treatment of UCL insufficiency produced outcomes much better than earlier reported outcomes of conservative treatment of these injuries. PRP injections may be particularly beneficial in young athletes who have sustained acute damage to an isolated part of the ligament and in athletes unwilling or unable to undergo the extended rehabilitation required after surgical reconstruction of the ligament.
For overhead athletes, elbow ulnar collateral ligament (UCL) insufficiency is a potential career-ending injury. Baseball players with UCL insufficiency typically complain of medial-sided elbow pain that affects their ability to throw. Loss of velocity, loss of control, difficulty warming up, and pain while throwing are all symptoms of UCL injury.
Classically, nonoperative treatment of UCL injuries involves activity modification, use of anti-inflammatory medication, and a structured physical therapy program. Asymptomatic players can return to throwing after a structured interval throwing program. Rettig and colleagues1 found a 42% rate of success in conservatively treating UCL injuries in throwing athletes. UCL reconstruction is reserved for players with complete tears of the UCL or with partial tears after failed conservative treatment. Several techniques have been used to reconstruct the ligament, but successful outcomes depend on a long rehabilitation process. According to most published series, 85% to 90% of athletes who had UCL reconstruction returned to their previous level of play, but it took, on average, 9 to 12 months.2,3 This prolonged recovery period is one reason that some older professional baseball players, as well as casual high school and college players, elect to forgo surgery.
Over the past few years, platelet-rich plasma (PRP) has garnered attention as a bridge between conservative treatment and surgery. PRP refers to a sample of autologous blood that contains a platelet concentration higher than baseline levels. This sample often has a 3 to 5 times increase in growth factor concentration.4-6 Initial studies focused on its ability to successfully treat lateral epicondylitis.7-9 More recent clinical work has shown that PRP can potentially enhance healing after anterior cruciate ligament reconstruction,10-14 rotator cuff repair,15-17 and subacromial decompression.11,18-23 If PRP could be used to successfully treat UCL insufficiency that is refractory to conservative treatment, then year-long recovery periods could be avoided. This could potentially prolong certain athletes’ careers or, at the very least, allow them to return to play much sooner. In the present case series, we hypothesized that PRP injections could be used to successfully treat partial UCL tears in high-level throwing athletes, obviating the need for surgery and its associated prolonged recovery period.
Materials and Methods
Institutional Review Board approval was obtained for this retrospective study of 44 baseball players treated with PRP injections for partial-thickness UCL tears.
Patients provided written informed consent. They were diagnosed with UCL insufficiency by physical examination, and findings were confirmed by magnetic resonance imaging (MRI). After diagnosis, all throwers underwent a trial of conservative treatment that included rest, activity modification, use of anti-inflammatory medication, and physical therapy followed by an attempt to return to throwing using an interval throwing program.
Study inclusion criteria were physical examinations and MRI results consistent with UCL insufficiency, and failure of the conservative treatment plan described.
Patients were injected using the Autologous Conditioned Plasma system (Arthrex). PRP solutions were prepared according to manufacturer guidelines. After the elbow was prepared sterilely, the UCL was injected at the location of the tear. Typically, 3 mL of PRP was injected into the elbow. Sixteen patients had 1 injection, 6 had 2, and 22 had 3. Repeat injections were considered for recalcitrant pain after 3 weeks.
After injection, patients used acetaminophen and ice for pain control. Anti-inflammatory medications were avoided for a minimum of 2 weeks after injection. Typical postinjection therapy protocol consisted of rest followed by progressive stretching and strengthening for about 4 to 6 weeks before the start of an interval throwing program. Although there is no well-defined postinjection recovery protocol, as a general rule rest was prescribed for the first 2 weeks, followed by a progressive stretching and strengthening program for the next month. Patients who were asymptomatic subjectively and clinically—negative moving valgus stress test, negative milking maneuver, no pain with valgus stress—were started on an interval throwing program.
Final follow-up involved a physical examination. Results were classified according to a modified version of the Conway Scale12,24-26: excellent (return to preinjury level of competition or performance), good (return to play at a lower level of competition or performance or, specifically for baseball players, ability to throw in daily batting practice), fair (able to play recreationally), and poor (unable to return to previous sport at any level).
By final follow-up, all patients had completed their postoperative rehabilitation protocol, and all had at least tried to return to their previous activities. No patients were lost to follow-up.
Results
Of the 44 baseball players, 6 were professional, 14 were in college, and 24 were in high school. There were 36 pitchers and 8 position players. Mean age was 17.3 years (range, 16-28 years). All patients were available for follow-up after injection (mean, 11 months). Fifteen of the 44 players had an excellent outcome (34%), 17 had a good outcome, 2 had a fair outcome, and 10 had a poor outcome. After injection, 4 (67%) of the 6 professional baseball players returned to professional play. Five (36%) of the 14 college players had an excellent outcome, and 4 (17%) of the 24 high school players had an excellent outcome. Of the 8 position players, 4 had an excellent outcome, 3 had a good outcome, and 1 had a poor outcome.
Before treatment, all patients had medial-sided elbow pain over the UCL inhibiting their ability to throw. Mean duration of symptoms before injection was 8.8 months (range, 1-36 months). There was no correlation between symptom duration and any outcome measure. On MRI, 29 patients showed partial tears: 22 proximally based and 7 distally based. The other 15 patients had diffuse signal without partial tear. All 7 patients with distally based partial tears and 3 of the patients with proximally based partial tears had a poor outcome. Overall, there were 6 excellent, 7 good, and 2 fair outcomes in the partial-tear group. In the patients with diffuse signal without partial tear, there were 9 excellent and 10 good outcomes.
Mean time from injection to return to throwing was 5 weeks, and mean time to return to competition was 12 weeks (range, 5-24 weeks). The 1 player who returned at 5 weeks was a professional relief pitcher whose team was in the playoffs. He has now pitched for an additional 2 baseball seasons without elbow difficulty.
There were no injection-related complications.
Discussion
To our knowledge, this is the first report documenting successful PRP treatment of UCL insufficiency. In this study, 73% of players who had failed a course of conservative treatment had good to excellent outcomes with PRP injection.
Data on successful nonoperative treatment of UCL injuries are limited. Rettig and colleagues1 treated 31 throwing athletes’ UCL injuries with a supervised rehabilitation program. Treatment included rest, use of anti-inflammatory medication, progressive strengthening, and an interval throwing program. Only 41% of the athletes returned to their previous level of play, and it took, on average, 24.5 weeks. There was no significant difference in age or in duration or acuity of symptoms between those who returned to play and those whose conservative treatment failed.
Surgical reconstruction of UCL injuries has been very successful, with upward of 90% of athletes returning to previous level of play.3,27The procedure, however, is not without associated complications, including retear of the ligament, stiffness, ulnar nerve injury, and fracture.27-29 In addition, even when successful, the procedure requires that athletes take 9 to 12 months to recover before returning to competition at their previous level.
Savoie and colleagues,30 in their recent study on UCL repairs, highlighted an important fact that is often overlooked when reviewing the literature on UCL tears. Most of the literature on these injuries focuses on college and professional baseball players in whom ligament damage is often extensive, precluding repair. In contrast to prior reports, Savoie and colleagues30 found excellent results in 93% of their young athletes who underwent UCL repair. It is possible that their results can be attributed to the fact that many of their athletes had tears isolated to one area of the ligament, as opposed to generalized ligament incompetence. Our improved results vis-à-vis other reports on conservative management may be attributable to the same phenomenon.
PRP has garnered much attention in the literature and media because of its potential to enhance healing of tendons and ligaments; in some cases, it can obviate the need for surgery. After failure of other nonoperative measures in 15 patients with elbow epicondylitis, Mishra and Pavelko8 treated each patient with a single PRP injection. They prepared the PRP using the GPS III system (Biomet). At final follow-up, 93% improvement was seen. Clearly, their experiment had design flaws: It was nonblinded, and 3 of the 5 patients in the control group treated with bupivacaine injection withdrew from the experiment. Despite its shortcomings, their study became the impetus for several other studies.
A larger, double-blinded, randomized controlled trial comparing PRP and cortisone injections for lateral epicondylitis in 100 patients is under way, and preliminary results have been published.9 A minimum of 6 months after injection, patients who received PRP showed more improvement in visual analog scale (VAS) pain scores and Disabilities of the Arm, Shoulder, and Hand (DASH) questionnaire scores. In another large, double-blinded, randomized controlled trial, patients with chronic lateral epicondylitis had significant improvements in VAS pain scores and DASH scores relative to patients injected with corticosteroids with a 2-year follow-up.31 Similarly, Thanasas and colleagues32 found significantly reduced VAS pain scores in patients injected with PRP versus autologous whole blood. Another study demonstrated improved tendon morphology using ultrasound imaging 6 months after PRP injection.33
Contrary to these positive results, Krogh and colleagues34 found that a single injection of PRP or glucocorticoid was not significantly superior to a saline injection for reducing pain and disability over a 3-month period in patients with lateral epicondylitis. Their study, however, had major flaws. Its original design called for a 12-month follow-up, but there was massive dropout in all 3 treatment arms, necessitating reporting of only 3-month data. In addition, 60% of the patients in the glucocorticoid group were not naïve to this treatment, so definitive conclusions about the efficacy of glucocorticoids could not be made.
In the present study, we successfully treated partial ligament tears with PRP injections. Sixty-seven percent of our baseball players returned to play at a mean of 4 months, much earlier than the 9 to 12 months typically required after ligament reconstruction. Many athletes, such as high school baseball players or aging veteran professional baseball players, do not have the luxury of 12 months for recovery. Therefore, this select group of patients clearly has a limited window of opportunity to return to play. In fact, these patients might be ideal candidates for PRP injections for UCL injuries. Return-to-play rates, however, differed significantly among professional players and nonprofessional players. The difference may be attributable to professional players’ conditioning, quality of physical therapy, extrinsic motivation, and other intangible factors. Four (67%) of our 6 professional baseball players returned to professional play after injection, whereas only 36% of college players and 17% of high school players had excellent outcomes.
Limitations
The present study had several weaknesses, several of which are inherent to PRP studies conducted so far. It was not a prospective, randomized controlled trial. It is important to note that PRP treatment in diseased tissue may have some drawbacks, as its success depends on the ability of healing tissue to use concentrated growth factors and cytokines to proliferate.35 Thus, a chronically injured ligament with depleted active cells may have a diminished response to PRP. Another limitation of this study is that we evaluated outcomes based on return to play using the Conway Scale, which is well reported but not validated. Despite the potential weaknesses of this outcome scale, it has become the benchmark for measuring the success of outcomes of UCL reconstruction. Furthermore, we did not measure patients’ satisfaction with the treatment. Players who could not return to their preinjury level of play may have considered the treatment a failure regardless of their ability to continue throwing. Last, MRI was not repeated to document ligament healing. We did not routinely perform a second MRI because we thought it would not affect treatment. Several series have found a high incidence of abnormal signal in baseball players’ UCLs. In this group of patients, the most important outcome is return to previous level of competition.
This study raised several questions. Is one PRP brand better than another? Should more than 1 injection be given? What is the ideal postinjection protocol? Clearly, larger, prospective, randomized controlled studies are needed to truly elucidate the potential role of PRP in the treatment algorithm for UCL injury. Nevertheless, in certain cases in which traditional conservative measures have failed and patients do not have the luxury of rehabilitating for 9 to 12 months after surgery, PRP may be a viable treatment option.
Conclusion
In this study, use of PRP in the treatment of UCL insufficiency produced outcomes much better than earlier reported outcomes of conservative treatment of these injuries. PRP injections may be particularly beneficial in young athletes who have sustained acute damage to an isolated part of the ligament and in athletes unwilling or unable to undergo the extended rehabilitation required after surgical reconstruction of the ligament.
1. Rettig AC, Sherrill C, Snead DS, Mendler JC, Mieling P. Nonoperative treatment of ulnar collateral ligament injuries in throwing athletes. Am J Sports Med. 2001;29(1):15-17.
2. Eygendaal D, Rahussen FT, Diercks RL. Biomechanics of the elbow joint in tennis players and relation to pathology. Br J Sports Med. 2007;41(11):820-823.
3. Bowers AL, Dines JS, Dines DM, Altchek DW. Elbow medial ulnar collateral ligament reconstruction: clinical relevance and the docking technique. J Shoulder Elbow Surg. 2010;19(2):110-117.
5. Kibler WB. Biomechanical analysis of the shoulder during tennis activities. Clin Sports Med. 1995;14(1):79-85.
5. Marx RE. Platelet-rich plasma: evidence to support its use. J Oral Maxillofac Surg. 2004;62(4):489-496.
6. Marx RE. Platelet-rich plasma (PRP): what is PRP and what is not PRP? Implant Dent. 2001;10(4):225-228.
7. Elliott B, Fleisig G, Nicholls R, Escamilia R. Technique effects on upper limb loading in the tennis serve. J Sci Med Sport. 2003;6(1):76-87.
8. Mishra A, Pavelko T. Treatment of chronic elbow tendinosis with buffered platelet-rich plasma. Am J Sports Med. 2006;34(11):1774-1778.
9. Mishra A, Woodall J Jr, Vieira A. Treatment of tendon and muscle using platelet-rich plasma. Clin Sports Med. 2009;28(1):113-125.
10. Kovacs MS. Applied physiology of tennis performance. Br J Sports Med. 2006;40(5):381-386.
11. Xie X, Wu H, Zhao S, Xie G, Huangfu X, Zhao J. The effect of platelet-rich plasma on patterns of gene expression in a dog model of anterior cruciate ligament reconstruction. J Surg Res. 2013;180(1):80-88.
12. Pluim BM, Staal JB, Windler GE, Jayanthi N. Tennis injuries: occurrence, aetiology, and prevention. Br J Sports Med. 2006;40(5):415-423.
13. Xie X, Zhao S, Wu H, et al. Platelet-rich plasma enhances autograft revascularization and reinnervation in a dog model of anterior cruciate ligament reconstruction. J Surg Res. 2013;183(1):214-222.
14. Lopez-Vidriero E, Goulding KA, Simon DA, Sanchez M, Johnson DH. The use of platelet-rich plasma in arthroscopy and sports medicine: optimizing the healing environment. Arthroscopy. 2010;26(2):269-278.
15. Jo CH, Shin JS, Shin WH, Lee SY, Yoon KS, Shin S. Platelet-rich plasma for arthroscopic repair of medium to large rotator cuff tears: a randomized controlled trial. Am J Sports Med. 2015;43(9):2102-2110.
16. Jo CH, Shin JS, Lee YG, et al. Platelet-rich plasma for arthroscopic repair of large to massive rotator cuff tears: a randomized, single-blinded, parallel-group trial. Am J Sports Med. 2013;41(10):2240-2248.
17. Randelli P, Arrigoni P, Ragone V, Aliprandi A, Cabitza P. Platelet-rich plasma in arthroscopic rotator cuff repair: a prospective RCT study, 2-year follow-up. J Shoulder Elbow Surg. 2011;20(4):518-528.
18. Randelli P, Arrigoni P, Ragone V, Aliprandi A, Cabitza P. Platelet rich plasma in arthroscopic rotator cuff repair: a prospective RCT study, 2-year follow-up. J Shoulder Elbow Surg. 2011;20(4):518-528.
19. Barber FA, Hrnack SA, Snyder SJ, Hapa O. Rotator cuff repair healing influenced by platelet-rich plasma construct augmentation. Arthroscopy. 2011;27(8):1029-1035.
20. Jo CH, Kim JE, Yoon KS, et al. Does platelet-rich plasma accelerate recovery after rotator cuff repair? A prospective cohort study. Am J Sports Med. 2011;39(10):2082-2090.
21. Jo CH, Kim JE, Yoon KS, Shin S. Platelet-rich plasma stimulates cell proliferation and enhances matrix gene expression and synthesis in tenocytes from human rotator cuff tendons with degenerative tears. Am J Sports Med. 2012;40(5):1035-1045.
22. Chahal J, Van Thiel GS, Mall N, et al. The role of platelet-rich plasma in arthroscopic rotator cuff repair: a systematic review with quantitative synthesis. Arthroscopy. 2012;28(11):1718-1727.
23. Mei-Dan O, Carmont MR. The role of platelet-rich plasma in rotator cuff repair. Sports Med Arthrosc Rev. 2011;19(3):244-250.
24. Dines JS, ElAttrache NS, Conway JE, Smith W, Ahmad CS. Clinical outcomes of the DANE TJ technique to treat ulnar collateral ligament insufficiency of the elbow. Am J Sports Med. 2007;35(12):2039-2044.
25. Hutchinson MR, Laprade RF, Burnett QM 2nd, Moss R, Terpstra J. Injury surveillance at the USTA boys’ tennis championships: a 6-yr study. Med Sci Sports Exerc. 1995;27(6):826-830.
26. Winge S, Jørgensen U, Nielsen A. Epidemiology of injuries in Danish championship tennis. Int J Sports Med. 1989;10(5):368-371.
27. Safran MR, Hutchinson MR, Moss R, Albrandt J. A comparison of injuries in elite boys and girls tennis players. Paper presented at: 9th Annual Meeting of the Society of Tennis Medicine and Science; March 1999; Indian Wells, CA.
28. Cain EL, Andrews JR, Dugas JR, et al. Outcome of ulnar collateral ligament reconstruction of the elbow in 1281 athletes: results in 743 athletes with minimum 2-year follow-up. Am J Sports Med. 2010;38(12):2426-2434.
29. Dines JS, Yocum LA, Frank JB, ElAttrache NS, Gambardella RA, Jobe FW. Revision surgery for failed elbow medial collateral ligament reconstruction. Am J Sports Med. 2008;36(6):1061-1065.
30. Savoie FH, Trenhaile SW, Roberts J, Field LD, Ramsey JR. Primary repair of ulnar collateral ligament injuries of the elbow in young athletes: a case series of injuries to the proximal and distal ends of the ligament. Am J Sports Med. 2008;36(6):1066-1072.
31. Gosens T, Peerbooms JC, van Laar W, Oudsten den BL. Ongoing positive effect of platelet-rich plasma versus corticosteroid injection in lateral epicondylitis: a double-blind randomized controlled trial with 2-year follow-up. Am J Sports Med. 2011;39(6):1200-1208.
32. Thanasas C, Papadimitriou G, Charalambidis C, Paraskevopoulos I, Papanikolaou A. Platelet-rich plasma versus autologous whole blood for the treatment of chronic lateral elbow epicondylitis: a randomized controlled clinical trial. Am J Sports Med. 2011;39(10):2130-2134.
33. Chaudhury S, La Lama de M, Adler RS, et al. Platelet-rich plasma for the treatment of lateral epicondylitis: sonographic assessment of tendon morphology and vascularity (pilot study). Skeletal Radiol. 2013;42(1):91-97.
34. Krogh TP, Fredberg U, Stengaard-Pedersen K, Christensen R, Jensen P, Ellingsen T. Treatment of lateral epicondylitis with platelet-rich plasma, glucocorticoid, or saline: a randomized, double-blind, placebo-controlled trial. Am J Sports Med. 2013;41(3):625-635.
35. Anz AW, Hackel JG, Nilssen EC, Andrews JR. Application of biologics in the treatment of the rotator cuff, meniscus, cartilage, and osteoarthritis. J Am Acad Orthop Surg. 2014;22(2):68-79.
1. Rettig AC, Sherrill C, Snead DS, Mendler JC, Mieling P. Nonoperative treatment of ulnar collateral ligament injuries in throwing athletes. Am J Sports Med. 2001;29(1):15-17.
2. Eygendaal D, Rahussen FT, Diercks RL. Biomechanics of the elbow joint in tennis players and relation to pathology. Br J Sports Med. 2007;41(11):820-823.
3. Bowers AL, Dines JS, Dines DM, Altchek DW. Elbow medial ulnar collateral ligament reconstruction: clinical relevance and the docking technique. J Shoulder Elbow Surg. 2010;19(2):110-117.
5. Kibler WB. Biomechanical analysis of the shoulder during tennis activities. Clin Sports Med. 1995;14(1):79-85.
5. Marx RE. Platelet-rich plasma: evidence to support its use. J Oral Maxillofac Surg. 2004;62(4):489-496.
6. Marx RE. Platelet-rich plasma (PRP): what is PRP and what is not PRP? Implant Dent. 2001;10(4):225-228.
7. Elliott B, Fleisig G, Nicholls R, Escamilia R. Technique effects on upper limb loading in the tennis serve. J Sci Med Sport. 2003;6(1):76-87.
8. Mishra A, Pavelko T. Treatment of chronic elbow tendinosis with buffered platelet-rich plasma. Am J Sports Med. 2006;34(11):1774-1778.
9. Mishra A, Woodall J Jr, Vieira A. Treatment of tendon and muscle using platelet-rich plasma. Clin Sports Med. 2009;28(1):113-125.
10. Kovacs MS. Applied physiology of tennis performance. Br J Sports Med. 2006;40(5):381-386.
11. Xie X, Wu H, Zhao S, Xie G, Huangfu X, Zhao J. The effect of platelet-rich plasma on patterns of gene expression in a dog model of anterior cruciate ligament reconstruction. J Surg Res. 2013;180(1):80-88.
12. Pluim BM, Staal JB, Windler GE, Jayanthi N. Tennis injuries: occurrence, aetiology, and prevention. Br J Sports Med. 2006;40(5):415-423.
13. Xie X, Zhao S, Wu H, et al. Platelet-rich plasma enhances autograft revascularization and reinnervation in a dog model of anterior cruciate ligament reconstruction. J Surg Res. 2013;183(1):214-222.
14. Lopez-Vidriero E, Goulding KA, Simon DA, Sanchez M, Johnson DH. The use of platelet-rich plasma in arthroscopy and sports medicine: optimizing the healing environment. Arthroscopy. 2010;26(2):269-278.
15. Jo CH, Shin JS, Shin WH, Lee SY, Yoon KS, Shin S. Platelet-rich plasma for arthroscopic repair of medium to large rotator cuff tears: a randomized controlled trial. Am J Sports Med. 2015;43(9):2102-2110.
16. Jo CH, Shin JS, Lee YG, et al. Platelet-rich plasma for arthroscopic repair of large to massive rotator cuff tears: a randomized, single-blinded, parallel-group trial. Am J Sports Med. 2013;41(10):2240-2248.
17. Randelli P, Arrigoni P, Ragone V, Aliprandi A, Cabitza P. Platelet-rich plasma in arthroscopic rotator cuff repair: a prospective RCT study, 2-year follow-up. J Shoulder Elbow Surg. 2011;20(4):518-528.
18. Randelli P, Arrigoni P, Ragone V, Aliprandi A, Cabitza P. Platelet rich plasma in arthroscopic rotator cuff repair: a prospective RCT study, 2-year follow-up. J Shoulder Elbow Surg. 2011;20(4):518-528.
19. Barber FA, Hrnack SA, Snyder SJ, Hapa O. Rotator cuff repair healing influenced by platelet-rich plasma construct augmentation. Arthroscopy. 2011;27(8):1029-1035.
20. Jo CH, Kim JE, Yoon KS, et al. Does platelet-rich plasma accelerate recovery after rotator cuff repair? A prospective cohort study. Am J Sports Med. 2011;39(10):2082-2090.
21. Jo CH, Kim JE, Yoon KS, Shin S. Platelet-rich plasma stimulates cell proliferation and enhances matrix gene expression and synthesis in tenocytes from human rotator cuff tendons with degenerative tears. Am J Sports Med. 2012;40(5):1035-1045.
22. Chahal J, Van Thiel GS, Mall N, et al. The role of platelet-rich plasma in arthroscopic rotator cuff repair: a systematic review with quantitative synthesis. Arthroscopy. 2012;28(11):1718-1727.
23. Mei-Dan O, Carmont MR. The role of platelet-rich plasma in rotator cuff repair. Sports Med Arthrosc Rev. 2011;19(3):244-250.
24. Dines JS, ElAttrache NS, Conway JE, Smith W, Ahmad CS. Clinical outcomes of the DANE TJ technique to treat ulnar collateral ligament insufficiency of the elbow. Am J Sports Med. 2007;35(12):2039-2044.
25. Hutchinson MR, Laprade RF, Burnett QM 2nd, Moss R, Terpstra J. Injury surveillance at the USTA boys’ tennis championships: a 6-yr study. Med Sci Sports Exerc. 1995;27(6):826-830.
26. Winge S, Jørgensen U, Nielsen A. Epidemiology of injuries in Danish championship tennis. Int J Sports Med. 1989;10(5):368-371.
27. Safran MR, Hutchinson MR, Moss R, Albrandt J. A comparison of injuries in elite boys and girls tennis players. Paper presented at: 9th Annual Meeting of the Society of Tennis Medicine and Science; March 1999; Indian Wells, CA.
28. Cain EL, Andrews JR, Dugas JR, et al. Outcome of ulnar collateral ligament reconstruction of the elbow in 1281 athletes: results in 743 athletes with minimum 2-year follow-up. Am J Sports Med. 2010;38(12):2426-2434.
29. Dines JS, Yocum LA, Frank JB, ElAttrache NS, Gambardella RA, Jobe FW. Revision surgery for failed elbow medial collateral ligament reconstruction. Am J Sports Med. 2008;36(6):1061-1065.
30. Savoie FH, Trenhaile SW, Roberts J, Field LD, Ramsey JR. Primary repair of ulnar collateral ligament injuries of the elbow in young athletes: a case series of injuries to the proximal and distal ends of the ligament. Am J Sports Med. 2008;36(6):1066-1072.
31. Gosens T, Peerbooms JC, van Laar W, Oudsten den BL. Ongoing positive effect of platelet-rich plasma versus corticosteroid injection in lateral epicondylitis: a double-blind randomized controlled trial with 2-year follow-up. Am J Sports Med. 2011;39(6):1200-1208.
32. Thanasas C, Papadimitriou G, Charalambidis C, Paraskevopoulos I, Papanikolaou A. Platelet-rich plasma versus autologous whole blood for the treatment of chronic lateral elbow epicondylitis: a randomized controlled clinical trial. Am J Sports Med. 2011;39(10):2130-2134.
33. Chaudhury S, La Lama de M, Adler RS, et al. Platelet-rich plasma for the treatment of lateral epicondylitis: sonographic assessment of tendon morphology and vascularity (pilot study). Skeletal Radiol. 2013;42(1):91-97.
34. Krogh TP, Fredberg U, Stengaard-Pedersen K, Christensen R, Jensen P, Ellingsen T. Treatment of lateral epicondylitis with platelet-rich plasma, glucocorticoid, or saline: a randomized, double-blind, placebo-controlled trial. Am J Sports Med. 2013;41(3):625-635.
35. Anz AW, Hackel JG, Nilssen EC, Andrews JR. Application of biologics in the treatment of the rotator cuff, meniscus, cartilage, and osteoarthritis. J Am Acad Orthop Surg. 2014;22(2):68-79.