User login
Inappropriate Hospital Prescribing
Medications can be considered inappropriate when their risk outweighs their benefit. The Beers list1 identifies medications that should be avoided in persons 65 years or older because they are ineffective or pose an unnecessarily high risk or because a safer alternative is available. Initially developed in 1991, the list has gained wide acceptance and has been updated twice.2, 3 In July 1999 it was adopted by the Centers for Medicare & Medicaid Services (CMS) for nursing home regulation, and in 2006 the National Committee on Quality Assurance adopted a modified version as a Health Plan Employer Data and Information Set (HEDIS) measure of quality of care for older Americans.4
A number of studies have demonstrated that inappropriate prescribing is common in the ambulatory setting,57 in nursing homes,8, 9 and in emergency departments10, 11 and that exposure to inappropriate medications is associated with increased risk of adverse drug reactions12 and hospitalization.13, 14 Initial studies of hospitalized patients1517 suggest that potentially inappropriate prescribing is also common among elderly inpatients and that reducing the misuse of psychotropic medications can prevent falls.18 We report on the incidence of and risk factors associated with potentially inappropriate prescribing in a large sample of hospitalized elders.
METHODS
Patients
We conducted a retrospective cohort study using data from 384 hospitals participating in Perspective (Premier, Inc., Charlotte, NC), a database developed for measuring quality and health care utilization. Participating hospitals represent all regions of the United States and are primarily small‐ to medium‐sized nonteaching hospitals most of which are in urban areas. Premier collects data elements from participating hospitals via a custom data extract from hospitals' decision support system. Hospitals aggregate the data elements into their decision support systems from multiple information technology systems including billing, medical records, pharmacy, and laboratory systems. In addition to the information contained in the standard hospital discharge file, Perspective includes a date‐stamped log of all billed items, including medications with dose and quantity, for individual patients.
We included patients at least 65 years old admitted between September 1, 2002, and June 30, 2005, with a principal diagnosis of acute myocardial infarction, chronic obstructive pulmonary disease, chest pain, community‐acquired pneumonia, congestive heart failure, ischemic stroke, or urinary tract infection. International Classification of Diseases, Ninth Revision (ICD‐9‐CM) codes were used to identify diagnoses. Patients cared for by an attending physician with a surgical specialty were excluded. The study protocol was approved by the institutional review board of Baystate Medical Center.
Data Elements
For each patient, Perspective contains fields for age, sex, race, marital status, insurance status, principal diagnosis, comorbidities, and specialty of the attending physician. Comorbidities were identified from ICD‐9‐CM secondary diagnosis codes and APR‐DRGs using Healthcare Cost and Utilization Project Comorbidity Software, version 3.1, based on the work of Elixhauser.19 Because almost all patients had Medicare coverage, plans were classified according to managed care status. Finally, for each patient we identified all medications administered, as well as discharge status, readmission rate, total costs, and length of stay. Hospitals were categorized by region (Northeast, South, Midwest, or West), bed size, setting (urban or rural), teaching status, and whether there were geriatricians.
Potentially Inappropriate Prescribing
Using the 2002 updated Beers criteria3 for potentially inappropriate medication (PIM) use in older adults, we identified the total number of PIMs administered to each patient during his or her hospital stay. We classified each PIM as either high or low severity based on the expert consensus expressed in the 1997 update of the Beers criteria.2 The list contains 48 PIMs and an additional 20 that should be avoided in patients with certain conditions. We did not include the second category of PIMs because we did not necessarily have sufficient patient information to make this determination. In addition, some of the standard PIMs, such as laxatives, although inappropriate for chronic outpatient use, could be appropriate in the hospital setting and were excluded from this analysis. Finally, several medications were considered inappropriate only above a given threshold (eg, lorazepam >3.0 mg/day) or for patients without a specific diagnosis (eg, digoxin >0.125 mg/day for patients without atrial fibrillation). We grouped PIMs that had similar side effects into 4 categories: sedatives, anticholinergics, causing orthostasis, or causing bleeding (Fig. 1).

Statistical Analysis
Summary statistics at the patient, physician, and hospital levels were constructed using frequencies and proportions for categorical data and means, standard deviations, medians, interquartile ranges, and box plots for continuous‐scale variables. Patients were identified as receiving a PIM if the drug was administered (above threshold dose where applicable) on at least 1 hospital day. We examined the association of each patient characteristic with use of any PIM, any high‐severity‐rated PIM, and each side effect category using chi‐square statistics. Kruskal‐Wallis analysis of variance was used to examine variation in hospital use rates by each hospital characteristic, and physician use rates for high‐severity PIMs by attending specialty. To examine whether it was feasible to avoid PIMs altogether, we compared individual hospitals as well as individual prescribers within their specialty, limiting the comparison to hospitals that contributed at least 100 patients and to physicians with at least 50 patients.
We developed a multivariable model for any high‐severity medication (HS‐PIM) use that included all patient, physician, and hospital characteristics except length of stay, mortality, cost, discharge status, and readmission rate. A generalized estimating equation model (SAS PROC GENMOD) with a logit link and a subcluster correlation structure was used to account for clustering at the hospital, physician, and diagnosis levels, adjusting for the clustering of primary diagnosis within physician level, nested within hospital level. Effects with P < .10 were retained in the model, and interaction effects were also evaluated for significance. Model fit was assessed using deviance and Pearson chi‐square statistics. All analyses were performed with SAS statistical software, version 9.1 (SAS Institute, Cary, NC).
RESULTS
We identified 519,853 patients at least 65 years old during the study period; 564 were excluded because of missing data for key variables or unclear principal diagnosis. An additional 25,318 were excluded because they were cared for by an attending with a surgical specialty. A total of 493,971 patients were included in the study (Table 1). Mean age was 78 years, and 24% of patients were 85 years or older. Forty‐three percent were male, 71% were white, and 39% were currently married. The most common principal diagnoses were community‐acquired pneumonia, congestive heart failure, and acute myocardial infarction. The most common comorbidities were hypertension, diabetes, and chronic pulmonary disease. Medicare was the primary payer for 91% of subjects, and 13% were in managed care plans. Most patients were cared for by internists (49%), family practitioners (18%), or cardiologists (17%). Only 1% of patients had a geriatrician as attending.
Characteristic | n (%) |
---|---|
| |
Age group | |
6574 years | 168,527 (34%) |
7584 years | 206,407 (42%) |
85+ years | 119,037 (24%) |
Sex | |
Male | 212,358 (43%) |
Female | 281,613 (57%) |
Race | |
White | 351,331 (71%) |
Black | 52,429 (11%) |
Hispanic | 18,057 (4%) |
American Indian | 1876 (0%) |
Asian/Pacific Islander | 5926 (1%) |
Other | 64,352 (13%) |
Marital status | |
Married/partner | 194,496 (39%) |
Widowed | 155,273 (31%) |
Single/separated/divorced | 75,964 (15%) |
Other | 68,238 (14%) |
Primary diagnosis | |
Pneumonia | 122,732 (25%) |
Heart failure | 109,071 (22%) |
Acute MI | 70,581 (14%) |
Ischemic stroke | 57,204 (12%) |
Chest pain | 50,404 (10%) |
COPD | 44,582 (9%) |
Urinary tract infection | 39,397 (8%) |
Comorbidities | |
Hypertension | 310,163 (63%) |
Diabetes | 151,755 (31%) |
Chronic pulmonary disease | 134,900 (27%) |
Fluid and electrolyte disorders | 128,703 (26%) |
Deficiency anemias | 92,668 (19%) |
Congestive heart failure | 69,201 (14%) |
Hypothyroidism | 68,711 (14%) |
Peripheral vascular disease | 47,244 (10%) |
Depression | 41,507 (8%) |
Other neurological disorders | 40,200 (8%) |
Renal failure | 38,134 (8%) |
Obesity | 25,143 (5%) |
Payer type | |
Not Managed care | 431,583 (87%) |
Managed care | 62,388 (13%) |
Attending physician specialty | |
Internal medicine (internist) | 241,982 (49%) |
Family/general medicine | 90,827 (18%) |
Cardiology | 83,317 (17%) |
Pulmonology | 21,163 (4%) |
Hospitalist | 14,924 (3%) |
Nephrology | 8257 (2%) |
Neurology | 5800 (1%) |
Geriatrics | 3099 (1%) |
Other* | 24,602 (5%) |
Mortality | |
Expired | 28,321 (6%) |
Alive | 465,650 (94%) |
Discharge status, n (% of survivors) | |
Home | 323,629 (66%) |
Nursing care | 119,468 (24%) |
Transfer/short‐term hospital | 13,531 (3%) |
Hospice | 9022 (2%) |
14‐Day readmission, n (% of survivors) | |
Yes | 35,309 (8%) |
No | 430,334 (92%) |
Length of stay (days), median (IQR) | 4 (2, 7) |
Total cost (dollars) | $5513 ($3366, $9902) |
Just under half of all patients (49%) received at least 1 PIM, and 6% received 3 or more (Table 2). Thirty‐eight percent received at least 1 drug with a high severity rating (HS‐PIM). The most common PIMs were promethazine, diphenhydramine, propoxyphene, clonidine, amiodarone, and lorazepam (>3 mg/day).
Patients, n (%) | |
---|---|
Number of PIMs | |
0 | 254,200 (51%) |
1 | 146,028 (30%) |
2 | 61,445 (12%) |
3 | 22,128 (4%) |
4+ | 10,170 (2%) |
Number of high‐severity‐rated PIMs | |
0 | 304,523 (62%) |
1 | 129,588 (26%) |
2 | 43,739 (9%) |
3 | 12,213 (2%) |
4+ | 3908 (1%) |
Use of any PIM by side effect class | |
Sedatives | 156,384 (32%) |
Anticholinergic effects | 109,293 (22%) |
Causing orthostasis | 43,805 (9%) |
Causing bleeding | 14,744 (3%) |
Most commonly prescribed | |
Promethazine | 49,888 (10%) |
Diphenhydramine | 45,458 (9%) |
Propoxyphene | 41,786 (8%) |
Clonidine | 34,765 (7%) |
Amiodarone | 34,318 (7%) |
Lorazepam (>3 mg/day) | 25,147 (5%) |
Patient, Physician, and Hospital Factors Associated with PIMs
Patient, physician, and hospital characteristics were all associated with use of PIMs (Table 3). In univariate analyses, older patients were less likely to receive any class of PIM, and this difference was accentuated for HS‐PIMs. Women, American Indians, married people, and those not in managed care plans were slightly more likely to receive PIMs, whereas patients admitted with acute myocardial infarction or congestive heart failure were even more likely to receive PIMs (P < .0001 for all comparisons).
Patient characteristic | Any PIM n (row %) | Any high‐severity PIM n (row %) | Sedatives n (row %) | Anticholinergic effects n (row %) | Causing orthostasis n (row %) | Causing bleeding n (row %) |
---|---|---|---|---|---|---|
| ||||||
Overall | 239,771 (49%) | 189,448 (38%) | 156,384 (32%) | 109,293 (22%) | 43,805 (9%) | 14,744 (3%) |
Age group | ||||||
6574 years | 89,168 (53%) | 72,573 (43%) | 61,399 (36%) | 44,792 (27%) | 15,799 (9%) | 6655 (4%) |
7584 years | 100,787 (49%) | 79,595 (39%) | 65,034 (32%) | 45,121 (22%) | 18,519 (9%) | 5727 (3%) |
85+ years | 49,816 (42%) | 37,280 (31%) | 29,951 (25%) | 19,380 (16%) | 9487 (8%) | 2362 (2%) |
Sex | ||||||
Male | 100,824 (47%) | 79,535 (37%) | 63,591 (30%) | 42,754 (20%) | 17,885 (8%) | 5771 (3%) |
Female | 138,947 (49%) | 109,913 (39%) | 92,793 (33%) | 66,539 (24%) | 25,920 (9%) | 8973 (3%) |
Race | ||||||
White | 173,481 (49%) | 139,941 (40%) | 112,556 (32%) | 81,097 (23%) | 27,555 (8%) | 10,590 (3%) |
Black | 26,793 (51%) | 18,655 (36%) | 18,720 (36%) | 11,263 (21%) | 8925 (17%) | 1536 (3%) |
Hispanic | 8509 (47%) | 6370 (35%) | 5549 (31%) | 3505 (19%) | 2047 (11%) | 648 (4%) |
American Indian | 1091 (58%) | 849 (45%) | 818 (44%) | 563 (30%) | 190 (10%) | 76 (4%) |
Asian/Pacific Islander | 2386 (40%) | 1896 (32%) | 1420 (24%) | 1023 (17%) | 519 (9%) | 127 (2%) |
Other | 27,511 (43%) | 21,737 (34%) | 17,321 (27%) | 11,842 (18%) | 4569 (7%) | 1767 (3%) |
Marital status | ||||||
Married/partner | 96,874 (50%) | 77,803 (40%) | 63,303 (33%) | 45,042 (23%) | 16,765 (9%) | 5969 (3%) |
Widowed | 74,622 (48%) | 58,012 (37%) | 48,367 (31%) | 33,516 (22%) | 13,865 (9%) | 4354 (3%) |
Single/separated/divorced | 36,583 (48%) | 28,799 (38%) | 24,251 (32%) | 16,115 (21%) | 7229 (10%) | 2399 (3%) |
Other | 31,692 (46%) | 24,834 (36%) | 20,463 (30%) | 14,620 (21%) | 5946 (9%) | 2022 (3%) |
Primary diagnosis | ||||||
Pneumonia | 56,845 (46%) | 46,271 (38%) | 35,353 (29%) | 25,484 (21%) | 9184 (7%) | 4155 (3%) |
Heart failure | 56,460 (52%) | 42,231 (39%) | 34,340 (31%) | 22,093 (20%) | 10,117 (9%) | 1945 (2%) |
Acute MI | 43,046 (61%) | 37,849 (54%) | 32,560 (46%) | 25,568 (36%) | 4738 (7%) | 2549 (4%) |
Ischemic stroke | 25,763 (45%) | 17,613 (31%) | 18,500 (32%) | 8742 (15%) | 9644 (17%) | 1384 (2%) |
Chest pain | 20,655 (41%) | 16,363 (32%) | 13,536 (27%) | 10,520 (21%) | 3474 (7%) | 2027 (4%) |
COPD | 18,876 (42%) | 14,626 (33%) | 12,087 (27%) | 8096 (18%) | 3209 (7%) | 1139 (3%) |
Urinary tract infection | 18,126 (46%) | 14,495 (37%) | 10,008 (25%) | 8790 (22%) | 3439 (9%) | 1545 (4%) |
Payer type | ||||||
Nonmanaged care | 212,150 (49%) | 168,013 (39%) | 138,679 (32%) | 97,776 (23%) | 38,341 (9%) | 12,868 (3%) |
Managed care | 27,621 (44%) | 21,435 (34%) | 17,705 (28%) | 11,517 (18%) | 5464 (9%) | 1876 (3%) |
Attending physician specialty* | ||||||
Internal medicine (internist%) | 112,664 (47%) | 86,907 (36%) | 71,382 (30%) | 48,746 (20%) | 23,221 (10%) | 7086 (3%) |
Family/general medicine | 41,303 (45%) | 32,338 (36%) | 25,653 (28%) | 18,274 (20%) | 7660 (8%) | 2852 (3%) |
Cardiology | 48,485 (58%) | 40,752 (49%) | 34,859 (42%) | 25,792 (31%) | 5455 (7%) | 2542 (3%) |
Pulmonology | 10,231 (48%) | 8105 (38%) | 6746 (32%) | 4064 (19%) | 1739 (8%) | 574 (3%) |
Hospitalist | 7003 (47%) | 5443 (36%) | 4447 (30%) | 3179 (21%) | 1471 (10%) | 463 (3%) |
Nephrology | 4508 (55%) | 3388 (41%) | 3132 (38%) | 2054 (25%) | 1326 (16%) | 198 (2%) |
Neurology | 2420 (42%) | 1789 (31%) | 1625 (28%) | 851 (15%) | 699 (12%) | 174 (3%) |
Geriatrics | 1020 (33%) | 785 (25%) | 596 (19%) | 404 (13%) | 196 (6%) | 41 (1%) |
The HS‐PIM prescribing varied substantially by attending specialty (Fig. 2). Internists, family practitioners, and hospitalists all had similar median rates (33%), cardiologists had a higher median rate (48%), and geriatricians had a lower rate (24%). The most common PIM also differed by specialty: whereas promethazine was the most commonly prescribed drug across most specialties, nephrologists and neurologists used clonidine, pulmonologists used lorazepam, and cardiologists used diphenhydramine most often. Among the 8% of physicians who saw at least 50 patients, there was also great variation in each specialty (Fig. 2). Among internists and cardiologists who saw at least 50 patients, the high‐severity PIM usage rate ranged from 0% to more than 90%.
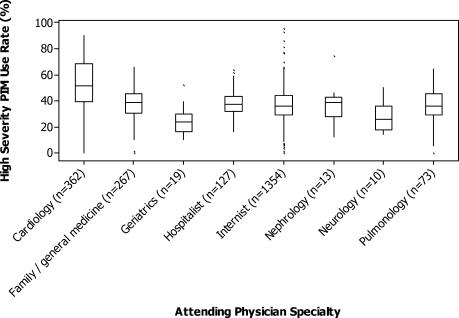
There was substantial variation in PIM usage among hospitals, most notably by region. The mean proportion of patients receiving PIMs ranged from 34% at hospitals in the Northeast to 55% at hospitals in the South (Table 4). Smaller hospitals and those in urban settings had slightly lower rates, as did those that had geriatricians on staff. The teaching status of the hospital had little effect. Variation at the individual hospital level was extreme (Fig. 3). Although half of all hospitals had rates between 43% and 58%, in 7 hospitals with more than 300 encounters each, PIMs were never prescribed for geriatric patients.
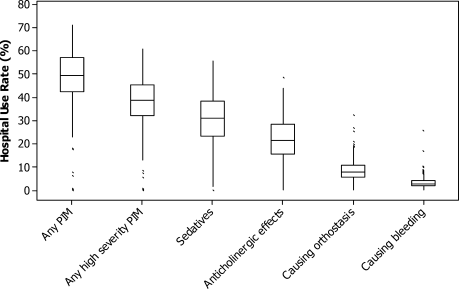
Hospitals Total = 384 n (%) | Patients N = 49,3971 n (%) | Any PIM Mean = 48.2 Mean (SD) | Any high‐severity PIM Mean = 38.7 Mean (SD) | Sedatives Mean = 30.2 Mean (SD) | Anticholinergic effects Mean = 21.5 Mean (SD) | Causing orthostasis Mean = 8.5 Mean (SD) | Causing bleeding Mean = 3.1 Mean (SD) | |
---|---|---|---|---|---|---|---|---|
| ||||||||
Hospital region | *** | *** | *** | *** | *** | ** | ||
Midwest | 76 (20%) | 95,791 (19%) | 38.8 (19.7) | 30.0 (16.4) | 24.3 (13.8) | 15.1 (9.9) | 6.9 (6.3) | 3.1 (2.3) |
Northeast | 47 (12%) | 79,138 (16%) | 34.1 (12.6) | 26.2 (11.2) | 19.0 (9.2) | 13.5 (8.1) | 4.9 (2.3) | 2.1 (1.6) |
South | 199 (52%) | 260,870 (53%) | 54.5 (10.1) | 42.7 (9.6) | 36.0 (10.8) | 26.4 (8.6) | 10.4 (4.6) | 3.6 (2.5) |
West | 62 (16%) | 58,172 (12%) | 45.8 (8.1) | 37.4 (7.1) | 27.3 (7.7) | 19.5 (5.7) | 7.4 (4.8) | 2.7 (1.3) |
Teaching status | ||||||||
Nonteaching | 297 (77%) | 324,948 (66%) | 47.3 (14.6) | 36.9 (12.3) | 29.8 (12.0) | 21.3 (9.9) | 8.7 (5.4) | 3.3 (2.4) |
Teaching | 87 (23%) | 169,023 (34%) | 48.2 (16.0) | 38.8 (14.2) | 31.6 (14.5) | 22.1 (10.2) | 7.8 (4.4) | 2.7 (1.5) |
Staffed beds | * | ** | ||||||
22200 | 143 (37%) | 80,741 (16%) | 45.5 (16.9) | 35.2 (14.6) | 27.5 (14.0) | 20.1 (10.3) | 8.0 (6.2) | 3.5 (3.1) |
200400 | 137 (36%) | 177,286 (36%) | 47.7 (14.2) | 37.8 (12.0) | 30.5 (11.6) | 22.0 (10.0) | 8.4 (4.7) | 3.0 (1.6) |
400+ | 104 (27%) | 235944 (48%) | 50.1 (12.4) | 39.6 (10.6) | 33.5 (10.9) | 22.7 (9.3) | 9.3 (4.2) | 2.9 (1.4) |
Population serviced | * | * | ** | |||||
Rural | 119 (31%) | 102,799 (21%) | 48.4 (13.0) | 38.3 (10.6) | 29.2 (11.0) | 23.2 (9.3) | 7.5 (4.0) | 3.7 (3.0) |
Urban | 265 (69%) | 391,172 (79%) | 47.1 (15.7) | 36.9 (13.7) | 30.6 (13.2) | 20.7 (10.2) | 9.0 (5.6) | 2.9 (1.8) |
Geriatrician presence | ||||||||
No | 340 (89%) | 409,281 (83%) | 47.7 (15.3) | 37.6 (13.0) | 30.3 (12.8) | 21.7 (10.0) | 8.4 (5.3) | 3.2 (2.3) |
Yes | 44 (11%) | 84,690 (17%) | 45.8 (11.4) | 35.5 (10.6) | 29.4 (10.8) | 19.6 (9.4) | 9.3 (4.3) | 2.9 (1.6) |
Multivariable Model
In a multivariable logit model that included all patient, hospital, and physician characteristics and that accounted for clustering at the hospital, physician, and diagnosis levels, several characteristics were associated with HS‐PIM prescribing (Table 5). By far the most important predictor of use was hospital region. Compared with patients at hospitals in the Midwest, patients in the South (OR 1.63, 95% CI 1.591.67) and West (OR 1.43, 95% CI 1.381.47) were more likely and those in the Northeast were less likely (OR 0.85, 95% CI 0.830.88) to receive HS‐PIMs. Larger hospitals had higher HS‐PIM rates than smaller ones, but teaching status and rural or urban setting were not associated with HS‐PIM prescribing. The presence of geriatricians in a hospital was also associated with lower HS‐PIM prescribing for the entire hospital.
Effect (reference) | Odds ratio | 95% Confidence limits | |
---|---|---|---|
Age | |||
6574 years | 1.00 | ||
7584 years | 0.83 | 0.82 | 0.84 |
85+ years | 0.59 | 0.58 | 0.61 |
Sex | |||
Female | 1.00 | ||
Male | 0.85 | 0.83 | 0.86 |
Race | |||
White | 1.00 | ||
Black | 0.78 | 0.76 | 0.80 |
Hispanic | 0.84 | 0.81 | 0.87 |
American Indian | 0.97 | 0.88 | 1.07 |
Asian/Pacific Islander | 0.74 | 0.70 | 0.79 |
Other | 0.94 | 0.92 | 0.97 |
Marital Status | |||
Married/partner | 1.00 | ||
Single/separated/divorced | 0.96 | 0.94 | 0.98 |
Widowed | 0.96 | 0.95 | 0.98 |
Other | 0.93 | 0.90 | 0.95 |
Primary diagnosis | |||
Pneumonia | 1.00 | ||
COPD | 0.83 | 0.81 | 0.85 |
Heart failure | 1.14 | 1.12 | 1.16 |
Ischemic stroke | 0.84 | 0.82 | 0.86 |
Acute MI | 1.95 | 1.90 | 2.01 |
Urinary tract infection | 1.06 | 1.03 | 1.09 |
Chest pain | 0.87 | 0.84 | 0.89 |
Comorbidities (yes or no) | |||
Hypertension | 0.98 | 0.97 | 0.99 |
Diabetes | 0.98 | 0.97 | 1.00 |
Chronic lung disease | 1.11 | 1.10 | 1.13 |
Fluid and electrolyte disorders | 1.26 | 1.24 | 1.27 |
Anemia deficiencies | 1.17 | 1.15 | 1.18 |
Congestive heart failure | 1.34 | 1.32 | 1.37 |
Hypothyroidism | 1.13 | 1.11 | 1.15 |
Peripheral vascular disease | 1.09 | 1.06 | 1.11 |
Depression | 1.38 | 1.35 | 1.41 |
Neurological disorders | 0.89 | 0.87 | 0.91 |
Renal failure | 1.23 | 1.20 | 1.26 |
Obesity | 1.11 | 1.08 | 1.14 |
Payer type | |||
Managed care | 1.00 | ||
Not managed care | 1.04 | 1.02 | 1.06 |
Attending physician specialty | |||
Internal medicine | 1.00 | ||
Cardiology | 1.32 | 1.28 | 1.36 |
Family/general medicine | 0.99 | 0.97 | 1.01 |
Geriatrics | 0.69 | 0.61 | 0.78 |
Hospitalist | 0.90 | 0.84 | 0.96 |
Nephrology | 1.02 | 0.96 | 1.08 |
Neurology | 0.93 | 0.86 | 1.00 |
Pulmonology | 1.10 | 1.05 | 1.15 |
Setting | |||
Rural | 1.00 | ||
Urban | 1.02 | 1.00 | 1.05 |
Teaching status | |||
Nonteaching | 1.00 | ||
Teaching | 1.01 | 0.98 | 1.03 |
Number of beds | |||
22200 | 1.00 | ||
200400 | 1.08 | 1.05 | 1.11 |
400+ | 1.12 | 1.09 | 1.16 |
Region | |||
Midwest | 1.00 | ||
Northeast | 0.85 | 0.83 | 0.88 |
South | 1.63 | 1.59 | 1.67 |
West | 1.43 | 1.38 | 1.47 |
Geriatrician presence | |||
No | 1.00 | ||
Yes | 0.93 | 0.90 | 0.95 |
Physician specialty was also important. Adjusting for diagnosis attenuated some of this association, but compared with internists, cardiologists (OR 1.32, 95% CI 1.281.36) and pulmonologists (OR 1.10, 95% CI 1.051.15) were still more likely, hospitalists (OR 0.90, 95% CI 0.840.96) were less likely, and geriatricians (0.69, 95% CI 0.610.78) were least likely to prescribe any HS‐PIM.
Patient factors were also associated with HS‐PIM use. Compared with patients age 6574 years, patients older than 85 years were much less likely to receive an HS‐PIM (OR 0.59, CI 0.580.61), as to a lesser extent were nonwhites compared with whites and unmarried people compared with those who were married. Compared with patients with pneumonia, those with COPD, stroke, or chest pain were less likely and those with myocardial infarction and congestive heart failure were more likely to receive HS‐PIMs. Patients with a secondary diagnosis of depression were also at high risk (OR 1.38, CI 1.351.41).
DISCUSSION
Although Americans age 65 years and older make up less than 15% of the U.S. population, they consume about one third of all prescription drugs20 and account for one third of all hospital admissions.21 Using the Beers list, numerous studies have documented high rates of potentially inappropriate prescribing for community‐dwelling elderly and nursing home patients and, in some studies, an attendant risk of falling,2224 hip fracture,25, 26 hospitalization,13 or death.14 Applying these same criteria to a large sample of medical inpatients, we found that almost half received a potentially inappropriate drug, most of high severity. Moreover, the PIM prescribing rate varied substantially by region, hospital, and attending physician specialty. Although the use of PIMs was associated with patient age, comorbidities, and primary diagnosis, these patient factors explained only a small portion of the variation in prescribing practices across groups of physicians and hospitals.
Using consensus criteria, Beers originally found that 40% of the residents in 12 nursing homes received at least 1 PIM,8 and studies of community‐dwelling elderly demonstrated rates of 21% to 37%, with little change over time.6, 27, 28 Several small studies have examined inpatient prescribing.16, 17, 29, 30 The largest17 found that only 15% of elderly Italian inpatients received a PIM. Our finding, that 49% of inpatients had received at least 1 PIM, may partially reflect the high prevalence of use among elderly US patients in nursing homes and the community.
Regional variation has been demonstrated for ambulatory patients in the US6 and Europe.31 Zhan et al. found slightly higher rates of PIM use in the Midwest and the South (23%) than in the Northeast and the West (19%). Variation in Europe was greater, with 41% of patients in the Czech Republic versus 5.8% of patients in Denmark receiving at least 1 PIM. We found that region was the strongest predictor of in‐hospital HS‐PIM use, with patients in the South most likely and patients in the Northeast least likely to receive HS‐PIMs. This variation persisted even after adjusting for differences in other patient and hospital factors, suggesting that local custom played a large role in the decision to prescribe HS‐PIMs. Moreover, because outpatient rates are more uniform, these large differences seem limited to inpatient practice.
Patient factors have also been examined. Advanced age was associated with decreased PIM use in some studies17, 28, 31 but not in others.6, 27 We found increasing age to be strongly associated with decreased PIM use, suggesting that in the hospital, at least, doctors take care to avoid prescribing certain drugs to the frail elderly. Women appear to be consistently at higher risk than men,6, 27, 28, 31 and white patients are more at risk than those of other races.6 Our finding that certain diagnoses were associated with higher or lower rates has not been reported previously. The lower rates associated with stroke and COPD suggest that prescribers were aware that these patients were at increased risk of delirium and respiratory depression. The higher rates associated with myocardial infarction may have to do with the use of standardized order sets (eg, cath lab orders) that do not consider the age of the patient going for the procedure.
Admission to a geriatric service32 and intervention by a clinical pharmacist33 have been shown to decrease PIM prescribing at discharge. We noted that patients cared for by a geriatrician had the lowest rates of PIM prescribing during hospitalization as well and that hospitals with geriatricians had lower rates overall, possibly demonstrating that geriatricians had a ripple effect on their colleagues. Hospitalists also had lower rates than internists, supporting the notion that hospitalists provide higher‐quality inpatient care.
Our study had some important limitations. First, we only had access to inpatient administrative records. Thus, we could not identify which medications were continued from home and which were begun in the hospital, nor could we know the indications for which specific drugs were prescribed or who prescribed them. Based on published outpatient rates, however, we could assume that many of the drugs were started in the hospital and that others could have been discontinued but were not. Second, the Beers list was developed by the modified Delphi method; there was little empirical evidence of the danger of specific drugs, although some classes, such as benzodiazepines, opiates and digoxin, have been associated with inpatient falls.18, 3436 Furthermore, our administrative database did not allow us to balance the risks and benefits for particular patients; hence, the medications were only potentially inappropriate, and our study did not address the consequences of such prescribing. Although some of these drugs may be appropriate under certain circumstances, it is unlikely that these circumstances would vary by 60% across geographic regions or that internists would encounter these circumstances more often than do hospitalists. Thus, although we could not identify specific patients who received inappropriate medications, we did identify certain hospitals and even whole regions of the country in which the rate of inappropriate prescribing was high. Third, the Beers list, which was developed for outpatient use, may be less relevant in the inpatient setting. However, given that inpatients have more organ dysfunction and are at higher risk of delirium and falls, it may actually be more applicable to hospitalized patients. We similarly did not distinguish between single and multiple doses because the Beers list does not make such a distinction, and there is no empirical evidence that a single dose is safe. Indeed, patients are often at highest risk of falls immediately after initiation of therapy.3739 We did, however, exclude drugs such as laxatives, which may be appropriate for brief inpatient use but not for chronic use.
Our study also had a number of strengths. The large sample size, representing approximately 5% of annual inpatient admissions in the US over 2 years, offered an instructive look at the recent prescribing patterns of thousands of US physicians. We were able to identify many patient, physician, and hospital factors associated with PIM prescribing that have not previously been reported. Some of these factors, such as advanced age and comorbid diagnoses, suggest that physicians do tailor their treatment to individual patients. Nevertheless, patient factors accounted for only a small portion of the variation in prescribing. The largest variation, associated with regional, hospital, and physician factors, highlights the opportunity for improvement.
At the same time, our findings are encouraging for 2 reasons. First, most inappropriate prescribing involved only a handful of medications, so small changes in prescribing patterns could have a tremendous impact. Second, observing the practice of individual physicians and hospitals reveals what is possible. We found that in most specialties there were physicians who rarely or never used PIMs. We also found 7 hospitals, each with at least 300 cases, where no PIMs were ever prescribed.
Where should hospitals focus their efforts to prevent inappropriate prescribing? Our data highlight the complexity of the problem, which seems daunting. PIM prescribing is spread across all specialties, including geriatrics, and although cardiologists had the highest rate of prescribing, internists, who were more numerous, accounted for a much higher overall number of potentially inappropriate prescriptions. It would be instructive to study the 7 hospitals where PIMs were never prescribed or to interview those physicians who never prescribed PIMs, but the anonymous nature of our data would not allow for this. However, our data do suggest some directions. First, hospitals should become aware of their own rates of PIM use because measurement is the first step in quality improvement. Next, hospitals should focus efforts on reducing the use of the most common drugs. Eliminating just 3 drugs promethazine, diphenhydramine, and propoxyphenewould reduce the use of PIMs in 24% of elderly patients. Enlisting hospital pharmacists and electronic health records and reviewing standard order sets for elderly patients are potentially effective strategies. Finally, increasing the presence of geriatricians and hospitalists would be expected to have a modest impact.
In a representative sample of elderly inpatients, we found that almost half received a potentially inappropriate medication and that the rate of inappropriate prescribing varied widely among doctors and hospitals. Additional research is needed to distinguish which of the Beers drugs are most harmful and which patients are at highest risk. Research should also focus on understanding differences in prescribing patterns, perhaps by studying the outliers at both ends of the quality spectrum, and on techniques to minimize non‐patient‐centered variation.
- Explicit criteria for determining inappropriate medication use in nursing home residents. UCLA Division of Geriatric Medicine.Arch Intern Med.1991;151:1825–1832. , , , , , .
- Explicit criteria for determining potentially inappropriate medication use by the elderly. An update.Arch Intern Med.1997;157:1531–1536. .
- Updating the Beers criteria for potentially inappropriate medication use in older adults: results of a US consensus panel of experts.Arch Intern Med.2003;163:2716–2724. , , , , , .
- National Committee on Quality Assurance. Drugs to be Avoided in the Elderly. Available at: http://www.ncqa.org/Programs/HEDIS/2006/Volume2/NDC/DAE_06.xls. Accessed November 20,2006.
- Inappropriate prescribing for elderly Americans in a large outpatient population.Arch Intern Med.2004;164:1621–1625. , , , et al.
- Potentially inappropriate medication use in the community‐dwelling elderly: findings from the 1996 Medical Expenditure Panel Survey.JAMA.2001;286:2823–2829. , , , et al.
- Prescribing potentially inappropriate psychotropic medications to the ambulatory elderly.Arch Intern Med.2000;160:2825–2831. , .
- Inappropriate medication prescribing in skilled‐nursing facilities.Ann Intern Med.1992;117:684–689. , , , et al.
- Adverse outcomes associated with inappropriate drug use in nursing homes.Ann Pharmacother.2005;39:405–411. , , , et al.
- Inappropriate medication administration to the acutely ill elderly: a nationwide emergency department study, 1992–2000.J Am Geriatr Soc.2004;52:1847–1855. , , .
- Appropriateness of medication selection for older persons in an urban academic emergency department.Acad Emerg Med.1999;6:1232–1242. , , , et al.
- Use of the Beers criteria to predict adverse drug reactions among first‐visit elderly outpatients.Pharmacotherapy.2005;25:831–838. , , , , , .
- The association of inappropriate drug use with hospitalisation and mortality: a population‐based study of the very old.Drugs Aging.2005;22(1):69–82. , , .
- Hospitalization and death associated with potentially inappropriate medication prescriptions among elderly nursing home residents.Arch Intern Med.2005;165(1):68–74. , , , , .
- Potentially inappropriate prescribing for geriatric inpatients: an acute care of the elderly unit compared to a general medicine service.Consult Pharm.2003;18(1):37–42, 47–39. , , .
- Inappropriate medication use among frail elderly inpatients.Ann Pharmacother.2004;38(1):9–14. , , , et al.
- Inappropriate medication use among hospitalized older adults in Italy: results from the Italian Group of Pharmacoepidemiology in the Elderly.Eur J Clin Pharmacol.2003;59(2):157–162. , , , , , .
- Guided prescription of psychotropic medications for geriatric inpatients.Arch Intern Med.2005;165:802–807. , , , , , .
- Comorbidity measures for use with administrative data.Med Care.1998;36(1):8–27. , , , .
- Inadequate prescription‐drug coverage for Medicare enrollees—a call to action.N Engl J Med.1999;340:722–728. , .
- National and regional estimates on hospital use for all patients from the HCUP Nationwide Inpatient Sample (NIS). Agency for Healthcare Research and Quality (AHRQ). Available at: http://hcupnet.ahrq.gov/HCUPnet.jsp. Accessed October 12,2006.
- Drugs and falls in community‐dwelling older people: a national veterans study.Clin Ther.2006;28:619–630. , , , , , .
- Psychotropic medications and risk for falls among community‐dwelling frail older people: an observational study.J Gerontol A Biol Sci Med Sci.2005;60:622–626. , , , , , .
- Drugs and falls in older people: a systematic review and meta‐analysis: I. Psychotropic drugs.J Am Geriatr Soc.1999;47(1):30–39. , , .
- Propoxyphene use and risk for hip fractures in older adults.Am J Geriatr Pharmacother.2006;4:219–226. , , .
- Central nervous system active medications and risk for fractures in older women.Arch Intern Med.2003;163:949–957. , , , et al.
- Potentially inappropriate medication use by elderly persons in U.S. Health Maintenance Organizations, 2000–2001.J Am Geriatr Soc.2005;53:227–232. , , , et al.
- Inappropriate medication prescribing for elderly ambulatory care patients.Arch Intern Med.2004;164:305–312. .
- Adverse drug reactions in an elderly hospitalised population: inappropriate prescription is a leading cause.Drugs Aging.2005;22:767–777. , , .
- Use of inappropriate medications and their prognostic significance among in‐hospital and nursing home patients with and without dementia in Finland.Drugs Aging.2006;23:333–343. , , , , .
- Potentially inappropriate medication use among elderly home care patients in Europe.JAMA.2005;293:1348–1358. , , , et al.
- Impact of hospitalisation in an acute medical geriatric unit on potentially inappropriate medication use.Drugs Aging.2006;23(1):49–59. , , , , .
- Pharmacists and their effectiveness in ensuring the appropriateness of the chronic medication regimens of geriatric inpatients.Consult Pharm.2004;19:432–436. , .
- Benzodiazepines with different half‐life and falling in a hospitalized population: the GIFA study. Gruppo Italiano di Farmacovigilanza nell'Anziano.J Clin Epidemiol.2000;53:1222–1229. , , , , , .
- Relationship between the administration of selected medications and falls in hospitalized elderly patients.Ann Pharmacother.1995;29:354–358. , .
- The use of sedative/hypnotic medication and its correlation with falling down in the hospital.Sleep.1996;19:698–701. .
- Benzodiazepine use and hip fractures in the elderly: who is at greatest risk?Arch Intern Med.2004;164:1567–1572. , , , et al.
- Hazardous benzodiazepine regimens in the elderly: effects of half‐life, dosage, and duration on risk of hip fracture.Am J Psychiatry.2001;158:892–898. , , , , .
- A 5‐year prospective assessment of the risk associated with individual benzodiazepines and doses in new elderly users.J Am Geriatr Soc.2005;53:233–241. , , , , .
Medications can be considered inappropriate when their risk outweighs their benefit. The Beers list1 identifies medications that should be avoided in persons 65 years or older because they are ineffective or pose an unnecessarily high risk or because a safer alternative is available. Initially developed in 1991, the list has gained wide acceptance and has been updated twice.2, 3 In July 1999 it was adopted by the Centers for Medicare & Medicaid Services (CMS) for nursing home regulation, and in 2006 the National Committee on Quality Assurance adopted a modified version as a Health Plan Employer Data and Information Set (HEDIS) measure of quality of care for older Americans.4
A number of studies have demonstrated that inappropriate prescribing is common in the ambulatory setting,57 in nursing homes,8, 9 and in emergency departments10, 11 and that exposure to inappropriate medications is associated with increased risk of adverse drug reactions12 and hospitalization.13, 14 Initial studies of hospitalized patients1517 suggest that potentially inappropriate prescribing is also common among elderly inpatients and that reducing the misuse of psychotropic medications can prevent falls.18 We report on the incidence of and risk factors associated with potentially inappropriate prescribing in a large sample of hospitalized elders.
METHODS
Patients
We conducted a retrospective cohort study using data from 384 hospitals participating in Perspective (Premier, Inc., Charlotte, NC), a database developed for measuring quality and health care utilization. Participating hospitals represent all regions of the United States and are primarily small‐ to medium‐sized nonteaching hospitals most of which are in urban areas. Premier collects data elements from participating hospitals via a custom data extract from hospitals' decision support system. Hospitals aggregate the data elements into their decision support systems from multiple information technology systems including billing, medical records, pharmacy, and laboratory systems. In addition to the information contained in the standard hospital discharge file, Perspective includes a date‐stamped log of all billed items, including medications with dose and quantity, for individual patients.
We included patients at least 65 years old admitted between September 1, 2002, and June 30, 2005, with a principal diagnosis of acute myocardial infarction, chronic obstructive pulmonary disease, chest pain, community‐acquired pneumonia, congestive heart failure, ischemic stroke, or urinary tract infection. International Classification of Diseases, Ninth Revision (ICD‐9‐CM) codes were used to identify diagnoses. Patients cared for by an attending physician with a surgical specialty were excluded. The study protocol was approved by the institutional review board of Baystate Medical Center.
Data Elements
For each patient, Perspective contains fields for age, sex, race, marital status, insurance status, principal diagnosis, comorbidities, and specialty of the attending physician. Comorbidities were identified from ICD‐9‐CM secondary diagnosis codes and APR‐DRGs using Healthcare Cost and Utilization Project Comorbidity Software, version 3.1, based on the work of Elixhauser.19 Because almost all patients had Medicare coverage, plans were classified according to managed care status. Finally, for each patient we identified all medications administered, as well as discharge status, readmission rate, total costs, and length of stay. Hospitals were categorized by region (Northeast, South, Midwest, or West), bed size, setting (urban or rural), teaching status, and whether there were geriatricians.
Potentially Inappropriate Prescribing
Using the 2002 updated Beers criteria3 for potentially inappropriate medication (PIM) use in older adults, we identified the total number of PIMs administered to each patient during his or her hospital stay. We classified each PIM as either high or low severity based on the expert consensus expressed in the 1997 update of the Beers criteria.2 The list contains 48 PIMs and an additional 20 that should be avoided in patients with certain conditions. We did not include the second category of PIMs because we did not necessarily have sufficient patient information to make this determination. In addition, some of the standard PIMs, such as laxatives, although inappropriate for chronic outpatient use, could be appropriate in the hospital setting and were excluded from this analysis. Finally, several medications were considered inappropriate only above a given threshold (eg, lorazepam >3.0 mg/day) or for patients without a specific diagnosis (eg, digoxin >0.125 mg/day for patients without atrial fibrillation). We grouped PIMs that had similar side effects into 4 categories: sedatives, anticholinergics, causing orthostasis, or causing bleeding (Fig. 1).

Statistical Analysis
Summary statistics at the patient, physician, and hospital levels were constructed using frequencies and proportions for categorical data and means, standard deviations, medians, interquartile ranges, and box plots for continuous‐scale variables. Patients were identified as receiving a PIM if the drug was administered (above threshold dose where applicable) on at least 1 hospital day. We examined the association of each patient characteristic with use of any PIM, any high‐severity‐rated PIM, and each side effect category using chi‐square statistics. Kruskal‐Wallis analysis of variance was used to examine variation in hospital use rates by each hospital characteristic, and physician use rates for high‐severity PIMs by attending specialty. To examine whether it was feasible to avoid PIMs altogether, we compared individual hospitals as well as individual prescribers within their specialty, limiting the comparison to hospitals that contributed at least 100 patients and to physicians with at least 50 patients.
We developed a multivariable model for any high‐severity medication (HS‐PIM) use that included all patient, physician, and hospital characteristics except length of stay, mortality, cost, discharge status, and readmission rate. A generalized estimating equation model (SAS PROC GENMOD) with a logit link and a subcluster correlation structure was used to account for clustering at the hospital, physician, and diagnosis levels, adjusting for the clustering of primary diagnosis within physician level, nested within hospital level. Effects with P < .10 were retained in the model, and interaction effects were also evaluated for significance. Model fit was assessed using deviance and Pearson chi‐square statistics. All analyses were performed with SAS statistical software, version 9.1 (SAS Institute, Cary, NC).
RESULTS
We identified 519,853 patients at least 65 years old during the study period; 564 were excluded because of missing data for key variables or unclear principal diagnosis. An additional 25,318 were excluded because they were cared for by an attending with a surgical specialty. A total of 493,971 patients were included in the study (Table 1). Mean age was 78 years, and 24% of patients were 85 years or older. Forty‐three percent were male, 71% were white, and 39% were currently married. The most common principal diagnoses were community‐acquired pneumonia, congestive heart failure, and acute myocardial infarction. The most common comorbidities were hypertension, diabetes, and chronic pulmonary disease. Medicare was the primary payer for 91% of subjects, and 13% were in managed care plans. Most patients were cared for by internists (49%), family practitioners (18%), or cardiologists (17%). Only 1% of patients had a geriatrician as attending.
Characteristic | n (%) |
---|---|
| |
Age group | |
6574 years | 168,527 (34%) |
7584 years | 206,407 (42%) |
85+ years | 119,037 (24%) |
Sex | |
Male | 212,358 (43%) |
Female | 281,613 (57%) |
Race | |
White | 351,331 (71%) |
Black | 52,429 (11%) |
Hispanic | 18,057 (4%) |
American Indian | 1876 (0%) |
Asian/Pacific Islander | 5926 (1%) |
Other | 64,352 (13%) |
Marital status | |
Married/partner | 194,496 (39%) |
Widowed | 155,273 (31%) |
Single/separated/divorced | 75,964 (15%) |
Other | 68,238 (14%) |
Primary diagnosis | |
Pneumonia | 122,732 (25%) |
Heart failure | 109,071 (22%) |
Acute MI | 70,581 (14%) |
Ischemic stroke | 57,204 (12%) |
Chest pain | 50,404 (10%) |
COPD | 44,582 (9%) |
Urinary tract infection | 39,397 (8%) |
Comorbidities | |
Hypertension | 310,163 (63%) |
Diabetes | 151,755 (31%) |
Chronic pulmonary disease | 134,900 (27%) |
Fluid and electrolyte disorders | 128,703 (26%) |
Deficiency anemias | 92,668 (19%) |
Congestive heart failure | 69,201 (14%) |
Hypothyroidism | 68,711 (14%) |
Peripheral vascular disease | 47,244 (10%) |
Depression | 41,507 (8%) |
Other neurological disorders | 40,200 (8%) |
Renal failure | 38,134 (8%) |
Obesity | 25,143 (5%) |
Payer type | |
Not Managed care | 431,583 (87%) |
Managed care | 62,388 (13%) |
Attending physician specialty | |
Internal medicine (internist) | 241,982 (49%) |
Family/general medicine | 90,827 (18%) |
Cardiology | 83,317 (17%) |
Pulmonology | 21,163 (4%) |
Hospitalist | 14,924 (3%) |
Nephrology | 8257 (2%) |
Neurology | 5800 (1%) |
Geriatrics | 3099 (1%) |
Other* | 24,602 (5%) |
Mortality | |
Expired | 28,321 (6%) |
Alive | 465,650 (94%) |
Discharge status, n (% of survivors) | |
Home | 323,629 (66%) |
Nursing care | 119,468 (24%) |
Transfer/short‐term hospital | 13,531 (3%) |
Hospice | 9022 (2%) |
14‐Day readmission, n (% of survivors) | |
Yes | 35,309 (8%) |
No | 430,334 (92%) |
Length of stay (days), median (IQR) | 4 (2, 7) |
Total cost (dollars) | $5513 ($3366, $9902) |
Just under half of all patients (49%) received at least 1 PIM, and 6% received 3 or more (Table 2). Thirty‐eight percent received at least 1 drug with a high severity rating (HS‐PIM). The most common PIMs were promethazine, diphenhydramine, propoxyphene, clonidine, amiodarone, and lorazepam (>3 mg/day).
Patients, n (%) | |
---|---|
Number of PIMs | |
0 | 254,200 (51%) |
1 | 146,028 (30%) |
2 | 61,445 (12%) |
3 | 22,128 (4%) |
4+ | 10,170 (2%) |
Number of high‐severity‐rated PIMs | |
0 | 304,523 (62%) |
1 | 129,588 (26%) |
2 | 43,739 (9%) |
3 | 12,213 (2%) |
4+ | 3908 (1%) |
Use of any PIM by side effect class | |
Sedatives | 156,384 (32%) |
Anticholinergic effects | 109,293 (22%) |
Causing orthostasis | 43,805 (9%) |
Causing bleeding | 14,744 (3%) |
Most commonly prescribed | |
Promethazine | 49,888 (10%) |
Diphenhydramine | 45,458 (9%) |
Propoxyphene | 41,786 (8%) |
Clonidine | 34,765 (7%) |
Amiodarone | 34,318 (7%) |
Lorazepam (>3 mg/day) | 25,147 (5%) |
Patient, Physician, and Hospital Factors Associated with PIMs
Patient, physician, and hospital characteristics were all associated with use of PIMs (Table 3). In univariate analyses, older patients were less likely to receive any class of PIM, and this difference was accentuated for HS‐PIMs. Women, American Indians, married people, and those not in managed care plans were slightly more likely to receive PIMs, whereas patients admitted with acute myocardial infarction or congestive heart failure were even more likely to receive PIMs (P < .0001 for all comparisons).
Patient characteristic | Any PIM n (row %) | Any high‐severity PIM n (row %) | Sedatives n (row %) | Anticholinergic effects n (row %) | Causing orthostasis n (row %) | Causing bleeding n (row %) |
---|---|---|---|---|---|---|
| ||||||
Overall | 239,771 (49%) | 189,448 (38%) | 156,384 (32%) | 109,293 (22%) | 43,805 (9%) | 14,744 (3%) |
Age group | ||||||
6574 years | 89,168 (53%) | 72,573 (43%) | 61,399 (36%) | 44,792 (27%) | 15,799 (9%) | 6655 (4%) |
7584 years | 100,787 (49%) | 79,595 (39%) | 65,034 (32%) | 45,121 (22%) | 18,519 (9%) | 5727 (3%) |
85+ years | 49,816 (42%) | 37,280 (31%) | 29,951 (25%) | 19,380 (16%) | 9487 (8%) | 2362 (2%) |
Sex | ||||||
Male | 100,824 (47%) | 79,535 (37%) | 63,591 (30%) | 42,754 (20%) | 17,885 (8%) | 5771 (3%) |
Female | 138,947 (49%) | 109,913 (39%) | 92,793 (33%) | 66,539 (24%) | 25,920 (9%) | 8973 (3%) |
Race | ||||||
White | 173,481 (49%) | 139,941 (40%) | 112,556 (32%) | 81,097 (23%) | 27,555 (8%) | 10,590 (3%) |
Black | 26,793 (51%) | 18,655 (36%) | 18,720 (36%) | 11,263 (21%) | 8925 (17%) | 1536 (3%) |
Hispanic | 8509 (47%) | 6370 (35%) | 5549 (31%) | 3505 (19%) | 2047 (11%) | 648 (4%) |
American Indian | 1091 (58%) | 849 (45%) | 818 (44%) | 563 (30%) | 190 (10%) | 76 (4%) |
Asian/Pacific Islander | 2386 (40%) | 1896 (32%) | 1420 (24%) | 1023 (17%) | 519 (9%) | 127 (2%) |
Other | 27,511 (43%) | 21,737 (34%) | 17,321 (27%) | 11,842 (18%) | 4569 (7%) | 1767 (3%) |
Marital status | ||||||
Married/partner | 96,874 (50%) | 77,803 (40%) | 63,303 (33%) | 45,042 (23%) | 16,765 (9%) | 5969 (3%) |
Widowed | 74,622 (48%) | 58,012 (37%) | 48,367 (31%) | 33,516 (22%) | 13,865 (9%) | 4354 (3%) |
Single/separated/divorced | 36,583 (48%) | 28,799 (38%) | 24,251 (32%) | 16,115 (21%) | 7229 (10%) | 2399 (3%) |
Other | 31,692 (46%) | 24,834 (36%) | 20,463 (30%) | 14,620 (21%) | 5946 (9%) | 2022 (3%) |
Primary diagnosis | ||||||
Pneumonia | 56,845 (46%) | 46,271 (38%) | 35,353 (29%) | 25,484 (21%) | 9184 (7%) | 4155 (3%) |
Heart failure | 56,460 (52%) | 42,231 (39%) | 34,340 (31%) | 22,093 (20%) | 10,117 (9%) | 1945 (2%) |
Acute MI | 43,046 (61%) | 37,849 (54%) | 32,560 (46%) | 25,568 (36%) | 4738 (7%) | 2549 (4%) |
Ischemic stroke | 25,763 (45%) | 17,613 (31%) | 18,500 (32%) | 8742 (15%) | 9644 (17%) | 1384 (2%) |
Chest pain | 20,655 (41%) | 16,363 (32%) | 13,536 (27%) | 10,520 (21%) | 3474 (7%) | 2027 (4%) |
COPD | 18,876 (42%) | 14,626 (33%) | 12,087 (27%) | 8096 (18%) | 3209 (7%) | 1139 (3%) |
Urinary tract infection | 18,126 (46%) | 14,495 (37%) | 10,008 (25%) | 8790 (22%) | 3439 (9%) | 1545 (4%) |
Payer type | ||||||
Nonmanaged care | 212,150 (49%) | 168,013 (39%) | 138,679 (32%) | 97,776 (23%) | 38,341 (9%) | 12,868 (3%) |
Managed care | 27,621 (44%) | 21,435 (34%) | 17,705 (28%) | 11,517 (18%) | 5464 (9%) | 1876 (3%) |
Attending physician specialty* | ||||||
Internal medicine (internist%) | 112,664 (47%) | 86,907 (36%) | 71,382 (30%) | 48,746 (20%) | 23,221 (10%) | 7086 (3%) |
Family/general medicine | 41,303 (45%) | 32,338 (36%) | 25,653 (28%) | 18,274 (20%) | 7660 (8%) | 2852 (3%) |
Cardiology | 48,485 (58%) | 40,752 (49%) | 34,859 (42%) | 25,792 (31%) | 5455 (7%) | 2542 (3%) |
Pulmonology | 10,231 (48%) | 8105 (38%) | 6746 (32%) | 4064 (19%) | 1739 (8%) | 574 (3%) |
Hospitalist | 7003 (47%) | 5443 (36%) | 4447 (30%) | 3179 (21%) | 1471 (10%) | 463 (3%) |
Nephrology | 4508 (55%) | 3388 (41%) | 3132 (38%) | 2054 (25%) | 1326 (16%) | 198 (2%) |
Neurology | 2420 (42%) | 1789 (31%) | 1625 (28%) | 851 (15%) | 699 (12%) | 174 (3%) |
Geriatrics | 1020 (33%) | 785 (25%) | 596 (19%) | 404 (13%) | 196 (6%) | 41 (1%) |
The HS‐PIM prescribing varied substantially by attending specialty (Fig. 2). Internists, family practitioners, and hospitalists all had similar median rates (33%), cardiologists had a higher median rate (48%), and geriatricians had a lower rate (24%). The most common PIM also differed by specialty: whereas promethazine was the most commonly prescribed drug across most specialties, nephrologists and neurologists used clonidine, pulmonologists used lorazepam, and cardiologists used diphenhydramine most often. Among the 8% of physicians who saw at least 50 patients, there was also great variation in each specialty (Fig. 2). Among internists and cardiologists who saw at least 50 patients, the high‐severity PIM usage rate ranged from 0% to more than 90%.
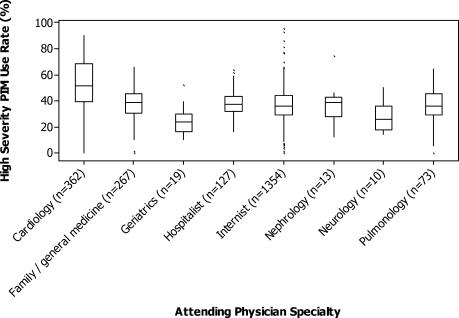
There was substantial variation in PIM usage among hospitals, most notably by region. The mean proportion of patients receiving PIMs ranged from 34% at hospitals in the Northeast to 55% at hospitals in the South (Table 4). Smaller hospitals and those in urban settings had slightly lower rates, as did those that had geriatricians on staff. The teaching status of the hospital had little effect. Variation at the individual hospital level was extreme (Fig. 3). Although half of all hospitals had rates between 43% and 58%, in 7 hospitals with more than 300 encounters each, PIMs were never prescribed for geriatric patients.
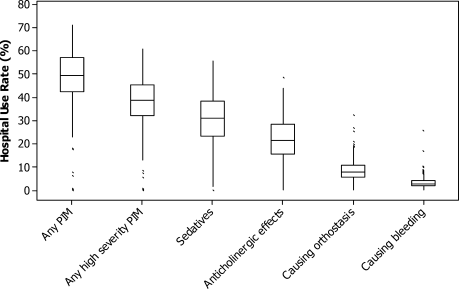
Hospitals Total = 384 n (%) | Patients N = 49,3971 n (%) | Any PIM Mean = 48.2 Mean (SD) | Any high‐severity PIM Mean = 38.7 Mean (SD) | Sedatives Mean = 30.2 Mean (SD) | Anticholinergic effects Mean = 21.5 Mean (SD) | Causing orthostasis Mean = 8.5 Mean (SD) | Causing bleeding Mean = 3.1 Mean (SD) | |
---|---|---|---|---|---|---|---|---|
| ||||||||
Hospital region | *** | *** | *** | *** | *** | ** | ||
Midwest | 76 (20%) | 95,791 (19%) | 38.8 (19.7) | 30.0 (16.4) | 24.3 (13.8) | 15.1 (9.9) | 6.9 (6.3) | 3.1 (2.3) |
Northeast | 47 (12%) | 79,138 (16%) | 34.1 (12.6) | 26.2 (11.2) | 19.0 (9.2) | 13.5 (8.1) | 4.9 (2.3) | 2.1 (1.6) |
South | 199 (52%) | 260,870 (53%) | 54.5 (10.1) | 42.7 (9.6) | 36.0 (10.8) | 26.4 (8.6) | 10.4 (4.6) | 3.6 (2.5) |
West | 62 (16%) | 58,172 (12%) | 45.8 (8.1) | 37.4 (7.1) | 27.3 (7.7) | 19.5 (5.7) | 7.4 (4.8) | 2.7 (1.3) |
Teaching status | ||||||||
Nonteaching | 297 (77%) | 324,948 (66%) | 47.3 (14.6) | 36.9 (12.3) | 29.8 (12.0) | 21.3 (9.9) | 8.7 (5.4) | 3.3 (2.4) |
Teaching | 87 (23%) | 169,023 (34%) | 48.2 (16.0) | 38.8 (14.2) | 31.6 (14.5) | 22.1 (10.2) | 7.8 (4.4) | 2.7 (1.5) |
Staffed beds | * | ** | ||||||
22200 | 143 (37%) | 80,741 (16%) | 45.5 (16.9) | 35.2 (14.6) | 27.5 (14.0) | 20.1 (10.3) | 8.0 (6.2) | 3.5 (3.1) |
200400 | 137 (36%) | 177,286 (36%) | 47.7 (14.2) | 37.8 (12.0) | 30.5 (11.6) | 22.0 (10.0) | 8.4 (4.7) | 3.0 (1.6) |
400+ | 104 (27%) | 235944 (48%) | 50.1 (12.4) | 39.6 (10.6) | 33.5 (10.9) | 22.7 (9.3) | 9.3 (4.2) | 2.9 (1.4) |
Population serviced | * | * | ** | |||||
Rural | 119 (31%) | 102,799 (21%) | 48.4 (13.0) | 38.3 (10.6) | 29.2 (11.0) | 23.2 (9.3) | 7.5 (4.0) | 3.7 (3.0) |
Urban | 265 (69%) | 391,172 (79%) | 47.1 (15.7) | 36.9 (13.7) | 30.6 (13.2) | 20.7 (10.2) | 9.0 (5.6) | 2.9 (1.8) |
Geriatrician presence | ||||||||
No | 340 (89%) | 409,281 (83%) | 47.7 (15.3) | 37.6 (13.0) | 30.3 (12.8) | 21.7 (10.0) | 8.4 (5.3) | 3.2 (2.3) |
Yes | 44 (11%) | 84,690 (17%) | 45.8 (11.4) | 35.5 (10.6) | 29.4 (10.8) | 19.6 (9.4) | 9.3 (4.3) | 2.9 (1.6) |
Multivariable Model
In a multivariable logit model that included all patient, hospital, and physician characteristics and that accounted for clustering at the hospital, physician, and diagnosis levels, several characteristics were associated with HS‐PIM prescribing (Table 5). By far the most important predictor of use was hospital region. Compared with patients at hospitals in the Midwest, patients in the South (OR 1.63, 95% CI 1.591.67) and West (OR 1.43, 95% CI 1.381.47) were more likely and those in the Northeast were less likely (OR 0.85, 95% CI 0.830.88) to receive HS‐PIMs. Larger hospitals had higher HS‐PIM rates than smaller ones, but teaching status and rural or urban setting were not associated with HS‐PIM prescribing. The presence of geriatricians in a hospital was also associated with lower HS‐PIM prescribing for the entire hospital.
Effect (reference) | Odds ratio | 95% Confidence limits | |
---|---|---|---|
Age | |||
6574 years | 1.00 | ||
7584 years | 0.83 | 0.82 | 0.84 |
85+ years | 0.59 | 0.58 | 0.61 |
Sex | |||
Female | 1.00 | ||
Male | 0.85 | 0.83 | 0.86 |
Race | |||
White | 1.00 | ||
Black | 0.78 | 0.76 | 0.80 |
Hispanic | 0.84 | 0.81 | 0.87 |
American Indian | 0.97 | 0.88 | 1.07 |
Asian/Pacific Islander | 0.74 | 0.70 | 0.79 |
Other | 0.94 | 0.92 | 0.97 |
Marital Status | |||
Married/partner | 1.00 | ||
Single/separated/divorced | 0.96 | 0.94 | 0.98 |
Widowed | 0.96 | 0.95 | 0.98 |
Other | 0.93 | 0.90 | 0.95 |
Primary diagnosis | |||
Pneumonia | 1.00 | ||
COPD | 0.83 | 0.81 | 0.85 |
Heart failure | 1.14 | 1.12 | 1.16 |
Ischemic stroke | 0.84 | 0.82 | 0.86 |
Acute MI | 1.95 | 1.90 | 2.01 |
Urinary tract infection | 1.06 | 1.03 | 1.09 |
Chest pain | 0.87 | 0.84 | 0.89 |
Comorbidities (yes or no) | |||
Hypertension | 0.98 | 0.97 | 0.99 |
Diabetes | 0.98 | 0.97 | 1.00 |
Chronic lung disease | 1.11 | 1.10 | 1.13 |
Fluid and electrolyte disorders | 1.26 | 1.24 | 1.27 |
Anemia deficiencies | 1.17 | 1.15 | 1.18 |
Congestive heart failure | 1.34 | 1.32 | 1.37 |
Hypothyroidism | 1.13 | 1.11 | 1.15 |
Peripheral vascular disease | 1.09 | 1.06 | 1.11 |
Depression | 1.38 | 1.35 | 1.41 |
Neurological disorders | 0.89 | 0.87 | 0.91 |
Renal failure | 1.23 | 1.20 | 1.26 |
Obesity | 1.11 | 1.08 | 1.14 |
Payer type | |||
Managed care | 1.00 | ||
Not managed care | 1.04 | 1.02 | 1.06 |
Attending physician specialty | |||
Internal medicine | 1.00 | ||
Cardiology | 1.32 | 1.28 | 1.36 |
Family/general medicine | 0.99 | 0.97 | 1.01 |
Geriatrics | 0.69 | 0.61 | 0.78 |
Hospitalist | 0.90 | 0.84 | 0.96 |
Nephrology | 1.02 | 0.96 | 1.08 |
Neurology | 0.93 | 0.86 | 1.00 |
Pulmonology | 1.10 | 1.05 | 1.15 |
Setting | |||
Rural | 1.00 | ||
Urban | 1.02 | 1.00 | 1.05 |
Teaching status | |||
Nonteaching | 1.00 | ||
Teaching | 1.01 | 0.98 | 1.03 |
Number of beds | |||
22200 | 1.00 | ||
200400 | 1.08 | 1.05 | 1.11 |
400+ | 1.12 | 1.09 | 1.16 |
Region | |||
Midwest | 1.00 | ||
Northeast | 0.85 | 0.83 | 0.88 |
South | 1.63 | 1.59 | 1.67 |
West | 1.43 | 1.38 | 1.47 |
Geriatrician presence | |||
No | 1.00 | ||
Yes | 0.93 | 0.90 | 0.95 |
Physician specialty was also important. Adjusting for diagnosis attenuated some of this association, but compared with internists, cardiologists (OR 1.32, 95% CI 1.281.36) and pulmonologists (OR 1.10, 95% CI 1.051.15) were still more likely, hospitalists (OR 0.90, 95% CI 0.840.96) were less likely, and geriatricians (0.69, 95% CI 0.610.78) were least likely to prescribe any HS‐PIM.
Patient factors were also associated with HS‐PIM use. Compared with patients age 6574 years, patients older than 85 years were much less likely to receive an HS‐PIM (OR 0.59, CI 0.580.61), as to a lesser extent were nonwhites compared with whites and unmarried people compared with those who were married. Compared with patients with pneumonia, those with COPD, stroke, or chest pain were less likely and those with myocardial infarction and congestive heart failure were more likely to receive HS‐PIMs. Patients with a secondary diagnosis of depression were also at high risk (OR 1.38, CI 1.351.41).
DISCUSSION
Although Americans age 65 years and older make up less than 15% of the U.S. population, they consume about one third of all prescription drugs20 and account for one third of all hospital admissions.21 Using the Beers list, numerous studies have documented high rates of potentially inappropriate prescribing for community‐dwelling elderly and nursing home patients and, in some studies, an attendant risk of falling,2224 hip fracture,25, 26 hospitalization,13 or death.14 Applying these same criteria to a large sample of medical inpatients, we found that almost half received a potentially inappropriate drug, most of high severity. Moreover, the PIM prescribing rate varied substantially by region, hospital, and attending physician specialty. Although the use of PIMs was associated with patient age, comorbidities, and primary diagnosis, these patient factors explained only a small portion of the variation in prescribing practices across groups of physicians and hospitals.
Using consensus criteria, Beers originally found that 40% of the residents in 12 nursing homes received at least 1 PIM,8 and studies of community‐dwelling elderly demonstrated rates of 21% to 37%, with little change over time.6, 27, 28 Several small studies have examined inpatient prescribing.16, 17, 29, 30 The largest17 found that only 15% of elderly Italian inpatients received a PIM. Our finding, that 49% of inpatients had received at least 1 PIM, may partially reflect the high prevalence of use among elderly US patients in nursing homes and the community.
Regional variation has been demonstrated for ambulatory patients in the US6 and Europe.31 Zhan et al. found slightly higher rates of PIM use in the Midwest and the South (23%) than in the Northeast and the West (19%). Variation in Europe was greater, with 41% of patients in the Czech Republic versus 5.8% of patients in Denmark receiving at least 1 PIM. We found that region was the strongest predictor of in‐hospital HS‐PIM use, with patients in the South most likely and patients in the Northeast least likely to receive HS‐PIMs. This variation persisted even after adjusting for differences in other patient and hospital factors, suggesting that local custom played a large role in the decision to prescribe HS‐PIMs. Moreover, because outpatient rates are more uniform, these large differences seem limited to inpatient practice.
Patient factors have also been examined. Advanced age was associated with decreased PIM use in some studies17, 28, 31 but not in others.6, 27 We found increasing age to be strongly associated with decreased PIM use, suggesting that in the hospital, at least, doctors take care to avoid prescribing certain drugs to the frail elderly. Women appear to be consistently at higher risk than men,6, 27, 28, 31 and white patients are more at risk than those of other races.6 Our finding that certain diagnoses were associated with higher or lower rates has not been reported previously. The lower rates associated with stroke and COPD suggest that prescribers were aware that these patients were at increased risk of delirium and respiratory depression. The higher rates associated with myocardial infarction may have to do with the use of standardized order sets (eg, cath lab orders) that do not consider the age of the patient going for the procedure.
Admission to a geriatric service32 and intervention by a clinical pharmacist33 have been shown to decrease PIM prescribing at discharge. We noted that patients cared for by a geriatrician had the lowest rates of PIM prescribing during hospitalization as well and that hospitals with geriatricians had lower rates overall, possibly demonstrating that geriatricians had a ripple effect on their colleagues. Hospitalists also had lower rates than internists, supporting the notion that hospitalists provide higher‐quality inpatient care.
Our study had some important limitations. First, we only had access to inpatient administrative records. Thus, we could not identify which medications were continued from home and which were begun in the hospital, nor could we know the indications for which specific drugs were prescribed or who prescribed them. Based on published outpatient rates, however, we could assume that many of the drugs were started in the hospital and that others could have been discontinued but were not. Second, the Beers list was developed by the modified Delphi method; there was little empirical evidence of the danger of specific drugs, although some classes, such as benzodiazepines, opiates and digoxin, have been associated with inpatient falls.18, 3436 Furthermore, our administrative database did not allow us to balance the risks and benefits for particular patients; hence, the medications were only potentially inappropriate, and our study did not address the consequences of such prescribing. Although some of these drugs may be appropriate under certain circumstances, it is unlikely that these circumstances would vary by 60% across geographic regions or that internists would encounter these circumstances more often than do hospitalists. Thus, although we could not identify specific patients who received inappropriate medications, we did identify certain hospitals and even whole regions of the country in which the rate of inappropriate prescribing was high. Third, the Beers list, which was developed for outpatient use, may be less relevant in the inpatient setting. However, given that inpatients have more organ dysfunction and are at higher risk of delirium and falls, it may actually be more applicable to hospitalized patients. We similarly did not distinguish between single and multiple doses because the Beers list does not make such a distinction, and there is no empirical evidence that a single dose is safe. Indeed, patients are often at highest risk of falls immediately after initiation of therapy.3739 We did, however, exclude drugs such as laxatives, which may be appropriate for brief inpatient use but not for chronic use.
Our study also had a number of strengths. The large sample size, representing approximately 5% of annual inpatient admissions in the US over 2 years, offered an instructive look at the recent prescribing patterns of thousands of US physicians. We were able to identify many patient, physician, and hospital factors associated with PIM prescribing that have not previously been reported. Some of these factors, such as advanced age and comorbid diagnoses, suggest that physicians do tailor their treatment to individual patients. Nevertheless, patient factors accounted for only a small portion of the variation in prescribing. The largest variation, associated with regional, hospital, and physician factors, highlights the opportunity for improvement.
At the same time, our findings are encouraging for 2 reasons. First, most inappropriate prescribing involved only a handful of medications, so small changes in prescribing patterns could have a tremendous impact. Second, observing the practice of individual physicians and hospitals reveals what is possible. We found that in most specialties there were physicians who rarely or never used PIMs. We also found 7 hospitals, each with at least 300 cases, where no PIMs were ever prescribed.
Where should hospitals focus their efforts to prevent inappropriate prescribing? Our data highlight the complexity of the problem, which seems daunting. PIM prescribing is spread across all specialties, including geriatrics, and although cardiologists had the highest rate of prescribing, internists, who were more numerous, accounted for a much higher overall number of potentially inappropriate prescriptions. It would be instructive to study the 7 hospitals where PIMs were never prescribed or to interview those physicians who never prescribed PIMs, but the anonymous nature of our data would not allow for this. However, our data do suggest some directions. First, hospitals should become aware of their own rates of PIM use because measurement is the first step in quality improvement. Next, hospitals should focus efforts on reducing the use of the most common drugs. Eliminating just 3 drugs promethazine, diphenhydramine, and propoxyphenewould reduce the use of PIMs in 24% of elderly patients. Enlisting hospital pharmacists and electronic health records and reviewing standard order sets for elderly patients are potentially effective strategies. Finally, increasing the presence of geriatricians and hospitalists would be expected to have a modest impact.
In a representative sample of elderly inpatients, we found that almost half received a potentially inappropriate medication and that the rate of inappropriate prescribing varied widely among doctors and hospitals. Additional research is needed to distinguish which of the Beers drugs are most harmful and which patients are at highest risk. Research should also focus on understanding differences in prescribing patterns, perhaps by studying the outliers at both ends of the quality spectrum, and on techniques to minimize non‐patient‐centered variation.
Medications can be considered inappropriate when their risk outweighs their benefit. The Beers list1 identifies medications that should be avoided in persons 65 years or older because they are ineffective or pose an unnecessarily high risk or because a safer alternative is available. Initially developed in 1991, the list has gained wide acceptance and has been updated twice.2, 3 In July 1999 it was adopted by the Centers for Medicare & Medicaid Services (CMS) for nursing home regulation, and in 2006 the National Committee on Quality Assurance adopted a modified version as a Health Plan Employer Data and Information Set (HEDIS) measure of quality of care for older Americans.4
A number of studies have demonstrated that inappropriate prescribing is common in the ambulatory setting,57 in nursing homes,8, 9 and in emergency departments10, 11 and that exposure to inappropriate medications is associated with increased risk of adverse drug reactions12 and hospitalization.13, 14 Initial studies of hospitalized patients1517 suggest that potentially inappropriate prescribing is also common among elderly inpatients and that reducing the misuse of psychotropic medications can prevent falls.18 We report on the incidence of and risk factors associated with potentially inappropriate prescribing in a large sample of hospitalized elders.
METHODS
Patients
We conducted a retrospective cohort study using data from 384 hospitals participating in Perspective (Premier, Inc., Charlotte, NC), a database developed for measuring quality and health care utilization. Participating hospitals represent all regions of the United States and are primarily small‐ to medium‐sized nonteaching hospitals most of which are in urban areas. Premier collects data elements from participating hospitals via a custom data extract from hospitals' decision support system. Hospitals aggregate the data elements into their decision support systems from multiple information technology systems including billing, medical records, pharmacy, and laboratory systems. In addition to the information contained in the standard hospital discharge file, Perspective includes a date‐stamped log of all billed items, including medications with dose and quantity, for individual patients.
We included patients at least 65 years old admitted between September 1, 2002, and June 30, 2005, with a principal diagnosis of acute myocardial infarction, chronic obstructive pulmonary disease, chest pain, community‐acquired pneumonia, congestive heart failure, ischemic stroke, or urinary tract infection. International Classification of Diseases, Ninth Revision (ICD‐9‐CM) codes were used to identify diagnoses. Patients cared for by an attending physician with a surgical specialty were excluded. The study protocol was approved by the institutional review board of Baystate Medical Center.
Data Elements
For each patient, Perspective contains fields for age, sex, race, marital status, insurance status, principal diagnosis, comorbidities, and specialty of the attending physician. Comorbidities were identified from ICD‐9‐CM secondary diagnosis codes and APR‐DRGs using Healthcare Cost and Utilization Project Comorbidity Software, version 3.1, based on the work of Elixhauser.19 Because almost all patients had Medicare coverage, plans were classified according to managed care status. Finally, for each patient we identified all medications administered, as well as discharge status, readmission rate, total costs, and length of stay. Hospitals were categorized by region (Northeast, South, Midwest, or West), bed size, setting (urban or rural), teaching status, and whether there were geriatricians.
Potentially Inappropriate Prescribing
Using the 2002 updated Beers criteria3 for potentially inappropriate medication (PIM) use in older adults, we identified the total number of PIMs administered to each patient during his or her hospital stay. We classified each PIM as either high or low severity based on the expert consensus expressed in the 1997 update of the Beers criteria.2 The list contains 48 PIMs and an additional 20 that should be avoided in patients with certain conditions. We did not include the second category of PIMs because we did not necessarily have sufficient patient information to make this determination. In addition, some of the standard PIMs, such as laxatives, although inappropriate for chronic outpatient use, could be appropriate in the hospital setting and were excluded from this analysis. Finally, several medications were considered inappropriate only above a given threshold (eg, lorazepam >3.0 mg/day) or for patients without a specific diagnosis (eg, digoxin >0.125 mg/day for patients without atrial fibrillation). We grouped PIMs that had similar side effects into 4 categories: sedatives, anticholinergics, causing orthostasis, or causing bleeding (Fig. 1).

Statistical Analysis
Summary statistics at the patient, physician, and hospital levels were constructed using frequencies and proportions for categorical data and means, standard deviations, medians, interquartile ranges, and box plots for continuous‐scale variables. Patients were identified as receiving a PIM if the drug was administered (above threshold dose where applicable) on at least 1 hospital day. We examined the association of each patient characteristic with use of any PIM, any high‐severity‐rated PIM, and each side effect category using chi‐square statistics. Kruskal‐Wallis analysis of variance was used to examine variation in hospital use rates by each hospital characteristic, and physician use rates for high‐severity PIMs by attending specialty. To examine whether it was feasible to avoid PIMs altogether, we compared individual hospitals as well as individual prescribers within their specialty, limiting the comparison to hospitals that contributed at least 100 patients and to physicians with at least 50 patients.
We developed a multivariable model for any high‐severity medication (HS‐PIM) use that included all patient, physician, and hospital characteristics except length of stay, mortality, cost, discharge status, and readmission rate. A generalized estimating equation model (SAS PROC GENMOD) with a logit link and a subcluster correlation structure was used to account for clustering at the hospital, physician, and diagnosis levels, adjusting for the clustering of primary diagnosis within physician level, nested within hospital level. Effects with P < .10 were retained in the model, and interaction effects were also evaluated for significance. Model fit was assessed using deviance and Pearson chi‐square statistics. All analyses were performed with SAS statistical software, version 9.1 (SAS Institute, Cary, NC).
RESULTS
We identified 519,853 patients at least 65 years old during the study period; 564 were excluded because of missing data for key variables or unclear principal diagnosis. An additional 25,318 were excluded because they were cared for by an attending with a surgical specialty. A total of 493,971 patients were included in the study (Table 1). Mean age was 78 years, and 24% of patients were 85 years or older. Forty‐three percent were male, 71% were white, and 39% were currently married. The most common principal diagnoses were community‐acquired pneumonia, congestive heart failure, and acute myocardial infarction. The most common comorbidities were hypertension, diabetes, and chronic pulmonary disease. Medicare was the primary payer for 91% of subjects, and 13% were in managed care plans. Most patients were cared for by internists (49%), family practitioners (18%), or cardiologists (17%). Only 1% of patients had a geriatrician as attending.
Characteristic | n (%) |
---|---|
| |
Age group | |
6574 years | 168,527 (34%) |
7584 years | 206,407 (42%) |
85+ years | 119,037 (24%) |
Sex | |
Male | 212,358 (43%) |
Female | 281,613 (57%) |
Race | |
White | 351,331 (71%) |
Black | 52,429 (11%) |
Hispanic | 18,057 (4%) |
American Indian | 1876 (0%) |
Asian/Pacific Islander | 5926 (1%) |
Other | 64,352 (13%) |
Marital status | |
Married/partner | 194,496 (39%) |
Widowed | 155,273 (31%) |
Single/separated/divorced | 75,964 (15%) |
Other | 68,238 (14%) |
Primary diagnosis | |
Pneumonia | 122,732 (25%) |
Heart failure | 109,071 (22%) |
Acute MI | 70,581 (14%) |
Ischemic stroke | 57,204 (12%) |
Chest pain | 50,404 (10%) |
COPD | 44,582 (9%) |
Urinary tract infection | 39,397 (8%) |
Comorbidities | |
Hypertension | 310,163 (63%) |
Diabetes | 151,755 (31%) |
Chronic pulmonary disease | 134,900 (27%) |
Fluid and electrolyte disorders | 128,703 (26%) |
Deficiency anemias | 92,668 (19%) |
Congestive heart failure | 69,201 (14%) |
Hypothyroidism | 68,711 (14%) |
Peripheral vascular disease | 47,244 (10%) |
Depression | 41,507 (8%) |
Other neurological disorders | 40,200 (8%) |
Renal failure | 38,134 (8%) |
Obesity | 25,143 (5%) |
Payer type | |
Not Managed care | 431,583 (87%) |
Managed care | 62,388 (13%) |
Attending physician specialty | |
Internal medicine (internist) | 241,982 (49%) |
Family/general medicine | 90,827 (18%) |
Cardiology | 83,317 (17%) |
Pulmonology | 21,163 (4%) |
Hospitalist | 14,924 (3%) |
Nephrology | 8257 (2%) |
Neurology | 5800 (1%) |
Geriatrics | 3099 (1%) |
Other* | 24,602 (5%) |
Mortality | |
Expired | 28,321 (6%) |
Alive | 465,650 (94%) |
Discharge status, n (% of survivors) | |
Home | 323,629 (66%) |
Nursing care | 119,468 (24%) |
Transfer/short‐term hospital | 13,531 (3%) |
Hospice | 9022 (2%) |
14‐Day readmission, n (% of survivors) | |
Yes | 35,309 (8%) |
No | 430,334 (92%) |
Length of stay (days), median (IQR) | 4 (2, 7) |
Total cost (dollars) | $5513 ($3366, $9902) |
Just under half of all patients (49%) received at least 1 PIM, and 6% received 3 or more (Table 2). Thirty‐eight percent received at least 1 drug with a high severity rating (HS‐PIM). The most common PIMs were promethazine, diphenhydramine, propoxyphene, clonidine, amiodarone, and lorazepam (>3 mg/day).
Patients, n (%) | |
---|---|
Number of PIMs | |
0 | 254,200 (51%) |
1 | 146,028 (30%) |
2 | 61,445 (12%) |
3 | 22,128 (4%) |
4+ | 10,170 (2%) |
Number of high‐severity‐rated PIMs | |
0 | 304,523 (62%) |
1 | 129,588 (26%) |
2 | 43,739 (9%) |
3 | 12,213 (2%) |
4+ | 3908 (1%) |
Use of any PIM by side effect class | |
Sedatives | 156,384 (32%) |
Anticholinergic effects | 109,293 (22%) |
Causing orthostasis | 43,805 (9%) |
Causing bleeding | 14,744 (3%) |
Most commonly prescribed | |
Promethazine | 49,888 (10%) |
Diphenhydramine | 45,458 (9%) |
Propoxyphene | 41,786 (8%) |
Clonidine | 34,765 (7%) |
Amiodarone | 34,318 (7%) |
Lorazepam (>3 mg/day) | 25,147 (5%) |
Patient, Physician, and Hospital Factors Associated with PIMs
Patient, physician, and hospital characteristics were all associated with use of PIMs (Table 3). In univariate analyses, older patients were less likely to receive any class of PIM, and this difference was accentuated for HS‐PIMs. Women, American Indians, married people, and those not in managed care plans were slightly more likely to receive PIMs, whereas patients admitted with acute myocardial infarction or congestive heart failure were even more likely to receive PIMs (P < .0001 for all comparisons).
Patient characteristic | Any PIM n (row %) | Any high‐severity PIM n (row %) | Sedatives n (row %) | Anticholinergic effects n (row %) | Causing orthostasis n (row %) | Causing bleeding n (row %) |
---|---|---|---|---|---|---|
| ||||||
Overall | 239,771 (49%) | 189,448 (38%) | 156,384 (32%) | 109,293 (22%) | 43,805 (9%) | 14,744 (3%) |
Age group | ||||||
6574 years | 89,168 (53%) | 72,573 (43%) | 61,399 (36%) | 44,792 (27%) | 15,799 (9%) | 6655 (4%) |
7584 years | 100,787 (49%) | 79,595 (39%) | 65,034 (32%) | 45,121 (22%) | 18,519 (9%) | 5727 (3%) |
85+ years | 49,816 (42%) | 37,280 (31%) | 29,951 (25%) | 19,380 (16%) | 9487 (8%) | 2362 (2%) |
Sex | ||||||
Male | 100,824 (47%) | 79,535 (37%) | 63,591 (30%) | 42,754 (20%) | 17,885 (8%) | 5771 (3%) |
Female | 138,947 (49%) | 109,913 (39%) | 92,793 (33%) | 66,539 (24%) | 25,920 (9%) | 8973 (3%) |
Race | ||||||
White | 173,481 (49%) | 139,941 (40%) | 112,556 (32%) | 81,097 (23%) | 27,555 (8%) | 10,590 (3%) |
Black | 26,793 (51%) | 18,655 (36%) | 18,720 (36%) | 11,263 (21%) | 8925 (17%) | 1536 (3%) |
Hispanic | 8509 (47%) | 6370 (35%) | 5549 (31%) | 3505 (19%) | 2047 (11%) | 648 (4%) |
American Indian | 1091 (58%) | 849 (45%) | 818 (44%) | 563 (30%) | 190 (10%) | 76 (4%) |
Asian/Pacific Islander | 2386 (40%) | 1896 (32%) | 1420 (24%) | 1023 (17%) | 519 (9%) | 127 (2%) |
Other | 27,511 (43%) | 21,737 (34%) | 17,321 (27%) | 11,842 (18%) | 4569 (7%) | 1767 (3%) |
Marital status | ||||||
Married/partner | 96,874 (50%) | 77,803 (40%) | 63,303 (33%) | 45,042 (23%) | 16,765 (9%) | 5969 (3%) |
Widowed | 74,622 (48%) | 58,012 (37%) | 48,367 (31%) | 33,516 (22%) | 13,865 (9%) | 4354 (3%) |
Single/separated/divorced | 36,583 (48%) | 28,799 (38%) | 24,251 (32%) | 16,115 (21%) | 7229 (10%) | 2399 (3%) |
Other | 31,692 (46%) | 24,834 (36%) | 20,463 (30%) | 14,620 (21%) | 5946 (9%) | 2022 (3%) |
Primary diagnosis | ||||||
Pneumonia | 56,845 (46%) | 46,271 (38%) | 35,353 (29%) | 25,484 (21%) | 9184 (7%) | 4155 (3%) |
Heart failure | 56,460 (52%) | 42,231 (39%) | 34,340 (31%) | 22,093 (20%) | 10,117 (9%) | 1945 (2%) |
Acute MI | 43,046 (61%) | 37,849 (54%) | 32,560 (46%) | 25,568 (36%) | 4738 (7%) | 2549 (4%) |
Ischemic stroke | 25,763 (45%) | 17,613 (31%) | 18,500 (32%) | 8742 (15%) | 9644 (17%) | 1384 (2%) |
Chest pain | 20,655 (41%) | 16,363 (32%) | 13,536 (27%) | 10,520 (21%) | 3474 (7%) | 2027 (4%) |
COPD | 18,876 (42%) | 14,626 (33%) | 12,087 (27%) | 8096 (18%) | 3209 (7%) | 1139 (3%) |
Urinary tract infection | 18,126 (46%) | 14,495 (37%) | 10,008 (25%) | 8790 (22%) | 3439 (9%) | 1545 (4%) |
Payer type | ||||||
Nonmanaged care | 212,150 (49%) | 168,013 (39%) | 138,679 (32%) | 97,776 (23%) | 38,341 (9%) | 12,868 (3%) |
Managed care | 27,621 (44%) | 21,435 (34%) | 17,705 (28%) | 11,517 (18%) | 5464 (9%) | 1876 (3%) |
Attending physician specialty* | ||||||
Internal medicine (internist%) | 112,664 (47%) | 86,907 (36%) | 71,382 (30%) | 48,746 (20%) | 23,221 (10%) | 7086 (3%) |
Family/general medicine | 41,303 (45%) | 32,338 (36%) | 25,653 (28%) | 18,274 (20%) | 7660 (8%) | 2852 (3%) |
Cardiology | 48,485 (58%) | 40,752 (49%) | 34,859 (42%) | 25,792 (31%) | 5455 (7%) | 2542 (3%) |
Pulmonology | 10,231 (48%) | 8105 (38%) | 6746 (32%) | 4064 (19%) | 1739 (8%) | 574 (3%) |
Hospitalist | 7003 (47%) | 5443 (36%) | 4447 (30%) | 3179 (21%) | 1471 (10%) | 463 (3%) |
Nephrology | 4508 (55%) | 3388 (41%) | 3132 (38%) | 2054 (25%) | 1326 (16%) | 198 (2%) |
Neurology | 2420 (42%) | 1789 (31%) | 1625 (28%) | 851 (15%) | 699 (12%) | 174 (3%) |
Geriatrics | 1020 (33%) | 785 (25%) | 596 (19%) | 404 (13%) | 196 (6%) | 41 (1%) |
The HS‐PIM prescribing varied substantially by attending specialty (Fig. 2). Internists, family practitioners, and hospitalists all had similar median rates (33%), cardiologists had a higher median rate (48%), and geriatricians had a lower rate (24%). The most common PIM also differed by specialty: whereas promethazine was the most commonly prescribed drug across most specialties, nephrologists and neurologists used clonidine, pulmonologists used lorazepam, and cardiologists used diphenhydramine most often. Among the 8% of physicians who saw at least 50 patients, there was also great variation in each specialty (Fig. 2). Among internists and cardiologists who saw at least 50 patients, the high‐severity PIM usage rate ranged from 0% to more than 90%.
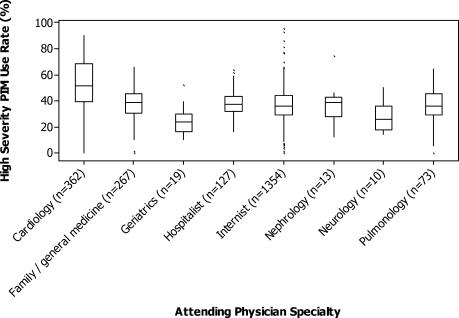
There was substantial variation in PIM usage among hospitals, most notably by region. The mean proportion of patients receiving PIMs ranged from 34% at hospitals in the Northeast to 55% at hospitals in the South (Table 4). Smaller hospitals and those in urban settings had slightly lower rates, as did those that had geriatricians on staff. The teaching status of the hospital had little effect. Variation at the individual hospital level was extreme (Fig. 3). Although half of all hospitals had rates between 43% and 58%, in 7 hospitals with more than 300 encounters each, PIMs were never prescribed for geriatric patients.
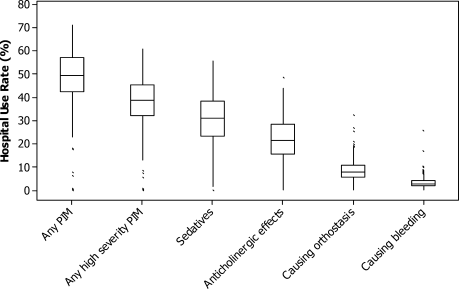
Hospitals Total = 384 n (%) | Patients N = 49,3971 n (%) | Any PIM Mean = 48.2 Mean (SD) | Any high‐severity PIM Mean = 38.7 Mean (SD) | Sedatives Mean = 30.2 Mean (SD) | Anticholinergic effects Mean = 21.5 Mean (SD) | Causing orthostasis Mean = 8.5 Mean (SD) | Causing bleeding Mean = 3.1 Mean (SD) | |
---|---|---|---|---|---|---|---|---|
| ||||||||
Hospital region | *** | *** | *** | *** | *** | ** | ||
Midwest | 76 (20%) | 95,791 (19%) | 38.8 (19.7) | 30.0 (16.4) | 24.3 (13.8) | 15.1 (9.9) | 6.9 (6.3) | 3.1 (2.3) |
Northeast | 47 (12%) | 79,138 (16%) | 34.1 (12.6) | 26.2 (11.2) | 19.0 (9.2) | 13.5 (8.1) | 4.9 (2.3) | 2.1 (1.6) |
South | 199 (52%) | 260,870 (53%) | 54.5 (10.1) | 42.7 (9.6) | 36.0 (10.8) | 26.4 (8.6) | 10.4 (4.6) | 3.6 (2.5) |
West | 62 (16%) | 58,172 (12%) | 45.8 (8.1) | 37.4 (7.1) | 27.3 (7.7) | 19.5 (5.7) | 7.4 (4.8) | 2.7 (1.3) |
Teaching status | ||||||||
Nonteaching | 297 (77%) | 324,948 (66%) | 47.3 (14.6) | 36.9 (12.3) | 29.8 (12.0) | 21.3 (9.9) | 8.7 (5.4) | 3.3 (2.4) |
Teaching | 87 (23%) | 169,023 (34%) | 48.2 (16.0) | 38.8 (14.2) | 31.6 (14.5) | 22.1 (10.2) | 7.8 (4.4) | 2.7 (1.5) |
Staffed beds | * | ** | ||||||
22200 | 143 (37%) | 80,741 (16%) | 45.5 (16.9) | 35.2 (14.6) | 27.5 (14.0) | 20.1 (10.3) | 8.0 (6.2) | 3.5 (3.1) |
200400 | 137 (36%) | 177,286 (36%) | 47.7 (14.2) | 37.8 (12.0) | 30.5 (11.6) | 22.0 (10.0) | 8.4 (4.7) | 3.0 (1.6) |
400+ | 104 (27%) | 235944 (48%) | 50.1 (12.4) | 39.6 (10.6) | 33.5 (10.9) | 22.7 (9.3) | 9.3 (4.2) | 2.9 (1.4) |
Population serviced | * | * | ** | |||||
Rural | 119 (31%) | 102,799 (21%) | 48.4 (13.0) | 38.3 (10.6) | 29.2 (11.0) | 23.2 (9.3) | 7.5 (4.0) | 3.7 (3.0) |
Urban | 265 (69%) | 391,172 (79%) | 47.1 (15.7) | 36.9 (13.7) | 30.6 (13.2) | 20.7 (10.2) | 9.0 (5.6) | 2.9 (1.8) |
Geriatrician presence | ||||||||
No | 340 (89%) | 409,281 (83%) | 47.7 (15.3) | 37.6 (13.0) | 30.3 (12.8) | 21.7 (10.0) | 8.4 (5.3) | 3.2 (2.3) |
Yes | 44 (11%) | 84,690 (17%) | 45.8 (11.4) | 35.5 (10.6) | 29.4 (10.8) | 19.6 (9.4) | 9.3 (4.3) | 2.9 (1.6) |
Multivariable Model
In a multivariable logit model that included all patient, hospital, and physician characteristics and that accounted for clustering at the hospital, physician, and diagnosis levels, several characteristics were associated with HS‐PIM prescribing (Table 5). By far the most important predictor of use was hospital region. Compared with patients at hospitals in the Midwest, patients in the South (OR 1.63, 95% CI 1.591.67) and West (OR 1.43, 95% CI 1.381.47) were more likely and those in the Northeast were less likely (OR 0.85, 95% CI 0.830.88) to receive HS‐PIMs. Larger hospitals had higher HS‐PIM rates than smaller ones, but teaching status and rural or urban setting were not associated with HS‐PIM prescribing. The presence of geriatricians in a hospital was also associated with lower HS‐PIM prescribing for the entire hospital.
Effect (reference) | Odds ratio | 95% Confidence limits | |
---|---|---|---|
Age | |||
6574 years | 1.00 | ||
7584 years | 0.83 | 0.82 | 0.84 |
85+ years | 0.59 | 0.58 | 0.61 |
Sex | |||
Female | 1.00 | ||
Male | 0.85 | 0.83 | 0.86 |
Race | |||
White | 1.00 | ||
Black | 0.78 | 0.76 | 0.80 |
Hispanic | 0.84 | 0.81 | 0.87 |
American Indian | 0.97 | 0.88 | 1.07 |
Asian/Pacific Islander | 0.74 | 0.70 | 0.79 |
Other | 0.94 | 0.92 | 0.97 |
Marital Status | |||
Married/partner | 1.00 | ||
Single/separated/divorced | 0.96 | 0.94 | 0.98 |
Widowed | 0.96 | 0.95 | 0.98 |
Other | 0.93 | 0.90 | 0.95 |
Primary diagnosis | |||
Pneumonia | 1.00 | ||
COPD | 0.83 | 0.81 | 0.85 |
Heart failure | 1.14 | 1.12 | 1.16 |
Ischemic stroke | 0.84 | 0.82 | 0.86 |
Acute MI | 1.95 | 1.90 | 2.01 |
Urinary tract infection | 1.06 | 1.03 | 1.09 |
Chest pain | 0.87 | 0.84 | 0.89 |
Comorbidities (yes or no) | |||
Hypertension | 0.98 | 0.97 | 0.99 |
Diabetes | 0.98 | 0.97 | 1.00 |
Chronic lung disease | 1.11 | 1.10 | 1.13 |
Fluid and electrolyte disorders | 1.26 | 1.24 | 1.27 |
Anemia deficiencies | 1.17 | 1.15 | 1.18 |
Congestive heart failure | 1.34 | 1.32 | 1.37 |
Hypothyroidism | 1.13 | 1.11 | 1.15 |
Peripheral vascular disease | 1.09 | 1.06 | 1.11 |
Depression | 1.38 | 1.35 | 1.41 |
Neurological disorders | 0.89 | 0.87 | 0.91 |
Renal failure | 1.23 | 1.20 | 1.26 |
Obesity | 1.11 | 1.08 | 1.14 |
Payer type | |||
Managed care | 1.00 | ||
Not managed care | 1.04 | 1.02 | 1.06 |
Attending physician specialty | |||
Internal medicine | 1.00 | ||
Cardiology | 1.32 | 1.28 | 1.36 |
Family/general medicine | 0.99 | 0.97 | 1.01 |
Geriatrics | 0.69 | 0.61 | 0.78 |
Hospitalist | 0.90 | 0.84 | 0.96 |
Nephrology | 1.02 | 0.96 | 1.08 |
Neurology | 0.93 | 0.86 | 1.00 |
Pulmonology | 1.10 | 1.05 | 1.15 |
Setting | |||
Rural | 1.00 | ||
Urban | 1.02 | 1.00 | 1.05 |
Teaching status | |||
Nonteaching | 1.00 | ||
Teaching | 1.01 | 0.98 | 1.03 |
Number of beds | |||
22200 | 1.00 | ||
200400 | 1.08 | 1.05 | 1.11 |
400+ | 1.12 | 1.09 | 1.16 |
Region | |||
Midwest | 1.00 | ||
Northeast | 0.85 | 0.83 | 0.88 |
South | 1.63 | 1.59 | 1.67 |
West | 1.43 | 1.38 | 1.47 |
Geriatrician presence | |||
No | 1.00 | ||
Yes | 0.93 | 0.90 | 0.95 |
Physician specialty was also important. Adjusting for diagnosis attenuated some of this association, but compared with internists, cardiologists (OR 1.32, 95% CI 1.281.36) and pulmonologists (OR 1.10, 95% CI 1.051.15) were still more likely, hospitalists (OR 0.90, 95% CI 0.840.96) were less likely, and geriatricians (0.69, 95% CI 0.610.78) were least likely to prescribe any HS‐PIM.
Patient factors were also associated with HS‐PIM use. Compared with patients age 6574 years, patients older than 85 years were much less likely to receive an HS‐PIM (OR 0.59, CI 0.580.61), as to a lesser extent were nonwhites compared with whites and unmarried people compared with those who were married. Compared with patients with pneumonia, those with COPD, stroke, or chest pain were less likely and those with myocardial infarction and congestive heart failure were more likely to receive HS‐PIMs. Patients with a secondary diagnosis of depression were also at high risk (OR 1.38, CI 1.351.41).
DISCUSSION
Although Americans age 65 years and older make up less than 15% of the U.S. population, they consume about one third of all prescription drugs20 and account for one third of all hospital admissions.21 Using the Beers list, numerous studies have documented high rates of potentially inappropriate prescribing for community‐dwelling elderly and nursing home patients and, in some studies, an attendant risk of falling,2224 hip fracture,25, 26 hospitalization,13 or death.14 Applying these same criteria to a large sample of medical inpatients, we found that almost half received a potentially inappropriate drug, most of high severity. Moreover, the PIM prescribing rate varied substantially by region, hospital, and attending physician specialty. Although the use of PIMs was associated with patient age, comorbidities, and primary diagnosis, these patient factors explained only a small portion of the variation in prescribing practices across groups of physicians and hospitals.
Using consensus criteria, Beers originally found that 40% of the residents in 12 nursing homes received at least 1 PIM,8 and studies of community‐dwelling elderly demonstrated rates of 21% to 37%, with little change over time.6, 27, 28 Several small studies have examined inpatient prescribing.16, 17, 29, 30 The largest17 found that only 15% of elderly Italian inpatients received a PIM. Our finding, that 49% of inpatients had received at least 1 PIM, may partially reflect the high prevalence of use among elderly US patients in nursing homes and the community.
Regional variation has been demonstrated for ambulatory patients in the US6 and Europe.31 Zhan et al. found slightly higher rates of PIM use in the Midwest and the South (23%) than in the Northeast and the West (19%). Variation in Europe was greater, with 41% of patients in the Czech Republic versus 5.8% of patients in Denmark receiving at least 1 PIM. We found that region was the strongest predictor of in‐hospital HS‐PIM use, with patients in the South most likely and patients in the Northeast least likely to receive HS‐PIMs. This variation persisted even after adjusting for differences in other patient and hospital factors, suggesting that local custom played a large role in the decision to prescribe HS‐PIMs. Moreover, because outpatient rates are more uniform, these large differences seem limited to inpatient practice.
Patient factors have also been examined. Advanced age was associated with decreased PIM use in some studies17, 28, 31 but not in others.6, 27 We found increasing age to be strongly associated with decreased PIM use, suggesting that in the hospital, at least, doctors take care to avoid prescribing certain drugs to the frail elderly. Women appear to be consistently at higher risk than men,6, 27, 28, 31 and white patients are more at risk than those of other races.6 Our finding that certain diagnoses were associated with higher or lower rates has not been reported previously. The lower rates associated with stroke and COPD suggest that prescribers were aware that these patients were at increased risk of delirium and respiratory depression. The higher rates associated with myocardial infarction may have to do with the use of standardized order sets (eg, cath lab orders) that do not consider the age of the patient going for the procedure.
Admission to a geriatric service32 and intervention by a clinical pharmacist33 have been shown to decrease PIM prescribing at discharge. We noted that patients cared for by a geriatrician had the lowest rates of PIM prescribing during hospitalization as well and that hospitals with geriatricians had lower rates overall, possibly demonstrating that geriatricians had a ripple effect on their colleagues. Hospitalists also had lower rates than internists, supporting the notion that hospitalists provide higher‐quality inpatient care.
Our study had some important limitations. First, we only had access to inpatient administrative records. Thus, we could not identify which medications were continued from home and which were begun in the hospital, nor could we know the indications for which specific drugs were prescribed or who prescribed them. Based on published outpatient rates, however, we could assume that many of the drugs were started in the hospital and that others could have been discontinued but were not. Second, the Beers list was developed by the modified Delphi method; there was little empirical evidence of the danger of specific drugs, although some classes, such as benzodiazepines, opiates and digoxin, have been associated with inpatient falls.18, 3436 Furthermore, our administrative database did not allow us to balance the risks and benefits for particular patients; hence, the medications were only potentially inappropriate, and our study did not address the consequences of such prescribing. Although some of these drugs may be appropriate under certain circumstances, it is unlikely that these circumstances would vary by 60% across geographic regions or that internists would encounter these circumstances more often than do hospitalists. Thus, although we could not identify specific patients who received inappropriate medications, we did identify certain hospitals and even whole regions of the country in which the rate of inappropriate prescribing was high. Third, the Beers list, which was developed for outpatient use, may be less relevant in the inpatient setting. However, given that inpatients have more organ dysfunction and are at higher risk of delirium and falls, it may actually be more applicable to hospitalized patients. We similarly did not distinguish between single and multiple doses because the Beers list does not make such a distinction, and there is no empirical evidence that a single dose is safe. Indeed, patients are often at highest risk of falls immediately after initiation of therapy.3739 We did, however, exclude drugs such as laxatives, which may be appropriate for brief inpatient use but not for chronic use.
Our study also had a number of strengths. The large sample size, representing approximately 5% of annual inpatient admissions in the US over 2 years, offered an instructive look at the recent prescribing patterns of thousands of US physicians. We were able to identify many patient, physician, and hospital factors associated with PIM prescribing that have not previously been reported. Some of these factors, such as advanced age and comorbid diagnoses, suggest that physicians do tailor their treatment to individual patients. Nevertheless, patient factors accounted for only a small portion of the variation in prescribing. The largest variation, associated with regional, hospital, and physician factors, highlights the opportunity for improvement.
At the same time, our findings are encouraging for 2 reasons. First, most inappropriate prescribing involved only a handful of medications, so small changes in prescribing patterns could have a tremendous impact. Second, observing the practice of individual physicians and hospitals reveals what is possible. We found that in most specialties there were physicians who rarely or never used PIMs. We also found 7 hospitals, each with at least 300 cases, where no PIMs were ever prescribed.
Where should hospitals focus their efforts to prevent inappropriate prescribing? Our data highlight the complexity of the problem, which seems daunting. PIM prescribing is spread across all specialties, including geriatrics, and although cardiologists had the highest rate of prescribing, internists, who were more numerous, accounted for a much higher overall number of potentially inappropriate prescriptions. It would be instructive to study the 7 hospitals where PIMs were never prescribed or to interview those physicians who never prescribed PIMs, but the anonymous nature of our data would not allow for this. However, our data do suggest some directions. First, hospitals should become aware of their own rates of PIM use because measurement is the first step in quality improvement. Next, hospitals should focus efforts on reducing the use of the most common drugs. Eliminating just 3 drugs promethazine, diphenhydramine, and propoxyphenewould reduce the use of PIMs in 24% of elderly patients. Enlisting hospital pharmacists and electronic health records and reviewing standard order sets for elderly patients are potentially effective strategies. Finally, increasing the presence of geriatricians and hospitalists would be expected to have a modest impact.
In a representative sample of elderly inpatients, we found that almost half received a potentially inappropriate medication and that the rate of inappropriate prescribing varied widely among doctors and hospitals. Additional research is needed to distinguish which of the Beers drugs are most harmful and which patients are at highest risk. Research should also focus on understanding differences in prescribing patterns, perhaps by studying the outliers at both ends of the quality spectrum, and on techniques to minimize non‐patient‐centered variation.
- Explicit criteria for determining inappropriate medication use in nursing home residents. UCLA Division of Geriatric Medicine.Arch Intern Med.1991;151:1825–1832. , , , , , .
- Explicit criteria for determining potentially inappropriate medication use by the elderly. An update.Arch Intern Med.1997;157:1531–1536. .
- Updating the Beers criteria for potentially inappropriate medication use in older adults: results of a US consensus panel of experts.Arch Intern Med.2003;163:2716–2724. , , , , , .
- National Committee on Quality Assurance. Drugs to be Avoided in the Elderly. Available at: http://www.ncqa.org/Programs/HEDIS/2006/Volume2/NDC/DAE_06.xls. Accessed November 20,2006.
- Inappropriate prescribing for elderly Americans in a large outpatient population.Arch Intern Med.2004;164:1621–1625. , , , et al.
- Potentially inappropriate medication use in the community‐dwelling elderly: findings from the 1996 Medical Expenditure Panel Survey.JAMA.2001;286:2823–2829. , , , et al.
- Prescribing potentially inappropriate psychotropic medications to the ambulatory elderly.Arch Intern Med.2000;160:2825–2831. , .
- Inappropriate medication prescribing in skilled‐nursing facilities.Ann Intern Med.1992;117:684–689. , , , et al.
- Adverse outcomes associated with inappropriate drug use in nursing homes.Ann Pharmacother.2005;39:405–411. , , , et al.
- Inappropriate medication administration to the acutely ill elderly: a nationwide emergency department study, 1992–2000.J Am Geriatr Soc.2004;52:1847–1855. , , .
- Appropriateness of medication selection for older persons in an urban academic emergency department.Acad Emerg Med.1999;6:1232–1242. , , , et al.
- Use of the Beers criteria to predict adverse drug reactions among first‐visit elderly outpatients.Pharmacotherapy.2005;25:831–838. , , , , , .
- The association of inappropriate drug use with hospitalisation and mortality: a population‐based study of the very old.Drugs Aging.2005;22(1):69–82. , , .
- Hospitalization and death associated with potentially inappropriate medication prescriptions among elderly nursing home residents.Arch Intern Med.2005;165(1):68–74. , , , , .
- Potentially inappropriate prescribing for geriatric inpatients: an acute care of the elderly unit compared to a general medicine service.Consult Pharm.2003;18(1):37–42, 47–39. , , .
- Inappropriate medication use among frail elderly inpatients.Ann Pharmacother.2004;38(1):9–14. , , , et al.
- Inappropriate medication use among hospitalized older adults in Italy: results from the Italian Group of Pharmacoepidemiology in the Elderly.Eur J Clin Pharmacol.2003;59(2):157–162. , , , , , .
- Guided prescription of psychotropic medications for geriatric inpatients.Arch Intern Med.2005;165:802–807. , , , , , .
- Comorbidity measures for use with administrative data.Med Care.1998;36(1):8–27. , , , .
- Inadequate prescription‐drug coverage for Medicare enrollees—a call to action.N Engl J Med.1999;340:722–728. , .
- National and regional estimates on hospital use for all patients from the HCUP Nationwide Inpatient Sample (NIS). Agency for Healthcare Research and Quality (AHRQ). Available at: http://hcupnet.ahrq.gov/HCUPnet.jsp. Accessed October 12,2006.
- Drugs and falls in community‐dwelling older people: a national veterans study.Clin Ther.2006;28:619–630. , , , , , .
- Psychotropic medications and risk for falls among community‐dwelling frail older people: an observational study.J Gerontol A Biol Sci Med Sci.2005;60:622–626. , , , , , .
- Drugs and falls in older people: a systematic review and meta‐analysis: I. Psychotropic drugs.J Am Geriatr Soc.1999;47(1):30–39. , , .
- Propoxyphene use and risk for hip fractures in older adults.Am J Geriatr Pharmacother.2006;4:219–226. , , .
- Central nervous system active medications and risk for fractures in older women.Arch Intern Med.2003;163:949–957. , , , et al.
- Potentially inappropriate medication use by elderly persons in U.S. Health Maintenance Organizations, 2000–2001.J Am Geriatr Soc.2005;53:227–232. , , , et al.
- Inappropriate medication prescribing for elderly ambulatory care patients.Arch Intern Med.2004;164:305–312. .
- Adverse drug reactions in an elderly hospitalised population: inappropriate prescription is a leading cause.Drugs Aging.2005;22:767–777. , , .
- Use of inappropriate medications and their prognostic significance among in‐hospital and nursing home patients with and without dementia in Finland.Drugs Aging.2006;23:333–343. , , , , .
- Potentially inappropriate medication use among elderly home care patients in Europe.JAMA.2005;293:1348–1358. , , , et al.
- Impact of hospitalisation in an acute medical geriatric unit on potentially inappropriate medication use.Drugs Aging.2006;23(1):49–59. , , , , .
- Pharmacists and their effectiveness in ensuring the appropriateness of the chronic medication regimens of geriatric inpatients.Consult Pharm.2004;19:432–436. , .
- Benzodiazepines with different half‐life and falling in a hospitalized population: the GIFA study. Gruppo Italiano di Farmacovigilanza nell'Anziano.J Clin Epidemiol.2000;53:1222–1229. , , , , , .
- Relationship between the administration of selected medications and falls in hospitalized elderly patients.Ann Pharmacother.1995;29:354–358. , .
- The use of sedative/hypnotic medication and its correlation with falling down in the hospital.Sleep.1996;19:698–701. .
- Benzodiazepine use and hip fractures in the elderly: who is at greatest risk?Arch Intern Med.2004;164:1567–1572. , , , et al.
- Hazardous benzodiazepine regimens in the elderly: effects of half‐life, dosage, and duration on risk of hip fracture.Am J Psychiatry.2001;158:892–898. , , , , .
- A 5‐year prospective assessment of the risk associated with individual benzodiazepines and doses in new elderly users.J Am Geriatr Soc.2005;53:233–241. , , , , .
- Explicit criteria for determining inappropriate medication use in nursing home residents. UCLA Division of Geriatric Medicine.Arch Intern Med.1991;151:1825–1832. , , , , , .
- Explicit criteria for determining potentially inappropriate medication use by the elderly. An update.Arch Intern Med.1997;157:1531–1536. .
- Updating the Beers criteria for potentially inappropriate medication use in older adults: results of a US consensus panel of experts.Arch Intern Med.2003;163:2716–2724. , , , , , .
- National Committee on Quality Assurance. Drugs to be Avoided in the Elderly. Available at: http://www.ncqa.org/Programs/HEDIS/2006/Volume2/NDC/DAE_06.xls. Accessed November 20,2006.
- Inappropriate prescribing for elderly Americans in a large outpatient population.Arch Intern Med.2004;164:1621–1625. , , , et al.
- Potentially inappropriate medication use in the community‐dwelling elderly: findings from the 1996 Medical Expenditure Panel Survey.JAMA.2001;286:2823–2829. , , , et al.
- Prescribing potentially inappropriate psychotropic medications to the ambulatory elderly.Arch Intern Med.2000;160:2825–2831. , .
- Inappropriate medication prescribing in skilled‐nursing facilities.Ann Intern Med.1992;117:684–689. , , , et al.
- Adverse outcomes associated with inappropriate drug use in nursing homes.Ann Pharmacother.2005;39:405–411. , , , et al.
- Inappropriate medication administration to the acutely ill elderly: a nationwide emergency department study, 1992–2000.J Am Geriatr Soc.2004;52:1847–1855. , , .
- Appropriateness of medication selection for older persons in an urban academic emergency department.Acad Emerg Med.1999;6:1232–1242. , , , et al.
- Use of the Beers criteria to predict adverse drug reactions among first‐visit elderly outpatients.Pharmacotherapy.2005;25:831–838. , , , , , .
- The association of inappropriate drug use with hospitalisation and mortality: a population‐based study of the very old.Drugs Aging.2005;22(1):69–82. , , .
- Hospitalization and death associated with potentially inappropriate medication prescriptions among elderly nursing home residents.Arch Intern Med.2005;165(1):68–74. , , , , .
- Potentially inappropriate prescribing for geriatric inpatients: an acute care of the elderly unit compared to a general medicine service.Consult Pharm.2003;18(1):37–42, 47–39. , , .
- Inappropriate medication use among frail elderly inpatients.Ann Pharmacother.2004;38(1):9–14. , , , et al.
- Inappropriate medication use among hospitalized older adults in Italy: results from the Italian Group of Pharmacoepidemiology in the Elderly.Eur J Clin Pharmacol.2003;59(2):157–162. , , , , , .
- Guided prescription of psychotropic medications for geriatric inpatients.Arch Intern Med.2005;165:802–807. , , , , , .
- Comorbidity measures for use with administrative data.Med Care.1998;36(1):8–27. , , , .
- Inadequate prescription‐drug coverage for Medicare enrollees—a call to action.N Engl J Med.1999;340:722–728. , .
- National and regional estimates on hospital use for all patients from the HCUP Nationwide Inpatient Sample (NIS). Agency for Healthcare Research and Quality (AHRQ). Available at: http://hcupnet.ahrq.gov/HCUPnet.jsp. Accessed October 12,2006.
- Drugs and falls in community‐dwelling older people: a national veterans study.Clin Ther.2006;28:619–630. , , , , , .
- Psychotropic medications and risk for falls among community‐dwelling frail older people: an observational study.J Gerontol A Biol Sci Med Sci.2005;60:622–626. , , , , , .
- Drugs and falls in older people: a systematic review and meta‐analysis: I. Psychotropic drugs.J Am Geriatr Soc.1999;47(1):30–39. , , .
- Propoxyphene use and risk for hip fractures in older adults.Am J Geriatr Pharmacother.2006;4:219–226. , , .
- Central nervous system active medications and risk for fractures in older women.Arch Intern Med.2003;163:949–957. , , , et al.
- Potentially inappropriate medication use by elderly persons in U.S. Health Maintenance Organizations, 2000–2001.J Am Geriatr Soc.2005;53:227–232. , , , et al.
- Inappropriate medication prescribing for elderly ambulatory care patients.Arch Intern Med.2004;164:305–312. .
- Adverse drug reactions in an elderly hospitalised population: inappropriate prescription is a leading cause.Drugs Aging.2005;22:767–777. , , .
- Use of inappropriate medications and their prognostic significance among in‐hospital and nursing home patients with and without dementia in Finland.Drugs Aging.2006;23:333–343. , , , , .
- Potentially inappropriate medication use among elderly home care patients in Europe.JAMA.2005;293:1348–1358. , , , et al.
- Impact of hospitalisation in an acute medical geriatric unit on potentially inappropriate medication use.Drugs Aging.2006;23(1):49–59. , , , , .
- Pharmacists and their effectiveness in ensuring the appropriateness of the chronic medication regimens of geriatric inpatients.Consult Pharm.2004;19:432–436. , .
- Benzodiazepines with different half‐life and falling in a hospitalized population: the GIFA study. Gruppo Italiano di Farmacovigilanza nell'Anziano.J Clin Epidemiol.2000;53:1222–1229. , , , , , .
- Relationship between the administration of selected medications and falls in hospitalized elderly patients.Ann Pharmacother.1995;29:354–358. , .
- The use of sedative/hypnotic medication and its correlation with falling down in the hospital.Sleep.1996;19:698–701. .
- Benzodiazepine use and hip fractures in the elderly: who is at greatest risk?Arch Intern Med.2004;164:1567–1572. , , , et al.
- Hazardous benzodiazepine regimens in the elderly: effects of half‐life, dosage, and duration on risk of hip fracture.Am J Psychiatry.2001;158:892–898. , , , , .
- A 5‐year prospective assessment of the risk associated with individual benzodiazepines and doses in new elderly users.J Am Geriatr Soc.2005;53:233–241. , , , , .
Copyright © 2008 Society of Hospital Medicine
Innovative Cardiac Examination Curriculum
Despite impressive advances in cardiac diagnostic technology, cardiac examination (CE) remains an essential skill for screening for abnormal sounds, for evaluating cardiovascular system function, and for guiding further diagnostic testing.16
In practice, these benefits may be attenuated if CE skills are inadequate. Numerous studies have documented substantial CE deficiencies among physicians at various points in their careers from medical school to practice.79 In 1 study, residents' CE mistakes accounted for one‐third of all physical diagnostic errors.10 When murmurs are detected, physicians will often reflexively order an echocardiogram and refer to a cardiologist, regardless of the cost or indication. As a consequence, echocardiography use is rising faster than the aging population or the incidence of cardiac pathological conditions would explain.11 Because cost‐effective medicine depends on the appropriate application of clinical skills like CE, the loss of these skills is a major shortcoming.1215
The reasons for the decline in physicians' CE skills are numerous. High reliance on ordering diagnostic tests,16 conducting teaching rounds away from the bedside,17, 18 time constraints during residency,16, 19 and declining CE skills of faculty members themselves7 all may contribute to the diminished CE skills of residents. Residents, who themselves identify abnormal heart sounds at alarmingly low rates, play an ever‐increasing role in medical students' instruction,7, 9 exacerbating the problem.
Responding to growing concerns over patient safety and quality of care,16, 20 public and professional organizations have called for renewed emphasis on teaching and evaluating clinical skills.21 For example, the American Board of Internal Medicine has added a physical diagnosis component to its recertification program.22 The Accreditation Council for Graduate Medical Education (ACGME) describes general competencies for residents, including patient care that should include proper physical examination skills.23 Although mandating uniform standards is a welcome change for improving CE competence, the challenge remains for medical school deans and program directors to fit structured physical examination skills training into an already crowded curriculum.16, 24 Moreover, the impact of these efforts to improve CE is uncertain because programs lack an objective measure of CE competence.
The CE training is itself a challenge: sight, sound, and touch all contribute to the clinical impression. For this reason, it is difficult to teach away from the bedside. Unlike pulmonary examination, for which a diagnosis is best made by listening, cardiac auscultation is only one (frequently overemphasized) aspect of CE.25 Medical knowledge of cardiac anatomy and physiology, visualization of cardiovascular findings, and integration of auditory and visual findings are all components of accurate CE.7 Traditionally, CE was taught through direct experience with patients at the bedside under the supervision of seasoned clinicians. However, exposure and learning from good teaching patients has waned. Audiotapes, heart sound simulators, mannequins, and other computer‐based interventions have been used as surrogates, but none has been widely adopted.26, 27 The best practice for teaching CE is not known.
To help to improve CE education during residency, we implemented and evaluated a novel Web‐based CE curriculum that emphasized 4 aspects of CE: cardiovascular anatomy and physiology, auditory skills, visual skills, and integration of auscultatory and visual findings. Our hypothesis was that this new curriculum would improve learning of CE skills, that residents would retain what they learn, and that this curriculum would be better than conventional education in teaching CE skills.
METHODS
Study Participants, Site, and Design
Internal medicine (IM) and family medicine (FM) interns (R1s, n = 59) from university‐ and community‐based residency programs, respectively, participated in this controlled trial of an educational intervention to teach CE.
The intervention group consisted of 26 IM and 8 FM interns at the beginning of the academic year in June 2003. To establish baseline scores, all interns took a 50‐question multimedia test of CE competency described previously.7, 28, 29 Subsequently, all interns completed a required 4‐week cardiology ward rotation. During this rotation, they were instructed to complete a Web‐based CE tutorial with accompanying worksheet and to attend 3 one‐hour sessions with a hospitalist instructor. Their schedules were arranged to allow for this educational time. During the third meeting with the instructor, interns were tested again to establish posttraining scores. Finally, at the end of the academic year, interns were tested once again to establish retention scores.
The control group consisted of 25 first‐year IM residents who were tested at the end of their academic year in June 2003. These test scores served as historical controls for interns who had just completed their first year of residency and who had received standard ward rotation without incorporated Web‐based training in CE. Interns from both groups had many opportunities for one‐on‐one instruction in CE because each intern was assigned for the cardiology rotation to a private practice cardiology attending. Figure 1 outlines the number of IM and FM interns eligible and the number actually tested at each stage of the study.
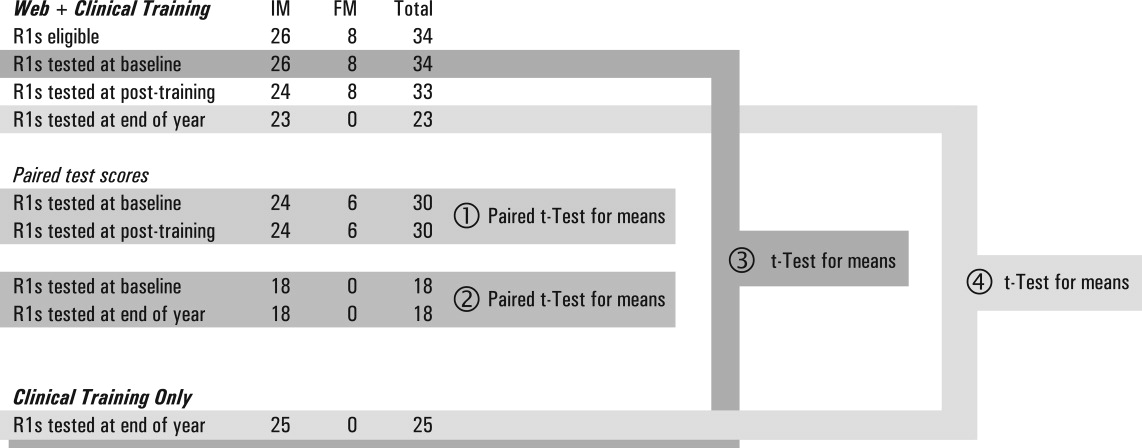
Educational Intervention
The CE curriculum consisted of a Web‐based program and 3 tutored sessions. The program used virtual patientsaudiovisual recordings of actual patientscombined with computer graphic animations and text to teach cardiac anatomy, hemodynamics, pathophysiology, and visual and auditory findings.30 This multimedia program was interactive and allowed comparisons to normal or to similar lesions. The content included cardiac findings identified as important by a survey of IM residency program directors,8 as well as ACGME training requirements for IM residents23 and cardiology fellows.31 Table 1 outlines the content of the Web‐based curriculum.
1. Frontal anatomy of heart, lungs, and vessels with: |
a. Interactive illustrations allowing depiction of individual structures |
b. Separate cartoons of anatomy of the right heart, left heart, and entire heart |
c. Correlation with borders forming regions on chest X‐ray |
2. Interactive phases of the cardiac cycle including: |
a. Phonocardiogram of normal heart sounds (S1, S2) |
b. ECG recording |
c. Left heart (aortic, left atrial, and left ventricular) pressures |
d. Right heart (pulmonary artery, right atrial, and right ventricular) pressures |
e. Animations of the left heart. |
3. Physiological splitting of S2 with: |
a. Phonocardiogram of normal heart sounds |
b. ECG tracing |
c. Left heart (aortic, left atrial, and left ventricular) pressures |
d. Right heart (pulmonary artery, right atrial, and right ventricular) pressures |
e. Interactive animations of the heart and lungs with respiration |
4. Patients with aortic regurgitation (AR) |
a. Integrating pulse with sounds and murmurs |
b. Acute severe AR |
Recognizing Quincke's pulse |
c. Austin Flint murmur |
Differentiating it from the pericardial rub |
d. Hemodynamics of chronic and acute AR and comparisons |
e. Well‐tolerated AR |
5. Patient with aortic stenosis (AS) |
a. Integrating pulse with sounds and murmurs |
Comparison with HCM |
b. Interactive descriptions of hemodynamics and flow |
6. Patients with mitral regurgitation (MR) |
a. Chronic MR |
b. Hemodynamics and comparisons of clinical findings for: |
i. Normal |
ii. Mitral valve prolapse (MVP) |
iii. Acute MR |
iv. Compensated MR |
c. Acute MR |
7. Patients with mitral stenosis (MS) |
a. Introduction: integrating inspection and auscultation |
b. Compare sounds: opening snap, split S2, S3 |
c. Severe MS: interactive comparison of sounds at apex and base |
d. Hemodynamic effects of heart rate |
This training was designed for typical conditions of residency training programs: studentteacher contact time was limited to three 1‐hour sessions; the instructor (J.K.) was an internist hospitalist (trained and facile in the use of the program), not a cardiologist; and self‐paced study was Web‐based to allow access at all hours at the hospital or at home. In their first session, at the beginning of the cardiology block, interns were introduced to the Web site and given a 1‐page homework assignment that corresponded to the Web‐based content (Table 2). During the second session, in the middle of the 4‐week block, a group discussion was held with the Web‐based program, in which the interns asked questions and reviewed their worksheet answers and program as needed with the hospitalist. During the third session, at the end of the block, questions were reviewed, and the interns took the posttraining test.
In preparation for the cardiology heart sounds module during the cardiology block at LBMMC, please answer the following questions and bring the completed questionnaire with you. The correct responses to these questions as well as the underlying mechanisms can be found in the Heart Sounds Tutorial ( |
---|
1. Which cardiac chamber is farthest from the anterior chest wall? __________ |
2. Which cardiac chamber is closest to the left sternal border? ___________ |
3. Are the mitral and aortic valves ever closed at the same time? ____________ |
4. Why does inspiratory lung inflation delay the pulmonic second sound? ____________ |
5. Three or more murmurs of different origin can be heard in aortic regurgitation. What is their timing (within the cardiac cycle) and causation? ____________ |
6. How do you elicit Quincke's pulse? ____________ |
7. Is arterial pulse pressure greater in acute or chronic aortic regurgitation? ____________ |
8. How does the severity of aortic regurgitation correlate with duration of the early diastolic murmur? ____________ |
9. Splitting of the first sound heard with the stethoscope diaphragm in a patient with aortic stenosis is caused by? ____________ |
10. What effect does the severity have on the duration of the murmur of aortic stenosis? ____________ |
11. Is the murmur of aortic stenosis ever holosystolic? ____________ |
12. Is the duration of the murmur of acute mitral regurgitation shorter or longer than that of chronic mitral regurgitation? ____________ |
13. What causes the third heart sound (S3) in mitral regurgitation? ____________ |
14. Why is the jugular venous a‐wave often prominent in mitral stenosis? ____________ |
15. Which heart sound is loudest in mitral stenosis?Why? ____________ |
16. What causes the split sound heard in mitral stenosis? ____________ |
17. Where (on the precordium) would you hear the murmur of mitral stenosis ____________ |
18. How do postural maneuvers affect the heart sounds and murmur in mitral prolapse? ____________ |
19. A 3‐phase friction rub can be confused with the 3‐murmur auditory complex in which valvar lesion? ____________ |
20. What are some causes of third heart sounds that do not imply poor ventricular function? ____________ |
21. Is a fourth heart sound usually best heard at the base (□Yes □No) or the apex (□Yes □No)? |
Evaluation
To evaluate what the R1 intervention group learned, we tested them at baseline, during internship orientation; in posttraining, at the end of their cardiology rotation; and for retention, at the end of their internship year. To evaluate what the controls learned, we tested them at the end of their internship year. The evaluation included a brief survey and the previously validated CE Test.7, 28, 29 Test scores did not carry academic consequences.
For the survey, we asked participants whether they had some prior training in CE and how many hours they estimated having spent learning CE skills during this study with a teacher or in a course. Using a 5‐point Likert scale, they self‐rated their interest and confidence in their own CE skills.
The CE Test is a 50‐question interactive multimedia program that evaluates CE competency using recordings from actual patients. For the CE Test, an overall score (maximum 100 points) and scores for 4 subcategories (expressed as percentages)knowledge of cardiac physiology (interpretation of pressures, sounds and flow related to cardiac contraction and relaxation), audio skills, visual skills, and integration of audio and visual skillsare computed. The same assessment instrument was used for all groups.
Statistical Analysis
Figure 1 lists the tests used to answer the following research questions:
Does the Web‐based curriculum improve CE skills? We compared intervention baseline and posttraining scores using the paired t test for means.
Do interns retain this improvement in skills? We compared intervention baseline and end‐of‐year scores using paired the t test for means.
Does clinical training alone improve CE skills? We compared intervention baseline and control end‐of‐year scores using the t test for means.
Is the Web‐based curriculum better than clinical training alone? We compared intervention and control end‐of‐year scores using the t test for means.
We used the paired t test when baseline and posttraining scores of the same intern could be matched. To test for differences in CE competency between the intervention and control groups, we compared mean scores using the independent Student t test for equal or unequal group variances, as appropriate. Because the interval from posttraining to end‐of‐year testing was variable, it was possible that longer time intervals could allow learning to decay. Therefore, we computed the Spearman correlation coefficient between follow‐up months, and the change in score from post‐Web training to end‐of‐year Pearson correlation coefficients was computed to examine associations of survey variables with test scores. The 2‐sided nominal P < .05 criterion was used to determine statistical significance. Analyses were performed with SPSS statistical software, version 13.0 (SPSS, Inc., Chicago, IL).
Institutional review board approval was granted to this study as exempted research in established educational settings involving normal educational practices.
RESULTS
Research Question 1
Individual baseline and posttraining CE Test scores for the intervention group are plotted in the first 2 columns of Figure 2. The posttraining mean score improved from baseline (66.0 5.2 vs. 54.2 5.4, P = .002). The knowledge, audio, and visual subcategories of CE competence showed similar improvements (P .001; Table 3). The score for the integration of audiovisual skills subcategory was higher at baseline than the other subcategory scores and remained unchanged.
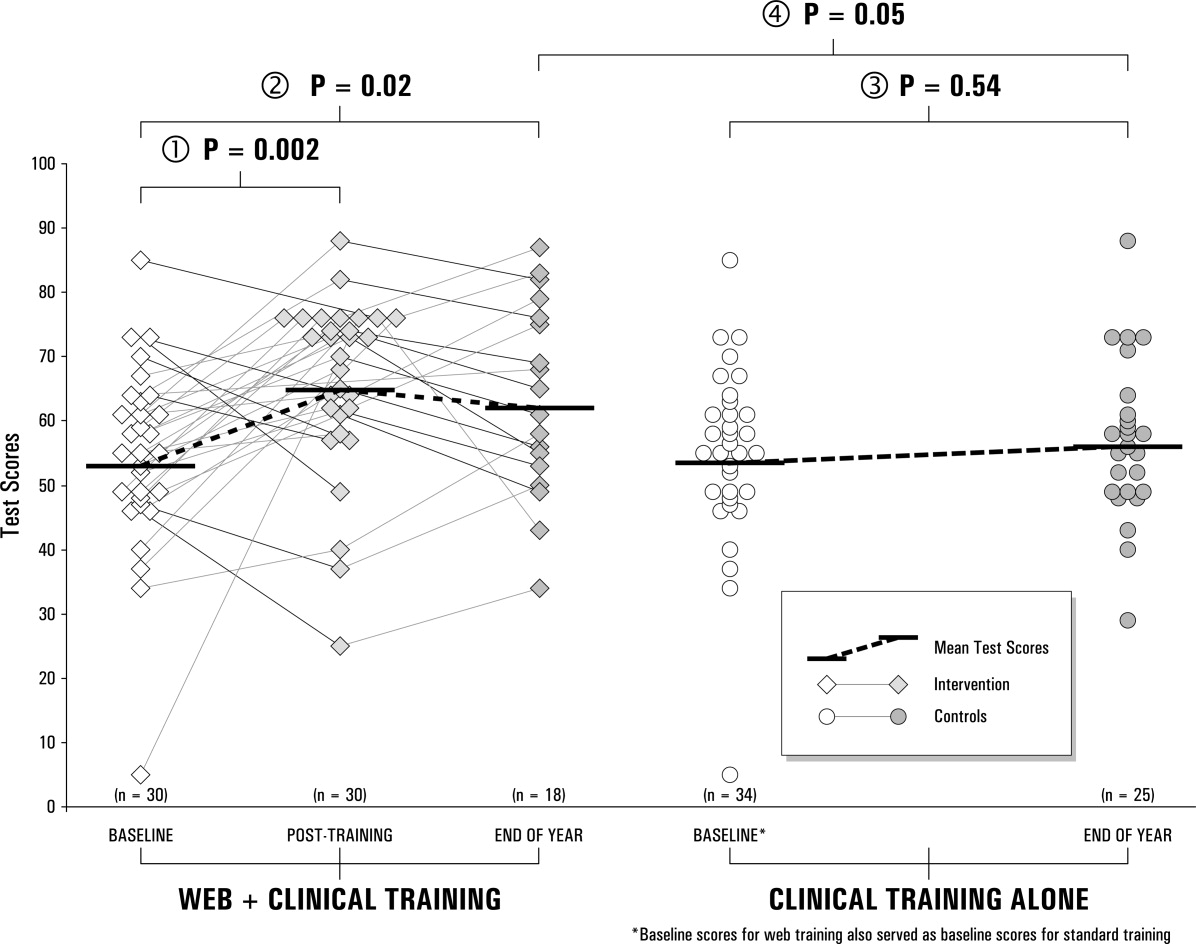
Question 1. Does the Web‐based curriculum improve CE skills? | |||||
---|---|---|---|---|---|
Subcategory | Intervention baseline (n = 30) | Intervention end of year (n = 30) | |||
Mean, % (SD) | 95% CI | Mean, % (SD) | 95% CI | P value (paired t test) | |
Question 2. Do interns retain this improvement? | |||||
Subcategory | Intervention baseline (n = 18) | Intervention end of year (n = 18) | |||
Mean, % (SD) | 95% CI | Mean, % (SD) | 95% CI | P value (paired t test) | |
Question 3. Does clinical training alone improve CE skills? | |||||
Subcategory | Intervention baseline* (n = 34) | Control end of year (n = 25) | |||
Mean, % (SD) | 95% CI | Mean, % (SD) | 95% CI | P value (t test) | |
Question 4. Is the Web‐based curriculum better than clinical training alone? | |||||
Subcategory | Intervention end of year (n = 23) | Control end of year (n = 25) | |||
Mean, % (SD) | 95% CI | Mean, % (SD) | 95% CI | P value (t test) | |
| |||||
Knowledge | 55.9% (14.7%) | 50.4%‐61.4% | 68.9% (15.3%) | 63.2%‐74.6% | <.001 |
Audio | 69.6% (17.4%) | 63.1%‐76.0% | 83.6% (10.3%) | 79.7%‐87.4 % | <.001 |
Visual | 56.7% (21.4%) | 48.7%‐64.7% | 71.9% (16.6%) | 65.7%‐78.1% | <.001 |
Integration | 77.5% (14.5%) | 72.1%‐83.0% | 76.8% (12.8%) | 72.1%‐81.6% | .85 |
Overall score | 54.2% (14.5%) | 48.8%‐59.6% | 66.0% (13.6%) | 60.9%‐71.2% | .002 |
Knowledge | 58.6% (15.2%) | 51.0%‐66.2% | 67.6% (16.7%) | 60.4%‐74.9% | .05 |
Audio | 67.4% (17.1%) | 58.9%‐75.9% | 82.3% (10.4%) | 77.8%‐86.8% | .004 |
Visual | 51.6% (22.0%) | 40.7%‐62.5% | 65.2% (20.6%) | 56.3%‐74.1% | .13 |
Integration | 76.0 % (15.8%) | 68.2%‐83.8% | 77.1% (13.2%) | 71.4%‐82.8% | .95 |
Overall score | 51.8 % (15.0%) | 44.3%‐59.3% | 63.5% (14.9%) | 56.1%‐70.9% | .02 |
Knowledge | 56.5% (14.6%) | 51.4%‐61.6% | 57.8% (15.4%) | 51.4%‐64.1% | .75 |
Audio | 70.0% (16.9%) | 64.1%‐75.9% | 73.3% (13.6%) | 67.7%‐79.0% | .42 |
Visual | 57.6% (20.9%) | 50.3%‐64.9% | 66.9% (15.3%) | 60.6%‐73.2% | .07 |
Integration | 77.8% (13.8%) | 72.9%‐82.5% | 76.0% (12.2%) | 71.0%‐81.0% | .62 |
Overall score | 54.7% (13.7%) | 49.9%‐59.5% | 56.8% (12.4%) | 51.7%‐61.9% | .54 |
Knowledge | 67.6% (16.7%) | 60.4%‐74.8% | 57.8% (15.4%) | 51.4%‐64.1% | 0.04 |
Audio | 82.3% (10.4%) | 77.8%‐86.8% | 73.3% (13.6%) | 67.7%‐79.0% | 0.01 |
Visual | 65.2% (20.6%) | 56.3%‐74.1% | 66.9% (15.3%) | 60.6%‐73.2% | 0.75 |
Integration | 77.1% (13.2%) | 71.4%‐82.8% | 76.0% (12.2%) | 71.0%‐81.0% | 0.76 |
Overall score | 64.7% (14.5%) | 58.4%‐71.0% | 56.8% (12.4%) | 51.7%‐61.9% | 0.05 |
Research Question 2
End‐of‐year scores are plotted in the third column of Figure 2. Overall scores remained higher at the end of the internship year than at baseline (63.5 7.4, P = .02). Improvements in knowledge and audio skills were retained as well (P .05; Table 3). Visual skills (inspection of neck and precordium), however, showed a steep decline, from 71.9 to 65.2, a score indistinguishable from that of the controls (66.9, P = .75). The interval from posttraining to end‐of‐year testing varied; the mean was 21 weeks. The Spearman correlation coefficient between follow‐up months and change in score from post‐Web training to the end of the year was not significant. ( = 0.12, P = .64). To reinforce the results of the correlational analysis, the paired t test was used to compute the t value for 18 interns matched at post‐Web training and retention testing, but the result was not significant (P = .61), indicating that without further training, R1 intervention scores did not change over time.
Research Question 3
Baseline scores for the control group (the prior year's interns) were not available, since the interns had just completed their academic year when the study began. Therefore the baseline scores from the present year's interns were used as a surrogate. Comparing these 2 groups (intervention at baseline, and controls at the end of the year), no difference was observed between mean scores (54.7 4.8 vs. 56.8 5.1, P = .54). Controls scored slightly higher at baseline in visual skills than did interns who received the intervention, but not significantly so (P = .07). There were also no differences in knowledge, audio skills, and integration of audio and visual skills.
Research Question 4
When we compared overall scores at the end of the year, the mean score for the intervention group was higher than that of the controls (64.7 6.3 vs. 56.8 5.1, P = .05). Similarly, knowledge and auditory scores indicated the intervention group had better competency (P .04), but visual and integration skills did not.
Survey
The vast majority of those in both the intervention (88%) and control groups (83%) reported some prior training in CE. In this study the mean total number of hours that those in the intervention group reported they spent preparing was 5.1 2.0 hours (range 2‐25 hours). This number of hours included the three 1‐hour sessions spent with the instructor. The control group reported they spent almost twice as many hours in CE instruction: a mean of 10.1 4.8 hours (range 2‐30 hours, P = .05). On a Likert scale of 1‐5 (from no interest to high interest), both the intervention and control groups expressed high interest in learning CE (4.7 0.2 and 4.4 0.5, respectively; P = .3). Despite significant differences in CE Test scores, the intervention and control groups shared the same confidence in their abilities (2.5 0.3, P = .82). Neither time spent learning nor interest level correlated with overall CE Test score.
DISCUSSION
To improve CE competence of residents, we designed and implemented a Web‐based CE curriculum using virtual patients (VPs), which standardized the interns' exposure to a spectrum of medical conditions and allowed them flexibility in choosing when and where the training occurred.30 In this controlled educational intervention, we found significant improvements in interns' mean CE competency scores immediately following the Web‐based training; these improvements were retained at the end of the academic year. The Web‐based curriculum also improved scores in all 4 subcategories except integration of audio and visual skills. Although cardiac physiology knowledge and audio skills were retained, visual skills showed a steep decline. This decline suggests that visual skills may be more labile and could benefit from more regular reinforcement. It may also be that old habits die hard: in an earlier study of baseline CE competency, we observed that most participants listened with their eyes closed or averted, actively tuning out the visual timing reference that would help them answer the question.7
Comparing control and intervention retention scores suggests that the Web‐based training is more effective than traditional training in CE. The interns spent a mean of 5 hours learning, including 3 hours with the hospitalist‐instructor. Of those 3 hours, 30 minutes was spent taking the test. Earlier studies32, 33 showed improved CE skills with 12‐20 hours of instructor‐led tutorials. The shorter instruction time for interns in this study translated into about half the magnitude of improvement that was seen in third‐year medical students, whose mean score improved from 58.7 6.7 to 73.4 3.8. Moreover, the students in the earlier study continued to show improvement in their CE competency without further intervention, whereas the test scores of interns in this study appeared to plateau. It is therefore likely that 5 hours per year is a lower bound for successful Web‐based CE training. For this reason, we do not recommend fewer than 5 hours per year of CE training using a Web‐based curriculum (including self‐study). Ideally, sessions should be scheduled each year in residency in order to form a critical mass of learning that has the potential to continue improvements beyond residency training.
When surveyed, interns in both the intervention and control groups were very interested in improving their skills but were not confident in their abilities. In fact, confidence did not correlate with ability, which suggests interns (and possibly others)34 do not have a reliable, intrinsic sense of their CE skills. Curiously, the control group reported spending almost twice the number of hours learning CE that the intervention group did. It is unlikely that the intervention group received less traditional instruction than the control group. Therefore, it is more likely that in estimating the hours spent learning CE, the intervention group reported only the formal instruction plus self‐study hours spent on cardiac examination. One way to interpret the greater number of hours of instruction reported by the controls is to look at their end‐of‐the‐year estimate of how much instruction they had received in the previous 12 months. The controls did not benefit from formal instruction in cardiac examination, and so their estimate included the hours spent with physician attendings receiving traditional bedside instruction. The number of hours reported by the controls could be accurate or could be an overestimate. If accurate, the greater number of hours for the controls did not translate into superior performance on the CE Test. If an overestimate, hindsight bias may have inflated the actual hours spent.
Some may argue that VPs are a poor substitute for actual patients. With few reservations, we are inclined to agree. The best training for CE is at the bedside with a clinical master and the luxury of time. Today, however, teaching has shifted from the bedside to conference rooms and even corridors.17, 18 Consequently, opportunities to practice hands‐on CE have become rare. Even when physical examination is performed at the bedside, the quality of the instruction on it depends on the teacher's interest, abilities, and available patients. Our intent was to implement a standardized curriculum that ensures that CE is practiced and tested regularly in order to prevent the creation of a generation of practicing physicians who never develop these skills.
A hospitalist, not a cardiologist, led the intervention. Because cardiac conditions are involved in more than 40% of admissions to the medical wards, a hospitalist should be able to evaluate a patient through physical examination in order to determine whether further tests are necessary or whether a cardiologist should be consulted. In academic medicine, most teaching of cardiac examination is no longer done by cardiologists, but rather by internists.31 Hospital medicine attendings often play a central role as attending physicians for medical students and house officers, and the hospitalist can and should play a larger role in bedside teaching. A Web‐based curriculum for residents that can be accessed at all hours is also suited to residents who have inpatient rotations.
Our CE curriculum was designed for the typical conditions of today's residency training programs, in which education must be balanced with the demands of patient care and the constraints of the limits on resident duty hours.16, 24 Studentfaculty contact time was limited to three 1‐hour sessions, reflecting the time constraints of most training programs.13, 16, 19 The program was incorporated into the regularly scheduled cardiology block throughout the academic year, ensuring that residents received broad exposure to cardiac clinical presentations at a time when bedside encounters with important cardiac physical findings were most likely. The goal was not to reduce or eliminate bedside teaching but to make the limited time that remained more productive. The fidelity of the training to actual bedside encounters was high: unlike the lone audio recordings of heart sounds, VPs in this study also simultaneously presented visual pulsation in the neck or precordium, training the resident to use these visual timing cues while auscultating patients. Furthermore, the Web‐based multimedia technology employed allowed direct comparison of a patient's bedside findings with case‐matched laboratory studies, which was enhanced with explanatory animations and tutorials. Working within the constraints of residency training programs, we were able to standardize the cardiac clinical exposure of trainees and to test for improvement in and retention of CE skills.
Several limitations should be considered. Although VPs mimic bedside patient encounters, we do not know if improvements from this Web‐based curriculum translate into improvements in patient care. Ideally, the study would have been conducted at more than 1 site in order to test whether the positive results we found are replicated elsewhere. Mitigating these drawbacks are the real‐world conditions of our study: the teaching hospital that conducted the study was not involved in the Web site development, and an internist‐hospitalist, not a cardiologist, led the instruction. In theory, the baseline testing itself may have boosted the posttraining scores of the intervention group simply by motivating the interns to improve their test scores. However, only an overall score was reported to interns; they were not told which questions they missed or in what subcategory they were most deficient. Although simple exposure to the test may be the reason for the interns' improvement, it has been shown that the test has testretest reliability over a 4‐ to 6‐week period28, 29 and that test scores do not improve with prior exposure to the test. Because those in the intervention group knew that they would be retested, we cannot eliminate the possibility that they were somehow more motivated to do well on the test by the end of the year. Finally, there may have been differences in the ward training received by the 2 groups of interns studied. The test scores of interns in the control group may not have been as high because of differences in patients admitted, topics discussed, or teaching attendings assigned to them during the academic year; however, because there were no radical changes from the previous year in either the patient population or the group of attending physicians, the differences in the quality of patient encounters between the control and intervention groups are thought to be minor.
In conclusion, the Web‐based curriculum met all the requirements for being effective education: it was self‐directed, interactive, relevant, and cost effective. This novel curriculum standardized learning experience during the cardiology block and saved time for both teacher and trainees. Baseline assessment helped to focus learning. Involvement of an instructor‐hospitalist added accountability, a resource for answering questions, and encouragement for building self‐study skills. More importantly, a realistic and reproducible test of CE skills documented whether the learning objectives were meta feature that is becoming increasingly desirable to residency program directors.35 Our study showed that training with virtual patients yields superior mean CE competency scores over those with standard cardiology ward rotations. Further studies may confirm whether this improvement in CE translates to improvement in making appropriate observations and diagnoses of actual patients.
Acknowledgements
The authors first thank their interns and residents for their willingness to participate in the training and to take the test. They also thank Dr. Lloyd Rucker, program director, University of California Irvine Internal Medicine Residency Program and Dr. John Michael Criley for his guidance in how to use the test and in designing the intervention. Finally, they thank David Criley for his contribution in developing the Web site.
- Cross‐sectional study of contribution of clinical assessment and simple cardiac investigations to diagnosis of left ventricular systolic dysfunction in patients admitted with acute dyspnea.BMJ.1997;314:936–940. , , , , , .
- Agency for Health Care Policy and Research.Heart failure: evaluation and care of patients with left‐ventricular systolic dysfunction.Rockville, MD:U.S. Department of Health and Human Services;1994.
- Value of the cardiovascular physical examination for detecting valvular heart disease in asymptomatic subjects.Am J Cardiol.1996;77:1327–1331. , , .
- Bedside diagnosis of systolic murmurs.N Engl J Med.1988;318:1572–1578. , , , et al.
- Gumbiner C. Cost assessment for evaluation of heart murmurs in children.Pediatrics.1993;91:365:368. , .
- Estimation of pressure gradients by auscultation: an innovative and accurate physical examination technique.Am Heart J.2001;141:500–506. .
- Competency in cardiac examination skills in medical students, trainees, physicians and faculty: a multicenter study.Arch Intern Med.2006;166:610–616. , , , et al.
- The teaching and practice of cardiac auscultation during internal medicine and cardiology training.Ann Intern Med.1993;119:47–54. , , , .
- Cardiac auscultatory skills of internal medicine and family practice trainees: a comparison of diagnostic proficiency.JAMA.1997;278:717–722. , .
- Detection and correction of house staff error in physical diagnosis.JAMA.1983;249:1035–1037. , .
- Is listening through a stethoscope a dying art?Boston Globe May 25,2004. .
- Salvaging the history, physical examination and doctor‐patient relationship in a technological cardiology environment.J Am Coll Cardiol.1999;33:892–893. , .
- Wither the Cardiac Physical Examination?J Am Coll Cardiol.2006;48:2156–2157. .
- New techniques should enhance, not replace, bedside diagnostic skills in cardiology, Part 1.Mod Concepts Cardiovasc Dis.1990;59:19–24. , .
- New techniques should enhance, not replace, bedside diagnostic skills in cardiology, Part 2.Mod Concepts Cardiovasc Dis.1990;59:25–30. , .
- Time, now, to recover the fun in the physical examination rather than abandon it.Arch Intern Med.2006;166:603–604. .
- On bedside teaching.Ann Intern Med.1997;126:217–220. .
- Cassie JM, Daggett CJ. The role of the attending physician in clinical training.J Med Educ.1978;53:429–431. .
- Teaching the resident in infernal medicine: present practices and suggestions for the future.JAMA.1986:256:725–729. . .
- Bedside rounds revisited.N Engl J Med.1997;336:1174–1175. .
- Effects of training in direct observation of medical residents' clinical competence a randomized trial.Ann Intern Med.2004;140:874–881. , , .
- American Board of Internal Medicine. Clinical Skills/PESEP. Available at: http://www.abim.org/moc/semmed.shtm. Accessed July 9,2007.
- Accreditation Council for Graduate Medical Education (ACGME). General Competencies and Outcomes Assessment for Designated Institutional Officials. Available at: http://www.acgme.org/outcome/comp/compFull.asp. Accessed July 9,2007.
- Accreditation Council for Graduate Medical Education, Resident Duty Hours. Available at: http://www.acgme.org/acWebsite/dutyHours/dh_dutyHoursCommonPR.pdf. Accessed, July 9,2007.
- Letter to the Editor re: Cardiac auscultatory skills of physicians‐in‐training: comparison of three English‐speaking countries.Am J Med.2001;111:505–506. .
- Assessing housestaff diagnostic skills using a cardiology patient simulator.Ann Intern Med.1992;117:751–756. , , , et al.
- A comparison of computer assisted instruction and small group teaching of cardiac auscultation to medical students.Med Educ.1991;25:389–395. , , , .
- Validation of a multimedia measure of cardiac physical examination proficiency.Boston, MA:Association of American Medical Colleges Group on Educational Affairs, Research in Medical Education Summary Presentations;November,2004. , , , , .
- Validation of a computerized test to assess competence in the cardiac physical examination. Submitted for publication,2007. , , , , .
- Blaufuss Medical Multimedia Laboratory. Heart sounds tutorial. Available at: http://www.blaufuss.org. Accessed July 7,2007.
- 30th Bethesda Conference: The Future of Academic Cardiology. Task force 3: teaching.J Am Coll Cardiol.1999;33:1120–1127. , .
- Beyond Heart Sounds: An interactive teaching and skills testing program for cardiac examination.Comput Cardiol.2000;27:591–594. , , .
- Using virtual patients to improve cardiac examination competency in medical students. In press,Clin Cardiol. , , , , .
- Accuracy of physician self‐assessment compared with observed measures of competence; a systematic review.JAMA.2006;296: 9;1094–1102. , , , , , .
- Assessing the ACGME general competencies: general considerations and assessment methods.Acad Emerg Med.2002;9:1278–1288. .
Despite impressive advances in cardiac diagnostic technology, cardiac examination (CE) remains an essential skill for screening for abnormal sounds, for evaluating cardiovascular system function, and for guiding further diagnostic testing.16
In practice, these benefits may be attenuated if CE skills are inadequate. Numerous studies have documented substantial CE deficiencies among physicians at various points in their careers from medical school to practice.79 In 1 study, residents' CE mistakes accounted for one‐third of all physical diagnostic errors.10 When murmurs are detected, physicians will often reflexively order an echocardiogram and refer to a cardiologist, regardless of the cost or indication. As a consequence, echocardiography use is rising faster than the aging population or the incidence of cardiac pathological conditions would explain.11 Because cost‐effective medicine depends on the appropriate application of clinical skills like CE, the loss of these skills is a major shortcoming.1215
The reasons for the decline in physicians' CE skills are numerous. High reliance on ordering diagnostic tests,16 conducting teaching rounds away from the bedside,17, 18 time constraints during residency,16, 19 and declining CE skills of faculty members themselves7 all may contribute to the diminished CE skills of residents. Residents, who themselves identify abnormal heart sounds at alarmingly low rates, play an ever‐increasing role in medical students' instruction,7, 9 exacerbating the problem.
Responding to growing concerns over patient safety and quality of care,16, 20 public and professional organizations have called for renewed emphasis on teaching and evaluating clinical skills.21 For example, the American Board of Internal Medicine has added a physical diagnosis component to its recertification program.22 The Accreditation Council for Graduate Medical Education (ACGME) describes general competencies for residents, including patient care that should include proper physical examination skills.23 Although mandating uniform standards is a welcome change for improving CE competence, the challenge remains for medical school deans and program directors to fit structured physical examination skills training into an already crowded curriculum.16, 24 Moreover, the impact of these efforts to improve CE is uncertain because programs lack an objective measure of CE competence.
The CE training is itself a challenge: sight, sound, and touch all contribute to the clinical impression. For this reason, it is difficult to teach away from the bedside. Unlike pulmonary examination, for which a diagnosis is best made by listening, cardiac auscultation is only one (frequently overemphasized) aspect of CE.25 Medical knowledge of cardiac anatomy and physiology, visualization of cardiovascular findings, and integration of auditory and visual findings are all components of accurate CE.7 Traditionally, CE was taught through direct experience with patients at the bedside under the supervision of seasoned clinicians. However, exposure and learning from good teaching patients has waned. Audiotapes, heart sound simulators, mannequins, and other computer‐based interventions have been used as surrogates, but none has been widely adopted.26, 27 The best practice for teaching CE is not known.
To help to improve CE education during residency, we implemented and evaluated a novel Web‐based CE curriculum that emphasized 4 aspects of CE: cardiovascular anatomy and physiology, auditory skills, visual skills, and integration of auscultatory and visual findings. Our hypothesis was that this new curriculum would improve learning of CE skills, that residents would retain what they learn, and that this curriculum would be better than conventional education in teaching CE skills.
METHODS
Study Participants, Site, and Design
Internal medicine (IM) and family medicine (FM) interns (R1s, n = 59) from university‐ and community‐based residency programs, respectively, participated in this controlled trial of an educational intervention to teach CE.
The intervention group consisted of 26 IM and 8 FM interns at the beginning of the academic year in June 2003. To establish baseline scores, all interns took a 50‐question multimedia test of CE competency described previously.7, 28, 29 Subsequently, all interns completed a required 4‐week cardiology ward rotation. During this rotation, they were instructed to complete a Web‐based CE tutorial with accompanying worksheet and to attend 3 one‐hour sessions with a hospitalist instructor. Their schedules were arranged to allow for this educational time. During the third meeting with the instructor, interns were tested again to establish posttraining scores. Finally, at the end of the academic year, interns were tested once again to establish retention scores.
The control group consisted of 25 first‐year IM residents who were tested at the end of their academic year in June 2003. These test scores served as historical controls for interns who had just completed their first year of residency and who had received standard ward rotation without incorporated Web‐based training in CE. Interns from both groups had many opportunities for one‐on‐one instruction in CE because each intern was assigned for the cardiology rotation to a private practice cardiology attending. Figure 1 outlines the number of IM and FM interns eligible and the number actually tested at each stage of the study.
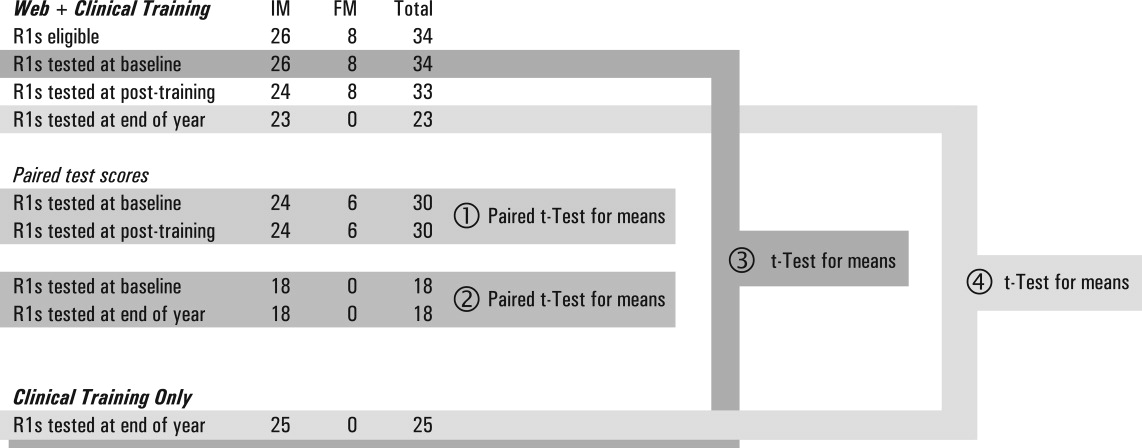
Educational Intervention
The CE curriculum consisted of a Web‐based program and 3 tutored sessions. The program used virtual patientsaudiovisual recordings of actual patientscombined with computer graphic animations and text to teach cardiac anatomy, hemodynamics, pathophysiology, and visual and auditory findings.30 This multimedia program was interactive and allowed comparisons to normal or to similar lesions. The content included cardiac findings identified as important by a survey of IM residency program directors,8 as well as ACGME training requirements for IM residents23 and cardiology fellows.31 Table 1 outlines the content of the Web‐based curriculum.
1. Frontal anatomy of heart, lungs, and vessels with: |
a. Interactive illustrations allowing depiction of individual structures |
b. Separate cartoons of anatomy of the right heart, left heart, and entire heart |
c. Correlation with borders forming regions on chest X‐ray |
2. Interactive phases of the cardiac cycle including: |
a. Phonocardiogram of normal heart sounds (S1, S2) |
b. ECG recording |
c. Left heart (aortic, left atrial, and left ventricular) pressures |
d. Right heart (pulmonary artery, right atrial, and right ventricular) pressures |
e. Animations of the left heart. |
3. Physiological splitting of S2 with: |
a. Phonocardiogram of normal heart sounds |
b. ECG tracing |
c. Left heart (aortic, left atrial, and left ventricular) pressures |
d. Right heart (pulmonary artery, right atrial, and right ventricular) pressures |
e. Interactive animations of the heart and lungs with respiration |
4. Patients with aortic regurgitation (AR) |
a. Integrating pulse with sounds and murmurs |
b. Acute severe AR |
Recognizing Quincke's pulse |
c. Austin Flint murmur |
Differentiating it from the pericardial rub |
d. Hemodynamics of chronic and acute AR and comparisons |
e. Well‐tolerated AR |
5. Patient with aortic stenosis (AS) |
a. Integrating pulse with sounds and murmurs |
Comparison with HCM |
b. Interactive descriptions of hemodynamics and flow |
6. Patients with mitral regurgitation (MR) |
a. Chronic MR |
b. Hemodynamics and comparisons of clinical findings for: |
i. Normal |
ii. Mitral valve prolapse (MVP) |
iii. Acute MR |
iv. Compensated MR |
c. Acute MR |
7. Patients with mitral stenosis (MS) |
a. Introduction: integrating inspection and auscultation |
b. Compare sounds: opening snap, split S2, S3 |
c. Severe MS: interactive comparison of sounds at apex and base |
d. Hemodynamic effects of heart rate |
This training was designed for typical conditions of residency training programs: studentteacher contact time was limited to three 1‐hour sessions; the instructor (J.K.) was an internist hospitalist (trained and facile in the use of the program), not a cardiologist; and self‐paced study was Web‐based to allow access at all hours at the hospital or at home. In their first session, at the beginning of the cardiology block, interns were introduced to the Web site and given a 1‐page homework assignment that corresponded to the Web‐based content (Table 2). During the second session, in the middle of the 4‐week block, a group discussion was held with the Web‐based program, in which the interns asked questions and reviewed their worksheet answers and program as needed with the hospitalist. During the third session, at the end of the block, questions were reviewed, and the interns took the posttraining test.
In preparation for the cardiology heart sounds module during the cardiology block at LBMMC, please answer the following questions and bring the completed questionnaire with you. The correct responses to these questions as well as the underlying mechanisms can be found in the Heart Sounds Tutorial ( |
---|
1. Which cardiac chamber is farthest from the anterior chest wall? __________ |
2. Which cardiac chamber is closest to the left sternal border? ___________ |
3. Are the mitral and aortic valves ever closed at the same time? ____________ |
4. Why does inspiratory lung inflation delay the pulmonic second sound? ____________ |
5. Three or more murmurs of different origin can be heard in aortic regurgitation. What is their timing (within the cardiac cycle) and causation? ____________ |
6. How do you elicit Quincke's pulse? ____________ |
7. Is arterial pulse pressure greater in acute or chronic aortic regurgitation? ____________ |
8. How does the severity of aortic regurgitation correlate with duration of the early diastolic murmur? ____________ |
9. Splitting of the first sound heard with the stethoscope diaphragm in a patient with aortic stenosis is caused by? ____________ |
10. What effect does the severity have on the duration of the murmur of aortic stenosis? ____________ |
11. Is the murmur of aortic stenosis ever holosystolic? ____________ |
12. Is the duration of the murmur of acute mitral regurgitation shorter or longer than that of chronic mitral regurgitation? ____________ |
13. What causes the third heart sound (S3) in mitral regurgitation? ____________ |
14. Why is the jugular venous a‐wave often prominent in mitral stenosis? ____________ |
15. Which heart sound is loudest in mitral stenosis?Why? ____________ |
16. What causes the split sound heard in mitral stenosis? ____________ |
17. Where (on the precordium) would you hear the murmur of mitral stenosis ____________ |
18. How do postural maneuvers affect the heart sounds and murmur in mitral prolapse? ____________ |
19. A 3‐phase friction rub can be confused with the 3‐murmur auditory complex in which valvar lesion? ____________ |
20. What are some causes of third heart sounds that do not imply poor ventricular function? ____________ |
21. Is a fourth heart sound usually best heard at the base (□Yes □No) or the apex (□Yes □No)? |
Evaluation
To evaluate what the R1 intervention group learned, we tested them at baseline, during internship orientation; in posttraining, at the end of their cardiology rotation; and for retention, at the end of their internship year. To evaluate what the controls learned, we tested them at the end of their internship year. The evaluation included a brief survey and the previously validated CE Test.7, 28, 29 Test scores did not carry academic consequences.
For the survey, we asked participants whether they had some prior training in CE and how many hours they estimated having spent learning CE skills during this study with a teacher or in a course. Using a 5‐point Likert scale, they self‐rated their interest and confidence in their own CE skills.
The CE Test is a 50‐question interactive multimedia program that evaluates CE competency using recordings from actual patients. For the CE Test, an overall score (maximum 100 points) and scores for 4 subcategories (expressed as percentages)knowledge of cardiac physiology (interpretation of pressures, sounds and flow related to cardiac contraction and relaxation), audio skills, visual skills, and integration of audio and visual skillsare computed. The same assessment instrument was used for all groups.
Statistical Analysis
Figure 1 lists the tests used to answer the following research questions:
Does the Web‐based curriculum improve CE skills? We compared intervention baseline and posttraining scores using the paired t test for means.
Do interns retain this improvement in skills? We compared intervention baseline and end‐of‐year scores using paired the t test for means.
Does clinical training alone improve CE skills? We compared intervention baseline and control end‐of‐year scores using the t test for means.
Is the Web‐based curriculum better than clinical training alone? We compared intervention and control end‐of‐year scores using the t test for means.
We used the paired t test when baseline and posttraining scores of the same intern could be matched. To test for differences in CE competency between the intervention and control groups, we compared mean scores using the independent Student t test for equal or unequal group variances, as appropriate. Because the interval from posttraining to end‐of‐year testing was variable, it was possible that longer time intervals could allow learning to decay. Therefore, we computed the Spearman correlation coefficient between follow‐up months, and the change in score from post‐Web training to end‐of‐year Pearson correlation coefficients was computed to examine associations of survey variables with test scores. The 2‐sided nominal P < .05 criterion was used to determine statistical significance. Analyses were performed with SPSS statistical software, version 13.0 (SPSS, Inc., Chicago, IL).
Institutional review board approval was granted to this study as exempted research in established educational settings involving normal educational practices.
RESULTS
Research Question 1
Individual baseline and posttraining CE Test scores for the intervention group are plotted in the first 2 columns of Figure 2. The posttraining mean score improved from baseline (66.0 5.2 vs. 54.2 5.4, P = .002). The knowledge, audio, and visual subcategories of CE competence showed similar improvements (P .001; Table 3). The score for the integration of audiovisual skills subcategory was higher at baseline than the other subcategory scores and remained unchanged.
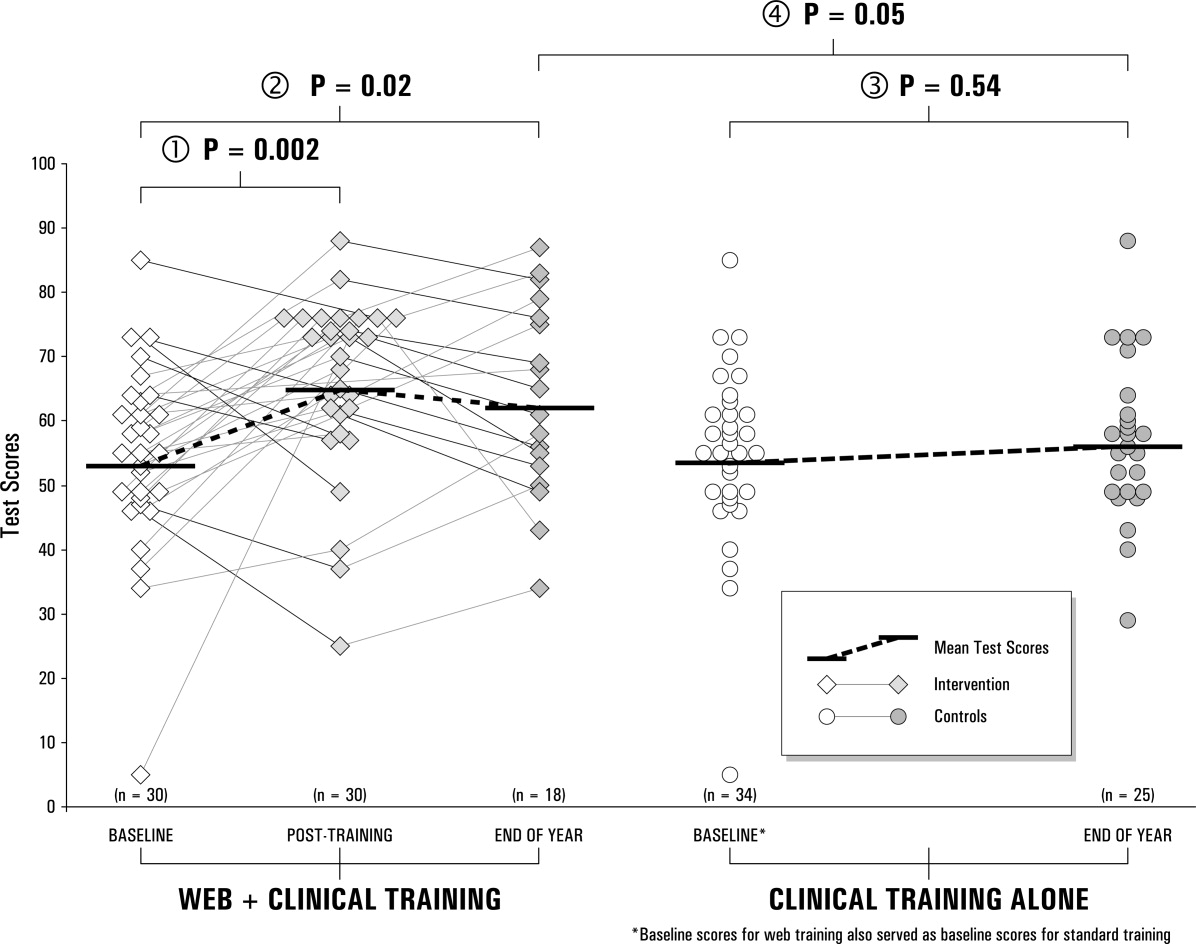
Question 1. Does the Web‐based curriculum improve CE skills? | |||||
---|---|---|---|---|---|
Subcategory | Intervention baseline (n = 30) | Intervention end of year (n = 30) | |||
Mean, % (SD) | 95% CI | Mean, % (SD) | 95% CI | P value (paired t test) | |
Question 2. Do interns retain this improvement? | |||||
Subcategory | Intervention baseline (n = 18) | Intervention end of year (n = 18) | |||
Mean, % (SD) | 95% CI | Mean, % (SD) | 95% CI | P value (paired t test) | |
Question 3. Does clinical training alone improve CE skills? | |||||
Subcategory | Intervention baseline* (n = 34) | Control end of year (n = 25) | |||
Mean, % (SD) | 95% CI | Mean, % (SD) | 95% CI | P value (t test) | |
Question 4. Is the Web‐based curriculum better than clinical training alone? | |||||
Subcategory | Intervention end of year (n = 23) | Control end of year (n = 25) | |||
Mean, % (SD) | 95% CI | Mean, % (SD) | 95% CI | P value (t test) | |
| |||||
Knowledge | 55.9% (14.7%) | 50.4%‐61.4% | 68.9% (15.3%) | 63.2%‐74.6% | <.001 |
Audio | 69.6% (17.4%) | 63.1%‐76.0% | 83.6% (10.3%) | 79.7%‐87.4 % | <.001 |
Visual | 56.7% (21.4%) | 48.7%‐64.7% | 71.9% (16.6%) | 65.7%‐78.1% | <.001 |
Integration | 77.5% (14.5%) | 72.1%‐83.0% | 76.8% (12.8%) | 72.1%‐81.6% | .85 |
Overall score | 54.2% (14.5%) | 48.8%‐59.6% | 66.0% (13.6%) | 60.9%‐71.2% | .002 |
Knowledge | 58.6% (15.2%) | 51.0%‐66.2% | 67.6% (16.7%) | 60.4%‐74.9% | .05 |
Audio | 67.4% (17.1%) | 58.9%‐75.9% | 82.3% (10.4%) | 77.8%‐86.8% | .004 |
Visual | 51.6% (22.0%) | 40.7%‐62.5% | 65.2% (20.6%) | 56.3%‐74.1% | .13 |
Integration | 76.0 % (15.8%) | 68.2%‐83.8% | 77.1% (13.2%) | 71.4%‐82.8% | .95 |
Overall score | 51.8 % (15.0%) | 44.3%‐59.3% | 63.5% (14.9%) | 56.1%‐70.9% | .02 |
Knowledge | 56.5% (14.6%) | 51.4%‐61.6% | 57.8% (15.4%) | 51.4%‐64.1% | .75 |
Audio | 70.0% (16.9%) | 64.1%‐75.9% | 73.3% (13.6%) | 67.7%‐79.0% | .42 |
Visual | 57.6% (20.9%) | 50.3%‐64.9% | 66.9% (15.3%) | 60.6%‐73.2% | .07 |
Integration | 77.8% (13.8%) | 72.9%‐82.5% | 76.0% (12.2%) | 71.0%‐81.0% | .62 |
Overall score | 54.7% (13.7%) | 49.9%‐59.5% | 56.8% (12.4%) | 51.7%‐61.9% | .54 |
Knowledge | 67.6% (16.7%) | 60.4%‐74.8% | 57.8% (15.4%) | 51.4%‐64.1% | 0.04 |
Audio | 82.3% (10.4%) | 77.8%‐86.8% | 73.3% (13.6%) | 67.7%‐79.0% | 0.01 |
Visual | 65.2% (20.6%) | 56.3%‐74.1% | 66.9% (15.3%) | 60.6%‐73.2% | 0.75 |
Integration | 77.1% (13.2%) | 71.4%‐82.8% | 76.0% (12.2%) | 71.0%‐81.0% | 0.76 |
Overall score | 64.7% (14.5%) | 58.4%‐71.0% | 56.8% (12.4%) | 51.7%‐61.9% | 0.05 |
Research Question 2
End‐of‐year scores are plotted in the third column of Figure 2. Overall scores remained higher at the end of the internship year than at baseline (63.5 7.4, P = .02). Improvements in knowledge and audio skills were retained as well (P .05; Table 3). Visual skills (inspection of neck and precordium), however, showed a steep decline, from 71.9 to 65.2, a score indistinguishable from that of the controls (66.9, P = .75). The interval from posttraining to end‐of‐year testing varied; the mean was 21 weeks. The Spearman correlation coefficient between follow‐up months and change in score from post‐Web training to the end of the year was not significant. ( = 0.12, P = .64). To reinforce the results of the correlational analysis, the paired t test was used to compute the t value for 18 interns matched at post‐Web training and retention testing, but the result was not significant (P = .61), indicating that without further training, R1 intervention scores did not change over time.
Research Question 3
Baseline scores for the control group (the prior year's interns) were not available, since the interns had just completed their academic year when the study began. Therefore the baseline scores from the present year's interns were used as a surrogate. Comparing these 2 groups (intervention at baseline, and controls at the end of the year), no difference was observed between mean scores (54.7 4.8 vs. 56.8 5.1, P = .54). Controls scored slightly higher at baseline in visual skills than did interns who received the intervention, but not significantly so (P = .07). There were also no differences in knowledge, audio skills, and integration of audio and visual skills.
Research Question 4
When we compared overall scores at the end of the year, the mean score for the intervention group was higher than that of the controls (64.7 6.3 vs. 56.8 5.1, P = .05). Similarly, knowledge and auditory scores indicated the intervention group had better competency (P .04), but visual and integration skills did not.
Survey
The vast majority of those in both the intervention (88%) and control groups (83%) reported some prior training in CE. In this study the mean total number of hours that those in the intervention group reported they spent preparing was 5.1 2.0 hours (range 2‐25 hours). This number of hours included the three 1‐hour sessions spent with the instructor. The control group reported they spent almost twice as many hours in CE instruction: a mean of 10.1 4.8 hours (range 2‐30 hours, P = .05). On a Likert scale of 1‐5 (from no interest to high interest), both the intervention and control groups expressed high interest in learning CE (4.7 0.2 and 4.4 0.5, respectively; P = .3). Despite significant differences in CE Test scores, the intervention and control groups shared the same confidence in their abilities (2.5 0.3, P = .82). Neither time spent learning nor interest level correlated with overall CE Test score.
DISCUSSION
To improve CE competence of residents, we designed and implemented a Web‐based CE curriculum using virtual patients (VPs), which standardized the interns' exposure to a spectrum of medical conditions and allowed them flexibility in choosing when and where the training occurred.30 In this controlled educational intervention, we found significant improvements in interns' mean CE competency scores immediately following the Web‐based training; these improvements were retained at the end of the academic year. The Web‐based curriculum also improved scores in all 4 subcategories except integration of audio and visual skills. Although cardiac physiology knowledge and audio skills were retained, visual skills showed a steep decline. This decline suggests that visual skills may be more labile and could benefit from more regular reinforcement. It may also be that old habits die hard: in an earlier study of baseline CE competency, we observed that most participants listened with their eyes closed or averted, actively tuning out the visual timing reference that would help them answer the question.7
Comparing control and intervention retention scores suggests that the Web‐based training is more effective than traditional training in CE. The interns spent a mean of 5 hours learning, including 3 hours with the hospitalist‐instructor. Of those 3 hours, 30 minutes was spent taking the test. Earlier studies32, 33 showed improved CE skills with 12‐20 hours of instructor‐led tutorials. The shorter instruction time for interns in this study translated into about half the magnitude of improvement that was seen in third‐year medical students, whose mean score improved from 58.7 6.7 to 73.4 3.8. Moreover, the students in the earlier study continued to show improvement in their CE competency without further intervention, whereas the test scores of interns in this study appeared to plateau. It is therefore likely that 5 hours per year is a lower bound for successful Web‐based CE training. For this reason, we do not recommend fewer than 5 hours per year of CE training using a Web‐based curriculum (including self‐study). Ideally, sessions should be scheduled each year in residency in order to form a critical mass of learning that has the potential to continue improvements beyond residency training.
When surveyed, interns in both the intervention and control groups were very interested in improving their skills but were not confident in their abilities. In fact, confidence did not correlate with ability, which suggests interns (and possibly others)34 do not have a reliable, intrinsic sense of their CE skills. Curiously, the control group reported spending almost twice the number of hours learning CE that the intervention group did. It is unlikely that the intervention group received less traditional instruction than the control group. Therefore, it is more likely that in estimating the hours spent learning CE, the intervention group reported only the formal instruction plus self‐study hours spent on cardiac examination. One way to interpret the greater number of hours of instruction reported by the controls is to look at their end‐of‐the‐year estimate of how much instruction they had received in the previous 12 months. The controls did not benefit from formal instruction in cardiac examination, and so their estimate included the hours spent with physician attendings receiving traditional bedside instruction. The number of hours reported by the controls could be accurate or could be an overestimate. If accurate, the greater number of hours for the controls did not translate into superior performance on the CE Test. If an overestimate, hindsight bias may have inflated the actual hours spent.
Some may argue that VPs are a poor substitute for actual patients. With few reservations, we are inclined to agree. The best training for CE is at the bedside with a clinical master and the luxury of time. Today, however, teaching has shifted from the bedside to conference rooms and even corridors.17, 18 Consequently, opportunities to practice hands‐on CE have become rare. Even when physical examination is performed at the bedside, the quality of the instruction on it depends on the teacher's interest, abilities, and available patients. Our intent was to implement a standardized curriculum that ensures that CE is practiced and tested regularly in order to prevent the creation of a generation of practicing physicians who never develop these skills.
A hospitalist, not a cardiologist, led the intervention. Because cardiac conditions are involved in more than 40% of admissions to the medical wards, a hospitalist should be able to evaluate a patient through physical examination in order to determine whether further tests are necessary or whether a cardiologist should be consulted. In academic medicine, most teaching of cardiac examination is no longer done by cardiologists, but rather by internists.31 Hospital medicine attendings often play a central role as attending physicians for medical students and house officers, and the hospitalist can and should play a larger role in bedside teaching. A Web‐based curriculum for residents that can be accessed at all hours is also suited to residents who have inpatient rotations.
Our CE curriculum was designed for the typical conditions of today's residency training programs, in which education must be balanced with the demands of patient care and the constraints of the limits on resident duty hours.16, 24 Studentfaculty contact time was limited to three 1‐hour sessions, reflecting the time constraints of most training programs.13, 16, 19 The program was incorporated into the regularly scheduled cardiology block throughout the academic year, ensuring that residents received broad exposure to cardiac clinical presentations at a time when bedside encounters with important cardiac physical findings were most likely. The goal was not to reduce or eliminate bedside teaching but to make the limited time that remained more productive. The fidelity of the training to actual bedside encounters was high: unlike the lone audio recordings of heart sounds, VPs in this study also simultaneously presented visual pulsation in the neck or precordium, training the resident to use these visual timing cues while auscultating patients. Furthermore, the Web‐based multimedia technology employed allowed direct comparison of a patient's bedside findings with case‐matched laboratory studies, which was enhanced with explanatory animations and tutorials. Working within the constraints of residency training programs, we were able to standardize the cardiac clinical exposure of trainees and to test for improvement in and retention of CE skills.
Several limitations should be considered. Although VPs mimic bedside patient encounters, we do not know if improvements from this Web‐based curriculum translate into improvements in patient care. Ideally, the study would have been conducted at more than 1 site in order to test whether the positive results we found are replicated elsewhere. Mitigating these drawbacks are the real‐world conditions of our study: the teaching hospital that conducted the study was not involved in the Web site development, and an internist‐hospitalist, not a cardiologist, led the instruction. In theory, the baseline testing itself may have boosted the posttraining scores of the intervention group simply by motivating the interns to improve their test scores. However, only an overall score was reported to interns; they were not told which questions they missed or in what subcategory they were most deficient. Although simple exposure to the test may be the reason for the interns' improvement, it has been shown that the test has testretest reliability over a 4‐ to 6‐week period28, 29 and that test scores do not improve with prior exposure to the test. Because those in the intervention group knew that they would be retested, we cannot eliminate the possibility that they were somehow more motivated to do well on the test by the end of the year. Finally, there may have been differences in the ward training received by the 2 groups of interns studied. The test scores of interns in the control group may not have been as high because of differences in patients admitted, topics discussed, or teaching attendings assigned to them during the academic year; however, because there were no radical changes from the previous year in either the patient population or the group of attending physicians, the differences in the quality of patient encounters between the control and intervention groups are thought to be minor.
In conclusion, the Web‐based curriculum met all the requirements for being effective education: it was self‐directed, interactive, relevant, and cost effective. This novel curriculum standardized learning experience during the cardiology block and saved time for both teacher and trainees. Baseline assessment helped to focus learning. Involvement of an instructor‐hospitalist added accountability, a resource for answering questions, and encouragement for building self‐study skills. More importantly, a realistic and reproducible test of CE skills documented whether the learning objectives were meta feature that is becoming increasingly desirable to residency program directors.35 Our study showed that training with virtual patients yields superior mean CE competency scores over those with standard cardiology ward rotations. Further studies may confirm whether this improvement in CE translates to improvement in making appropriate observations and diagnoses of actual patients.
Acknowledgements
The authors first thank their interns and residents for their willingness to participate in the training and to take the test. They also thank Dr. Lloyd Rucker, program director, University of California Irvine Internal Medicine Residency Program and Dr. John Michael Criley for his guidance in how to use the test and in designing the intervention. Finally, they thank David Criley for his contribution in developing the Web site.
Despite impressive advances in cardiac diagnostic technology, cardiac examination (CE) remains an essential skill for screening for abnormal sounds, for evaluating cardiovascular system function, and for guiding further diagnostic testing.16
In practice, these benefits may be attenuated if CE skills are inadequate. Numerous studies have documented substantial CE deficiencies among physicians at various points in their careers from medical school to practice.79 In 1 study, residents' CE mistakes accounted for one‐third of all physical diagnostic errors.10 When murmurs are detected, physicians will often reflexively order an echocardiogram and refer to a cardiologist, regardless of the cost or indication. As a consequence, echocardiography use is rising faster than the aging population or the incidence of cardiac pathological conditions would explain.11 Because cost‐effective medicine depends on the appropriate application of clinical skills like CE, the loss of these skills is a major shortcoming.1215
The reasons for the decline in physicians' CE skills are numerous. High reliance on ordering diagnostic tests,16 conducting teaching rounds away from the bedside,17, 18 time constraints during residency,16, 19 and declining CE skills of faculty members themselves7 all may contribute to the diminished CE skills of residents. Residents, who themselves identify abnormal heart sounds at alarmingly low rates, play an ever‐increasing role in medical students' instruction,7, 9 exacerbating the problem.
Responding to growing concerns over patient safety and quality of care,16, 20 public and professional organizations have called for renewed emphasis on teaching and evaluating clinical skills.21 For example, the American Board of Internal Medicine has added a physical diagnosis component to its recertification program.22 The Accreditation Council for Graduate Medical Education (ACGME) describes general competencies for residents, including patient care that should include proper physical examination skills.23 Although mandating uniform standards is a welcome change for improving CE competence, the challenge remains for medical school deans and program directors to fit structured physical examination skills training into an already crowded curriculum.16, 24 Moreover, the impact of these efforts to improve CE is uncertain because programs lack an objective measure of CE competence.
The CE training is itself a challenge: sight, sound, and touch all contribute to the clinical impression. For this reason, it is difficult to teach away from the bedside. Unlike pulmonary examination, for which a diagnosis is best made by listening, cardiac auscultation is only one (frequently overemphasized) aspect of CE.25 Medical knowledge of cardiac anatomy and physiology, visualization of cardiovascular findings, and integration of auditory and visual findings are all components of accurate CE.7 Traditionally, CE was taught through direct experience with patients at the bedside under the supervision of seasoned clinicians. However, exposure and learning from good teaching patients has waned. Audiotapes, heart sound simulators, mannequins, and other computer‐based interventions have been used as surrogates, but none has been widely adopted.26, 27 The best practice for teaching CE is not known.
To help to improve CE education during residency, we implemented and evaluated a novel Web‐based CE curriculum that emphasized 4 aspects of CE: cardiovascular anatomy and physiology, auditory skills, visual skills, and integration of auscultatory and visual findings. Our hypothesis was that this new curriculum would improve learning of CE skills, that residents would retain what they learn, and that this curriculum would be better than conventional education in teaching CE skills.
METHODS
Study Participants, Site, and Design
Internal medicine (IM) and family medicine (FM) interns (R1s, n = 59) from university‐ and community‐based residency programs, respectively, participated in this controlled trial of an educational intervention to teach CE.
The intervention group consisted of 26 IM and 8 FM interns at the beginning of the academic year in June 2003. To establish baseline scores, all interns took a 50‐question multimedia test of CE competency described previously.7, 28, 29 Subsequently, all interns completed a required 4‐week cardiology ward rotation. During this rotation, they were instructed to complete a Web‐based CE tutorial with accompanying worksheet and to attend 3 one‐hour sessions with a hospitalist instructor. Their schedules were arranged to allow for this educational time. During the third meeting with the instructor, interns were tested again to establish posttraining scores. Finally, at the end of the academic year, interns were tested once again to establish retention scores.
The control group consisted of 25 first‐year IM residents who were tested at the end of their academic year in June 2003. These test scores served as historical controls for interns who had just completed their first year of residency and who had received standard ward rotation without incorporated Web‐based training in CE. Interns from both groups had many opportunities for one‐on‐one instruction in CE because each intern was assigned for the cardiology rotation to a private practice cardiology attending. Figure 1 outlines the number of IM and FM interns eligible and the number actually tested at each stage of the study.
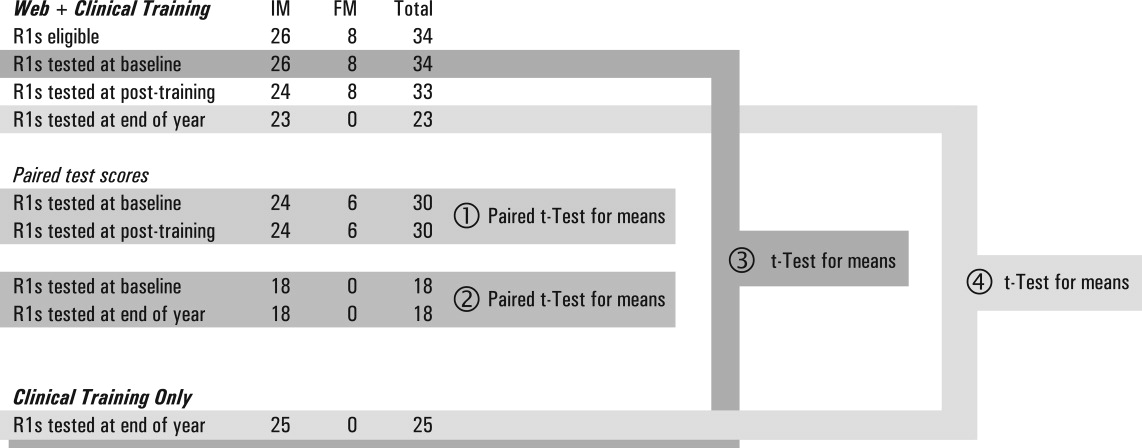
Educational Intervention
The CE curriculum consisted of a Web‐based program and 3 tutored sessions. The program used virtual patientsaudiovisual recordings of actual patientscombined with computer graphic animations and text to teach cardiac anatomy, hemodynamics, pathophysiology, and visual and auditory findings.30 This multimedia program was interactive and allowed comparisons to normal or to similar lesions. The content included cardiac findings identified as important by a survey of IM residency program directors,8 as well as ACGME training requirements for IM residents23 and cardiology fellows.31 Table 1 outlines the content of the Web‐based curriculum.
1. Frontal anatomy of heart, lungs, and vessels with: |
a. Interactive illustrations allowing depiction of individual structures |
b. Separate cartoons of anatomy of the right heart, left heart, and entire heart |
c. Correlation with borders forming regions on chest X‐ray |
2. Interactive phases of the cardiac cycle including: |
a. Phonocardiogram of normal heart sounds (S1, S2) |
b. ECG recording |
c. Left heart (aortic, left atrial, and left ventricular) pressures |
d. Right heart (pulmonary artery, right atrial, and right ventricular) pressures |
e. Animations of the left heart. |
3. Physiological splitting of S2 with: |
a. Phonocardiogram of normal heart sounds |
b. ECG tracing |
c. Left heart (aortic, left atrial, and left ventricular) pressures |
d. Right heart (pulmonary artery, right atrial, and right ventricular) pressures |
e. Interactive animations of the heart and lungs with respiration |
4. Patients with aortic regurgitation (AR) |
a. Integrating pulse with sounds and murmurs |
b. Acute severe AR |
Recognizing Quincke's pulse |
c. Austin Flint murmur |
Differentiating it from the pericardial rub |
d. Hemodynamics of chronic and acute AR and comparisons |
e. Well‐tolerated AR |
5. Patient with aortic stenosis (AS) |
a. Integrating pulse with sounds and murmurs |
Comparison with HCM |
b. Interactive descriptions of hemodynamics and flow |
6. Patients with mitral regurgitation (MR) |
a. Chronic MR |
b. Hemodynamics and comparisons of clinical findings for: |
i. Normal |
ii. Mitral valve prolapse (MVP) |
iii. Acute MR |
iv. Compensated MR |
c. Acute MR |
7. Patients with mitral stenosis (MS) |
a. Introduction: integrating inspection and auscultation |
b. Compare sounds: opening snap, split S2, S3 |
c. Severe MS: interactive comparison of sounds at apex and base |
d. Hemodynamic effects of heart rate |
This training was designed for typical conditions of residency training programs: studentteacher contact time was limited to three 1‐hour sessions; the instructor (J.K.) was an internist hospitalist (trained and facile in the use of the program), not a cardiologist; and self‐paced study was Web‐based to allow access at all hours at the hospital or at home. In their first session, at the beginning of the cardiology block, interns were introduced to the Web site and given a 1‐page homework assignment that corresponded to the Web‐based content (Table 2). During the second session, in the middle of the 4‐week block, a group discussion was held with the Web‐based program, in which the interns asked questions and reviewed their worksheet answers and program as needed with the hospitalist. During the third session, at the end of the block, questions were reviewed, and the interns took the posttraining test.
In preparation for the cardiology heart sounds module during the cardiology block at LBMMC, please answer the following questions and bring the completed questionnaire with you. The correct responses to these questions as well as the underlying mechanisms can be found in the Heart Sounds Tutorial ( |
---|
1. Which cardiac chamber is farthest from the anterior chest wall? __________ |
2. Which cardiac chamber is closest to the left sternal border? ___________ |
3. Are the mitral and aortic valves ever closed at the same time? ____________ |
4. Why does inspiratory lung inflation delay the pulmonic second sound? ____________ |
5. Three or more murmurs of different origin can be heard in aortic regurgitation. What is their timing (within the cardiac cycle) and causation? ____________ |
6. How do you elicit Quincke's pulse? ____________ |
7. Is arterial pulse pressure greater in acute or chronic aortic regurgitation? ____________ |
8. How does the severity of aortic regurgitation correlate with duration of the early diastolic murmur? ____________ |
9. Splitting of the first sound heard with the stethoscope diaphragm in a patient with aortic stenosis is caused by? ____________ |
10. What effect does the severity have on the duration of the murmur of aortic stenosis? ____________ |
11. Is the murmur of aortic stenosis ever holosystolic? ____________ |
12. Is the duration of the murmur of acute mitral regurgitation shorter or longer than that of chronic mitral regurgitation? ____________ |
13. What causes the third heart sound (S3) in mitral regurgitation? ____________ |
14. Why is the jugular venous a‐wave often prominent in mitral stenosis? ____________ |
15. Which heart sound is loudest in mitral stenosis?Why? ____________ |
16. What causes the split sound heard in mitral stenosis? ____________ |
17. Where (on the precordium) would you hear the murmur of mitral stenosis ____________ |
18. How do postural maneuvers affect the heart sounds and murmur in mitral prolapse? ____________ |
19. A 3‐phase friction rub can be confused with the 3‐murmur auditory complex in which valvar lesion? ____________ |
20. What are some causes of third heart sounds that do not imply poor ventricular function? ____________ |
21. Is a fourth heart sound usually best heard at the base (□Yes □No) or the apex (□Yes □No)? |
Evaluation
To evaluate what the R1 intervention group learned, we tested them at baseline, during internship orientation; in posttraining, at the end of their cardiology rotation; and for retention, at the end of their internship year. To evaluate what the controls learned, we tested them at the end of their internship year. The evaluation included a brief survey and the previously validated CE Test.7, 28, 29 Test scores did not carry academic consequences.
For the survey, we asked participants whether they had some prior training in CE and how many hours they estimated having spent learning CE skills during this study with a teacher or in a course. Using a 5‐point Likert scale, they self‐rated their interest and confidence in their own CE skills.
The CE Test is a 50‐question interactive multimedia program that evaluates CE competency using recordings from actual patients. For the CE Test, an overall score (maximum 100 points) and scores for 4 subcategories (expressed as percentages)knowledge of cardiac physiology (interpretation of pressures, sounds and flow related to cardiac contraction and relaxation), audio skills, visual skills, and integration of audio and visual skillsare computed. The same assessment instrument was used for all groups.
Statistical Analysis
Figure 1 lists the tests used to answer the following research questions:
Does the Web‐based curriculum improve CE skills? We compared intervention baseline and posttraining scores using the paired t test for means.
Do interns retain this improvement in skills? We compared intervention baseline and end‐of‐year scores using paired the t test for means.
Does clinical training alone improve CE skills? We compared intervention baseline and control end‐of‐year scores using the t test for means.
Is the Web‐based curriculum better than clinical training alone? We compared intervention and control end‐of‐year scores using the t test for means.
We used the paired t test when baseline and posttraining scores of the same intern could be matched. To test for differences in CE competency between the intervention and control groups, we compared mean scores using the independent Student t test for equal or unequal group variances, as appropriate. Because the interval from posttraining to end‐of‐year testing was variable, it was possible that longer time intervals could allow learning to decay. Therefore, we computed the Spearman correlation coefficient between follow‐up months, and the change in score from post‐Web training to end‐of‐year Pearson correlation coefficients was computed to examine associations of survey variables with test scores. The 2‐sided nominal P < .05 criterion was used to determine statistical significance. Analyses were performed with SPSS statistical software, version 13.0 (SPSS, Inc., Chicago, IL).
Institutional review board approval was granted to this study as exempted research in established educational settings involving normal educational practices.
RESULTS
Research Question 1
Individual baseline and posttraining CE Test scores for the intervention group are plotted in the first 2 columns of Figure 2. The posttraining mean score improved from baseline (66.0 5.2 vs. 54.2 5.4, P = .002). The knowledge, audio, and visual subcategories of CE competence showed similar improvements (P .001; Table 3). The score for the integration of audiovisual skills subcategory was higher at baseline than the other subcategory scores and remained unchanged.
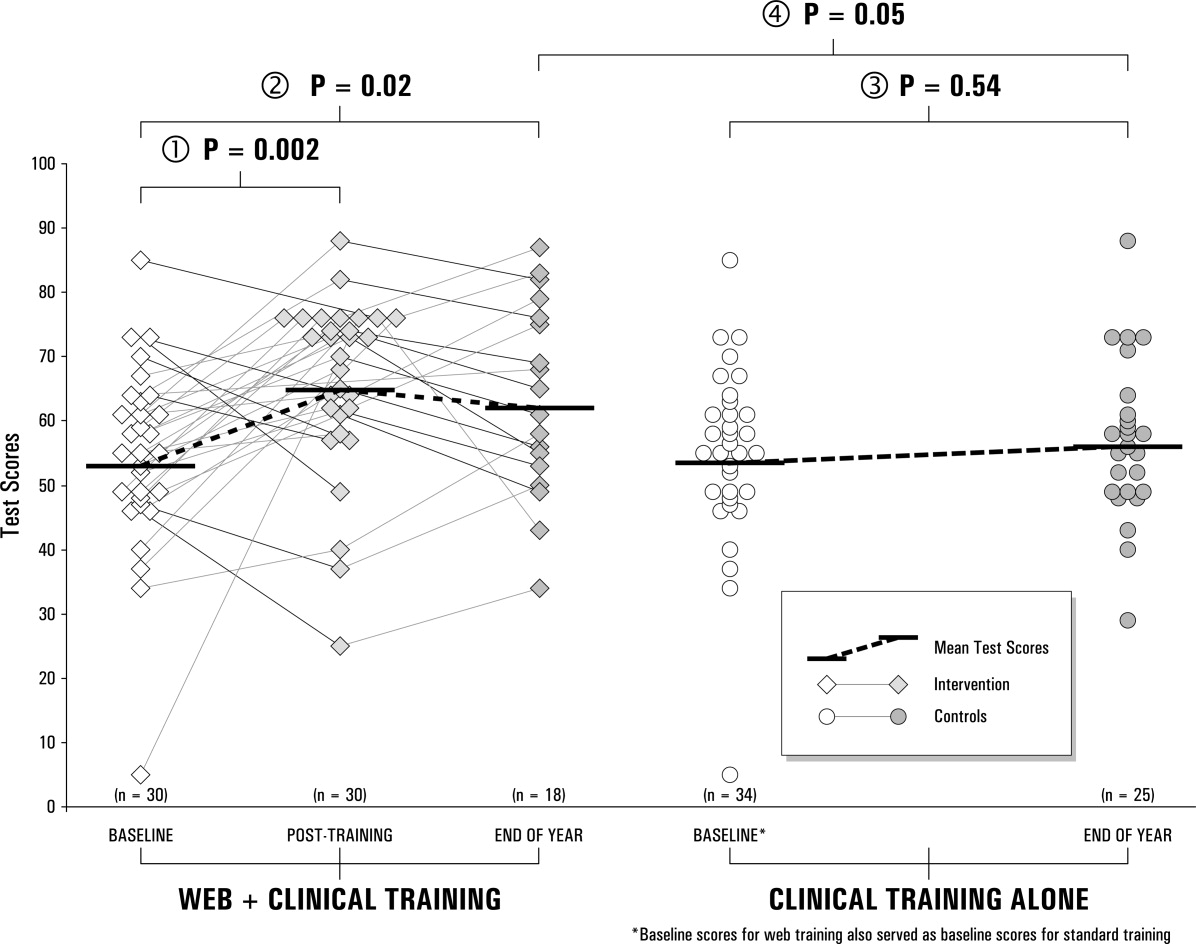
Question 1. Does the Web‐based curriculum improve CE skills? | |||||
---|---|---|---|---|---|
Subcategory | Intervention baseline (n = 30) | Intervention end of year (n = 30) | |||
Mean, % (SD) | 95% CI | Mean, % (SD) | 95% CI | P value (paired t test) | |
Question 2. Do interns retain this improvement? | |||||
Subcategory | Intervention baseline (n = 18) | Intervention end of year (n = 18) | |||
Mean, % (SD) | 95% CI | Mean, % (SD) | 95% CI | P value (paired t test) | |
Question 3. Does clinical training alone improve CE skills? | |||||
Subcategory | Intervention baseline* (n = 34) | Control end of year (n = 25) | |||
Mean, % (SD) | 95% CI | Mean, % (SD) | 95% CI | P value (t test) | |
Question 4. Is the Web‐based curriculum better than clinical training alone? | |||||
Subcategory | Intervention end of year (n = 23) | Control end of year (n = 25) | |||
Mean, % (SD) | 95% CI | Mean, % (SD) | 95% CI | P value (t test) | |
| |||||
Knowledge | 55.9% (14.7%) | 50.4%‐61.4% | 68.9% (15.3%) | 63.2%‐74.6% | <.001 |
Audio | 69.6% (17.4%) | 63.1%‐76.0% | 83.6% (10.3%) | 79.7%‐87.4 % | <.001 |
Visual | 56.7% (21.4%) | 48.7%‐64.7% | 71.9% (16.6%) | 65.7%‐78.1% | <.001 |
Integration | 77.5% (14.5%) | 72.1%‐83.0% | 76.8% (12.8%) | 72.1%‐81.6% | .85 |
Overall score | 54.2% (14.5%) | 48.8%‐59.6% | 66.0% (13.6%) | 60.9%‐71.2% | .002 |
Knowledge | 58.6% (15.2%) | 51.0%‐66.2% | 67.6% (16.7%) | 60.4%‐74.9% | .05 |
Audio | 67.4% (17.1%) | 58.9%‐75.9% | 82.3% (10.4%) | 77.8%‐86.8% | .004 |
Visual | 51.6% (22.0%) | 40.7%‐62.5% | 65.2% (20.6%) | 56.3%‐74.1% | .13 |
Integration | 76.0 % (15.8%) | 68.2%‐83.8% | 77.1% (13.2%) | 71.4%‐82.8% | .95 |
Overall score | 51.8 % (15.0%) | 44.3%‐59.3% | 63.5% (14.9%) | 56.1%‐70.9% | .02 |
Knowledge | 56.5% (14.6%) | 51.4%‐61.6% | 57.8% (15.4%) | 51.4%‐64.1% | .75 |
Audio | 70.0% (16.9%) | 64.1%‐75.9% | 73.3% (13.6%) | 67.7%‐79.0% | .42 |
Visual | 57.6% (20.9%) | 50.3%‐64.9% | 66.9% (15.3%) | 60.6%‐73.2% | .07 |
Integration | 77.8% (13.8%) | 72.9%‐82.5% | 76.0% (12.2%) | 71.0%‐81.0% | .62 |
Overall score | 54.7% (13.7%) | 49.9%‐59.5% | 56.8% (12.4%) | 51.7%‐61.9% | .54 |
Knowledge | 67.6% (16.7%) | 60.4%‐74.8% | 57.8% (15.4%) | 51.4%‐64.1% | 0.04 |
Audio | 82.3% (10.4%) | 77.8%‐86.8% | 73.3% (13.6%) | 67.7%‐79.0% | 0.01 |
Visual | 65.2% (20.6%) | 56.3%‐74.1% | 66.9% (15.3%) | 60.6%‐73.2% | 0.75 |
Integration | 77.1% (13.2%) | 71.4%‐82.8% | 76.0% (12.2%) | 71.0%‐81.0% | 0.76 |
Overall score | 64.7% (14.5%) | 58.4%‐71.0% | 56.8% (12.4%) | 51.7%‐61.9% | 0.05 |
Research Question 2
End‐of‐year scores are plotted in the third column of Figure 2. Overall scores remained higher at the end of the internship year than at baseline (63.5 7.4, P = .02). Improvements in knowledge and audio skills were retained as well (P .05; Table 3). Visual skills (inspection of neck and precordium), however, showed a steep decline, from 71.9 to 65.2, a score indistinguishable from that of the controls (66.9, P = .75). The interval from posttraining to end‐of‐year testing varied; the mean was 21 weeks. The Spearman correlation coefficient between follow‐up months and change in score from post‐Web training to the end of the year was not significant. ( = 0.12, P = .64). To reinforce the results of the correlational analysis, the paired t test was used to compute the t value for 18 interns matched at post‐Web training and retention testing, but the result was not significant (P = .61), indicating that without further training, R1 intervention scores did not change over time.
Research Question 3
Baseline scores for the control group (the prior year's interns) were not available, since the interns had just completed their academic year when the study began. Therefore the baseline scores from the present year's interns were used as a surrogate. Comparing these 2 groups (intervention at baseline, and controls at the end of the year), no difference was observed between mean scores (54.7 4.8 vs. 56.8 5.1, P = .54). Controls scored slightly higher at baseline in visual skills than did interns who received the intervention, but not significantly so (P = .07). There were also no differences in knowledge, audio skills, and integration of audio and visual skills.
Research Question 4
When we compared overall scores at the end of the year, the mean score for the intervention group was higher than that of the controls (64.7 6.3 vs. 56.8 5.1, P = .05). Similarly, knowledge and auditory scores indicated the intervention group had better competency (P .04), but visual and integration skills did not.
Survey
The vast majority of those in both the intervention (88%) and control groups (83%) reported some prior training in CE. In this study the mean total number of hours that those in the intervention group reported they spent preparing was 5.1 2.0 hours (range 2‐25 hours). This number of hours included the three 1‐hour sessions spent with the instructor. The control group reported they spent almost twice as many hours in CE instruction: a mean of 10.1 4.8 hours (range 2‐30 hours, P = .05). On a Likert scale of 1‐5 (from no interest to high interest), both the intervention and control groups expressed high interest in learning CE (4.7 0.2 and 4.4 0.5, respectively; P = .3). Despite significant differences in CE Test scores, the intervention and control groups shared the same confidence in their abilities (2.5 0.3, P = .82). Neither time spent learning nor interest level correlated with overall CE Test score.
DISCUSSION
To improve CE competence of residents, we designed and implemented a Web‐based CE curriculum using virtual patients (VPs), which standardized the interns' exposure to a spectrum of medical conditions and allowed them flexibility in choosing when and where the training occurred.30 In this controlled educational intervention, we found significant improvements in interns' mean CE competency scores immediately following the Web‐based training; these improvements were retained at the end of the academic year. The Web‐based curriculum also improved scores in all 4 subcategories except integration of audio and visual skills. Although cardiac physiology knowledge and audio skills were retained, visual skills showed a steep decline. This decline suggests that visual skills may be more labile and could benefit from more regular reinforcement. It may also be that old habits die hard: in an earlier study of baseline CE competency, we observed that most participants listened with their eyes closed or averted, actively tuning out the visual timing reference that would help them answer the question.7
Comparing control and intervention retention scores suggests that the Web‐based training is more effective than traditional training in CE. The interns spent a mean of 5 hours learning, including 3 hours with the hospitalist‐instructor. Of those 3 hours, 30 minutes was spent taking the test. Earlier studies32, 33 showed improved CE skills with 12‐20 hours of instructor‐led tutorials. The shorter instruction time for interns in this study translated into about half the magnitude of improvement that was seen in third‐year medical students, whose mean score improved from 58.7 6.7 to 73.4 3.8. Moreover, the students in the earlier study continued to show improvement in their CE competency without further intervention, whereas the test scores of interns in this study appeared to plateau. It is therefore likely that 5 hours per year is a lower bound for successful Web‐based CE training. For this reason, we do not recommend fewer than 5 hours per year of CE training using a Web‐based curriculum (including self‐study). Ideally, sessions should be scheduled each year in residency in order to form a critical mass of learning that has the potential to continue improvements beyond residency training.
When surveyed, interns in both the intervention and control groups were very interested in improving their skills but were not confident in their abilities. In fact, confidence did not correlate with ability, which suggests interns (and possibly others)34 do not have a reliable, intrinsic sense of their CE skills. Curiously, the control group reported spending almost twice the number of hours learning CE that the intervention group did. It is unlikely that the intervention group received less traditional instruction than the control group. Therefore, it is more likely that in estimating the hours spent learning CE, the intervention group reported only the formal instruction plus self‐study hours spent on cardiac examination. One way to interpret the greater number of hours of instruction reported by the controls is to look at their end‐of‐the‐year estimate of how much instruction they had received in the previous 12 months. The controls did not benefit from formal instruction in cardiac examination, and so their estimate included the hours spent with physician attendings receiving traditional bedside instruction. The number of hours reported by the controls could be accurate or could be an overestimate. If accurate, the greater number of hours for the controls did not translate into superior performance on the CE Test. If an overestimate, hindsight bias may have inflated the actual hours spent.
Some may argue that VPs are a poor substitute for actual patients. With few reservations, we are inclined to agree. The best training for CE is at the bedside with a clinical master and the luxury of time. Today, however, teaching has shifted from the bedside to conference rooms and even corridors.17, 18 Consequently, opportunities to practice hands‐on CE have become rare. Even when physical examination is performed at the bedside, the quality of the instruction on it depends on the teacher's interest, abilities, and available patients. Our intent was to implement a standardized curriculum that ensures that CE is practiced and tested regularly in order to prevent the creation of a generation of practicing physicians who never develop these skills.
A hospitalist, not a cardiologist, led the intervention. Because cardiac conditions are involved in more than 40% of admissions to the medical wards, a hospitalist should be able to evaluate a patient through physical examination in order to determine whether further tests are necessary or whether a cardiologist should be consulted. In academic medicine, most teaching of cardiac examination is no longer done by cardiologists, but rather by internists.31 Hospital medicine attendings often play a central role as attending physicians for medical students and house officers, and the hospitalist can and should play a larger role in bedside teaching. A Web‐based curriculum for residents that can be accessed at all hours is also suited to residents who have inpatient rotations.
Our CE curriculum was designed for the typical conditions of today's residency training programs, in which education must be balanced with the demands of patient care and the constraints of the limits on resident duty hours.16, 24 Studentfaculty contact time was limited to three 1‐hour sessions, reflecting the time constraints of most training programs.13, 16, 19 The program was incorporated into the regularly scheduled cardiology block throughout the academic year, ensuring that residents received broad exposure to cardiac clinical presentations at a time when bedside encounters with important cardiac physical findings were most likely. The goal was not to reduce or eliminate bedside teaching but to make the limited time that remained more productive. The fidelity of the training to actual bedside encounters was high: unlike the lone audio recordings of heart sounds, VPs in this study also simultaneously presented visual pulsation in the neck or precordium, training the resident to use these visual timing cues while auscultating patients. Furthermore, the Web‐based multimedia technology employed allowed direct comparison of a patient's bedside findings with case‐matched laboratory studies, which was enhanced with explanatory animations and tutorials. Working within the constraints of residency training programs, we were able to standardize the cardiac clinical exposure of trainees and to test for improvement in and retention of CE skills.
Several limitations should be considered. Although VPs mimic bedside patient encounters, we do not know if improvements from this Web‐based curriculum translate into improvements in patient care. Ideally, the study would have been conducted at more than 1 site in order to test whether the positive results we found are replicated elsewhere. Mitigating these drawbacks are the real‐world conditions of our study: the teaching hospital that conducted the study was not involved in the Web site development, and an internist‐hospitalist, not a cardiologist, led the instruction. In theory, the baseline testing itself may have boosted the posttraining scores of the intervention group simply by motivating the interns to improve their test scores. However, only an overall score was reported to interns; they were not told which questions they missed or in what subcategory they were most deficient. Although simple exposure to the test may be the reason for the interns' improvement, it has been shown that the test has testretest reliability over a 4‐ to 6‐week period28, 29 and that test scores do not improve with prior exposure to the test. Because those in the intervention group knew that they would be retested, we cannot eliminate the possibility that they were somehow more motivated to do well on the test by the end of the year. Finally, there may have been differences in the ward training received by the 2 groups of interns studied. The test scores of interns in the control group may not have been as high because of differences in patients admitted, topics discussed, or teaching attendings assigned to them during the academic year; however, because there were no radical changes from the previous year in either the patient population or the group of attending physicians, the differences in the quality of patient encounters between the control and intervention groups are thought to be minor.
In conclusion, the Web‐based curriculum met all the requirements for being effective education: it was self‐directed, interactive, relevant, and cost effective. This novel curriculum standardized learning experience during the cardiology block and saved time for both teacher and trainees. Baseline assessment helped to focus learning. Involvement of an instructor‐hospitalist added accountability, a resource for answering questions, and encouragement for building self‐study skills. More importantly, a realistic and reproducible test of CE skills documented whether the learning objectives were meta feature that is becoming increasingly desirable to residency program directors.35 Our study showed that training with virtual patients yields superior mean CE competency scores over those with standard cardiology ward rotations. Further studies may confirm whether this improvement in CE translates to improvement in making appropriate observations and diagnoses of actual patients.
Acknowledgements
The authors first thank their interns and residents for their willingness to participate in the training and to take the test. They also thank Dr. Lloyd Rucker, program director, University of California Irvine Internal Medicine Residency Program and Dr. John Michael Criley for his guidance in how to use the test and in designing the intervention. Finally, they thank David Criley for his contribution in developing the Web site.
- Cross‐sectional study of contribution of clinical assessment and simple cardiac investigations to diagnosis of left ventricular systolic dysfunction in patients admitted with acute dyspnea.BMJ.1997;314:936–940. , , , , , .
- Agency for Health Care Policy and Research.Heart failure: evaluation and care of patients with left‐ventricular systolic dysfunction.Rockville, MD:U.S. Department of Health and Human Services;1994.
- Value of the cardiovascular physical examination for detecting valvular heart disease in asymptomatic subjects.Am J Cardiol.1996;77:1327–1331. , , .
- Bedside diagnosis of systolic murmurs.N Engl J Med.1988;318:1572–1578. , , , et al.
- Gumbiner C. Cost assessment for evaluation of heart murmurs in children.Pediatrics.1993;91:365:368. , .
- Estimation of pressure gradients by auscultation: an innovative and accurate physical examination technique.Am Heart J.2001;141:500–506. .
- Competency in cardiac examination skills in medical students, trainees, physicians and faculty: a multicenter study.Arch Intern Med.2006;166:610–616. , , , et al.
- The teaching and practice of cardiac auscultation during internal medicine and cardiology training.Ann Intern Med.1993;119:47–54. , , , .
- Cardiac auscultatory skills of internal medicine and family practice trainees: a comparison of diagnostic proficiency.JAMA.1997;278:717–722. , .
- Detection and correction of house staff error in physical diagnosis.JAMA.1983;249:1035–1037. , .
- Is listening through a stethoscope a dying art?Boston Globe May 25,2004. .
- Salvaging the history, physical examination and doctor‐patient relationship in a technological cardiology environment.J Am Coll Cardiol.1999;33:892–893. , .
- Wither the Cardiac Physical Examination?J Am Coll Cardiol.2006;48:2156–2157. .
- New techniques should enhance, not replace, bedside diagnostic skills in cardiology, Part 1.Mod Concepts Cardiovasc Dis.1990;59:19–24. , .
- New techniques should enhance, not replace, bedside diagnostic skills in cardiology, Part 2.Mod Concepts Cardiovasc Dis.1990;59:25–30. , .
- Time, now, to recover the fun in the physical examination rather than abandon it.Arch Intern Med.2006;166:603–604. .
- On bedside teaching.Ann Intern Med.1997;126:217–220. .
- Cassie JM, Daggett CJ. The role of the attending physician in clinical training.J Med Educ.1978;53:429–431. .
- Teaching the resident in infernal medicine: present practices and suggestions for the future.JAMA.1986:256:725–729. . .
- Bedside rounds revisited.N Engl J Med.1997;336:1174–1175. .
- Effects of training in direct observation of medical residents' clinical competence a randomized trial.Ann Intern Med.2004;140:874–881. , , .
- American Board of Internal Medicine. Clinical Skills/PESEP. Available at: http://www.abim.org/moc/semmed.shtm. Accessed July 9,2007.
- Accreditation Council for Graduate Medical Education (ACGME). General Competencies and Outcomes Assessment for Designated Institutional Officials. Available at: http://www.acgme.org/outcome/comp/compFull.asp. Accessed July 9,2007.
- Accreditation Council for Graduate Medical Education, Resident Duty Hours. Available at: http://www.acgme.org/acWebsite/dutyHours/dh_dutyHoursCommonPR.pdf. Accessed, July 9,2007.
- Letter to the Editor re: Cardiac auscultatory skills of physicians‐in‐training: comparison of three English‐speaking countries.Am J Med.2001;111:505–506. .
- Assessing housestaff diagnostic skills using a cardiology patient simulator.Ann Intern Med.1992;117:751–756. , , , et al.
- A comparison of computer assisted instruction and small group teaching of cardiac auscultation to medical students.Med Educ.1991;25:389–395. , , , .
- Validation of a multimedia measure of cardiac physical examination proficiency.Boston, MA:Association of American Medical Colleges Group on Educational Affairs, Research in Medical Education Summary Presentations;November,2004. , , , , .
- Validation of a computerized test to assess competence in the cardiac physical examination. Submitted for publication,2007. , , , , .
- Blaufuss Medical Multimedia Laboratory. Heart sounds tutorial. Available at: http://www.blaufuss.org. Accessed July 7,2007.
- 30th Bethesda Conference: The Future of Academic Cardiology. Task force 3: teaching.J Am Coll Cardiol.1999;33:1120–1127. , .
- Beyond Heart Sounds: An interactive teaching and skills testing program for cardiac examination.Comput Cardiol.2000;27:591–594. , , .
- Using virtual patients to improve cardiac examination competency in medical students. In press,Clin Cardiol. , , , , .
- Accuracy of physician self‐assessment compared with observed measures of competence; a systematic review.JAMA.2006;296: 9;1094–1102. , , , , , .
- Assessing the ACGME general competencies: general considerations and assessment methods.Acad Emerg Med.2002;9:1278–1288. .
- Cross‐sectional study of contribution of clinical assessment and simple cardiac investigations to diagnosis of left ventricular systolic dysfunction in patients admitted with acute dyspnea.BMJ.1997;314:936–940. , , , , , .
- Agency for Health Care Policy and Research.Heart failure: evaluation and care of patients with left‐ventricular systolic dysfunction.Rockville, MD:U.S. Department of Health and Human Services;1994.
- Value of the cardiovascular physical examination for detecting valvular heart disease in asymptomatic subjects.Am J Cardiol.1996;77:1327–1331. , , .
- Bedside diagnosis of systolic murmurs.N Engl J Med.1988;318:1572–1578. , , , et al.
- Gumbiner C. Cost assessment for evaluation of heart murmurs in children.Pediatrics.1993;91:365:368. , .
- Estimation of pressure gradients by auscultation: an innovative and accurate physical examination technique.Am Heart J.2001;141:500–506. .
- Competency in cardiac examination skills in medical students, trainees, physicians and faculty: a multicenter study.Arch Intern Med.2006;166:610–616. , , , et al.
- The teaching and practice of cardiac auscultation during internal medicine and cardiology training.Ann Intern Med.1993;119:47–54. , , , .
- Cardiac auscultatory skills of internal medicine and family practice trainees: a comparison of diagnostic proficiency.JAMA.1997;278:717–722. , .
- Detection and correction of house staff error in physical diagnosis.JAMA.1983;249:1035–1037. , .
- Is listening through a stethoscope a dying art?Boston Globe May 25,2004. .
- Salvaging the history, physical examination and doctor‐patient relationship in a technological cardiology environment.J Am Coll Cardiol.1999;33:892–893. , .
- Wither the Cardiac Physical Examination?J Am Coll Cardiol.2006;48:2156–2157. .
- New techniques should enhance, not replace, bedside diagnostic skills in cardiology, Part 1.Mod Concepts Cardiovasc Dis.1990;59:19–24. , .
- New techniques should enhance, not replace, bedside diagnostic skills in cardiology, Part 2.Mod Concepts Cardiovasc Dis.1990;59:25–30. , .
- Time, now, to recover the fun in the physical examination rather than abandon it.Arch Intern Med.2006;166:603–604. .
- On bedside teaching.Ann Intern Med.1997;126:217–220. .
- Cassie JM, Daggett CJ. The role of the attending physician in clinical training.J Med Educ.1978;53:429–431. .
- Teaching the resident in infernal medicine: present practices and suggestions for the future.JAMA.1986:256:725–729. . .
- Bedside rounds revisited.N Engl J Med.1997;336:1174–1175. .
- Effects of training in direct observation of medical residents' clinical competence a randomized trial.Ann Intern Med.2004;140:874–881. , , .
- American Board of Internal Medicine. Clinical Skills/PESEP. Available at: http://www.abim.org/moc/semmed.shtm. Accessed July 9,2007.
- Accreditation Council for Graduate Medical Education (ACGME). General Competencies and Outcomes Assessment for Designated Institutional Officials. Available at: http://www.acgme.org/outcome/comp/compFull.asp. Accessed July 9,2007.
- Accreditation Council for Graduate Medical Education, Resident Duty Hours. Available at: http://www.acgme.org/acWebsite/dutyHours/dh_dutyHoursCommonPR.pdf. Accessed, July 9,2007.
- Letter to the Editor re: Cardiac auscultatory skills of physicians‐in‐training: comparison of three English‐speaking countries.Am J Med.2001;111:505–506. .
- Assessing housestaff diagnostic skills using a cardiology patient simulator.Ann Intern Med.1992;117:751–756. , , , et al.
- A comparison of computer assisted instruction and small group teaching of cardiac auscultation to medical students.Med Educ.1991;25:389–395. , , , .
- Validation of a multimedia measure of cardiac physical examination proficiency.Boston, MA:Association of American Medical Colleges Group on Educational Affairs, Research in Medical Education Summary Presentations;November,2004. , , , , .
- Validation of a computerized test to assess competence in the cardiac physical examination. Submitted for publication,2007. , , , , .
- Blaufuss Medical Multimedia Laboratory. Heart sounds tutorial. Available at: http://www.blaufuss.org. Accessed July 7,2007.
- 30th Bethesda Conference: The Future of Academic Cardiology. Task force 3: teaching.J Am Coll Cardiol.1999;33:1120–1127. , .
- Beyond Heart Sounds: An interactive teaching and skills testing program for cardiac examination.Comput Cardiol.2000;27:591–594. , , .
- Using virtual patients to improve cardiac examination competency in medical students. In press,Clin Cardiol. , , , , .
- Accuracy of physician self‐assessment compared with observed measures of competence; a systematic review.JAMA.2006;296: 9;1094–1102. , , , , , .
- Assessing the ACGME general competencies: general considerations and assessment methods.Acad Emerg Med.2002;9:1278–1288. .
Copyright © 2008 Society of Hospital Medicine
Purpuric Rash of Meningococcemia
A previously healthy 62‐year‐old man presented to the emergency department with 4 days of headache, fever, and chills. Within several hours of presentation, he developed septic shock. His temperature was 39C, and his white blood count was 17,000/mm3 with 38% bands. He had acute renal failure (creatinine = 2.5) and mild mental status changes. His blood pressure decreased from 103/63 to 71/46 and was not responsive to intravenous fluid administration. He therefore was begun on pressors. Eight hours after arrival, a petechial and purpuric rash suddenly appeared on the patient's extremities, including the palms of his hands (Fig. 1). Ceftriaxone was added to his initial antibiotics (vancomycin and pipercillin‐tazobactam), and he was treated with stress‐dose hydrocortisone and activated drotrecogin alpha. The following day, blood cultures grew gram‐negative diplococci in pairs, and preventive measures were taken, including treating close contacts and placing the patient under droplet precautions. The cultures eventually confirmed Neisseria meningitidis. The patient made excellent progresshis mental status improved, he was weaned off pressors after 3 days, and his renal failure resolved. Figure 2 demonstrates the evolution of the purpuric lesions, which became more prominent as he otherwise made clinical improvement. He was discharged home after 2 weeks of intravenous ceftriaxone in good condition.
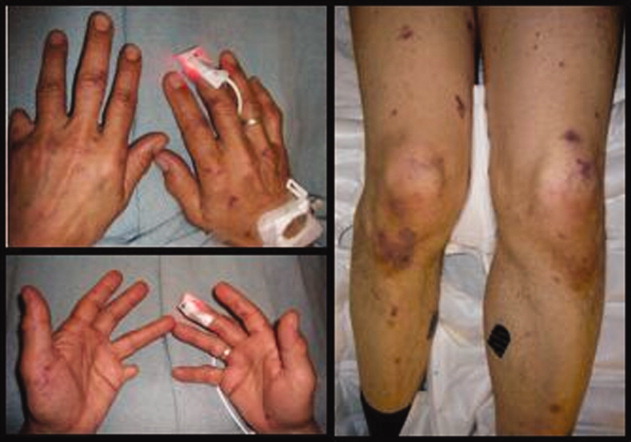
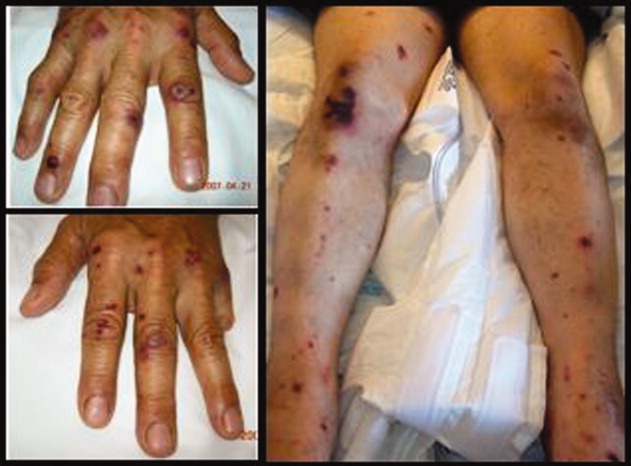
A previously healthy 62‐year‐old man presented to the emergency department with 4 days of headache, fever, and chills. Within several hours of presentation, he developed septic shock. His temperature was 39C, and his white blood count was 17,000/mm3 with 38% bands. He had acute renal failure (creatinine = 2.5) and mild mental status changes. His blood pressure decreased from 103/63 to 71/46 and was not responsive to intravenous fluid administration. He therefore was begun on pressors. Eight hours after arrival, a petechial and purpuric rash suddenly appeared on the patient's extremities, including the palms of his hands (Fig. 1). Ceftriaxone was added to his initial antibiotics (vancomycin and pipercillin‐tazobactam), and he was treated with stress‐dose hydrocortisone and activated drotrecogin alpha. The following day, blood cultures grew gram‐negative diplococci in pairs, and preventive measures were taken, including treating close contacts and placing the patient under droplet precautions. The cultures eventually confirmed Neisseria meningitidis. The patient made excellent progresshis mental status improved, he was weaned off pressors after 3 days, and his renal failure resolved. Figure 2 demonstrates the evolution of the purpuric lesions, which became more prominent as he otherwise made clinical improvement. He was discharged home after 2 weeks of intravenous ceftriaxone in good condition.
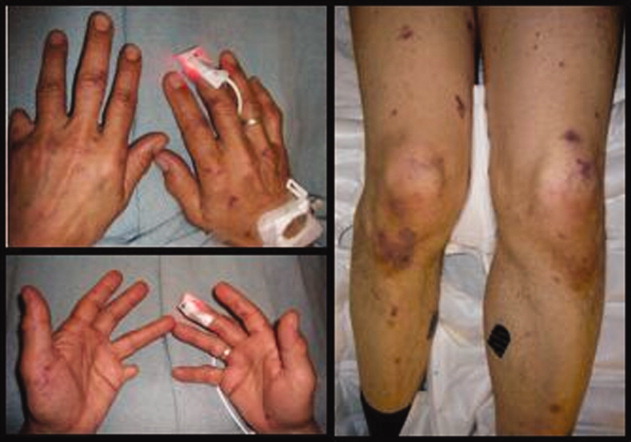
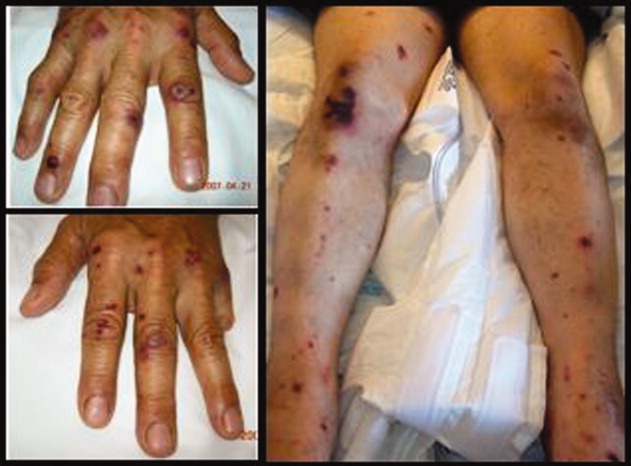
A previously healthy 62‐year‐old man presented to the emergency department with 4 days of headache, fever, and chills. Within several hours of presentation, he developed septic shock. His temperature was 39C, and his white blood count was 17,000/mm3 with 38% bands. He had acute renal failure (creatinine = 2.5) and mild mental status changes. His blood pressure decreased from 103/63 to 71/46 and was not responsive to intravenous fluid administration. He therefore was begun on pressors. Eight hours after arrival, a petechial and purpuric rash suddenly appeared on the patient's extremities, including the palms of his hands (Fig. 1). Ceftriaxone was added to his initial antibiotics (vancomycin and pipercillin‐tazobactam), and he was treated with stress‐dose hydrocortisone and activated drotrecogin alpha. The following day, blood cultures grew gram‐negative diplococci in pairs, and preventive measures were taken, including treating close contacts and placing the patient under droplet precautions. The cultures eventually confirmed Neisseria meningitidis. The patient made excellent progresshis mental status improved, he was weaned off pressors after 3 days, and his renal failure resolved. Figure 2 demonstrates the evolution of the purpuric lesions, which became more prominent as he otherwise made clinical improvement. He was discharged home after 2 weeks of intravenous ceftriaxone in good condition.
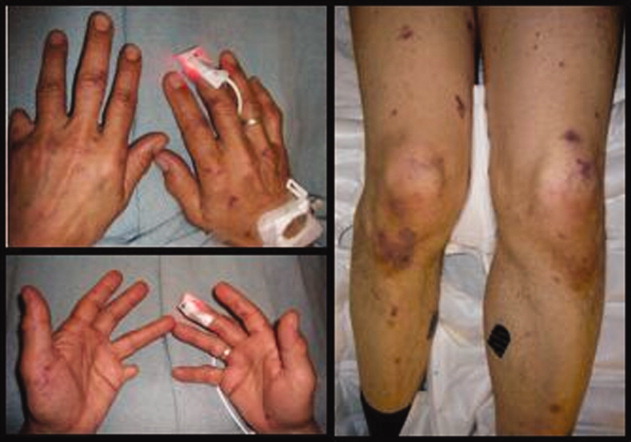
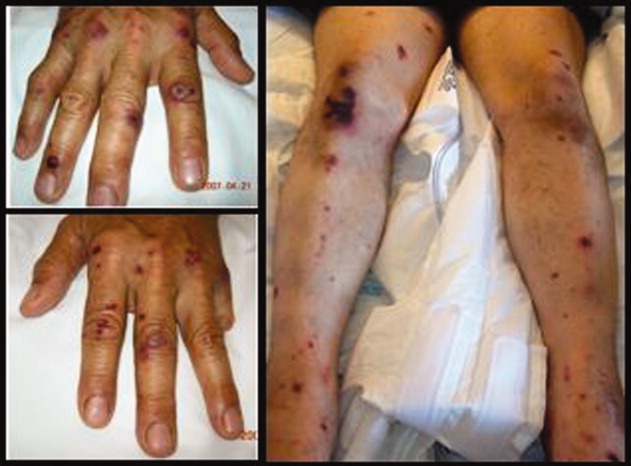
Increased DVT Prophylaxis Resulting in Decreased Hospital‐Acquired DVT
Hospital‐acquired venous thromboembolic events (VTEs) in medically ill patients account for a significant percentage of in‐hospital mortality.1 There have been reports that 50%75% of symptomatic VTEs related to hospitalization occur in medical patients.2, 3 The incidence of hospital‐acquired pulmonary embolism (PE) and deep vein thrombosis (DVT) has been reported nationally as 0.4% and 1.3% of all hospital admissions, respectively.4 The incidence of hospital‐acquired VTEs in patients not on prophylaxis reported in the MEDENOX trial was approximately 15%, although these were predominantly asymptomatic cases.5
Several studies including the MEDENOX and PREVENT trials have supported the use of low‐molecular‐weight heparin (LMWH) and unfractionated heparin (UFH) in the prevention of VTEs.57 Based on this evidence, the American College of Chest Physicians (ACCP) developed guidelines for the use of LMWH and UFH in the prevention of VTEs in patients with acute medical illnesses.8 Despite the promulgation of these guidelines, several studies have indicated that the use of these medications remains suboptimal. Two recent studies showed that the rate of thromboprophylaxis in hospitalized medically ill patients at risk for VTEs was 15%16%.9, 10
A review by Kakkar et al. stated that the underutilization of thromboprophylaxis may be a result of lack of awareness by physicians, disagreement with the guidelines, and lack of outcome data.11 Studies have demonstrated improvement in the use of thromboprophylaxis in hospitalized patients using several strategies. One of these strategies was the use of a hospitalwide clinical pharmacy education program, resulting in the thromboprophylaxis rate improving from 11% to 44% (P < .001).12 Another strategy studied was the use of a combination of physician education, a decision support tool, and regular audit and feedback, which resulted in the rate of thromboprophylaxis increasing from 43% to 85% after 18 months.13 The use of computer alert programs was also studied and was shown to increase the use of thromboprophylaxis among hospitalized patients from 13% to 23.6% (P < 0.001).14
The objective of this article is to report the impact of a continuous quality improvement (CQI) project on adherence to the DVT prophylaxis guidelines as well as the subsequent incidence of hospital‐acquired DVT in medical patients at a large urban teaching hospital between 2002 and 2005.
METHODS
In November 2002, the Kings County Hospital Center Department of Medicine embarked on a CQI project to increase adherence to thromboprophylaxis guidelines. A 3‐tiered approach of provider education, provider reminders with decision support, and audit and feedback was taken. This cycle was repeated each month with the start of a new group of house staff and faculty attendings. This 3‐tiered approach was developed, implemented, and maintained over a 4‐year period from 2002 to 2005. The measured outcomes were rate of DVT prophylaxis and incidence of hospital‐acquired DVT.
Provider Education and Reminders with Decision Support
The first approach was the inclusion of DVT prophylaxis in the Assessment and Plan section of the preprinted admission database. This section on DVT prophylaxis, which required a physician to indicate if a patient required prophylaxis and the type of prophylaxis chosen, was initiated in November 2002 (Fig. 1, arrow A).
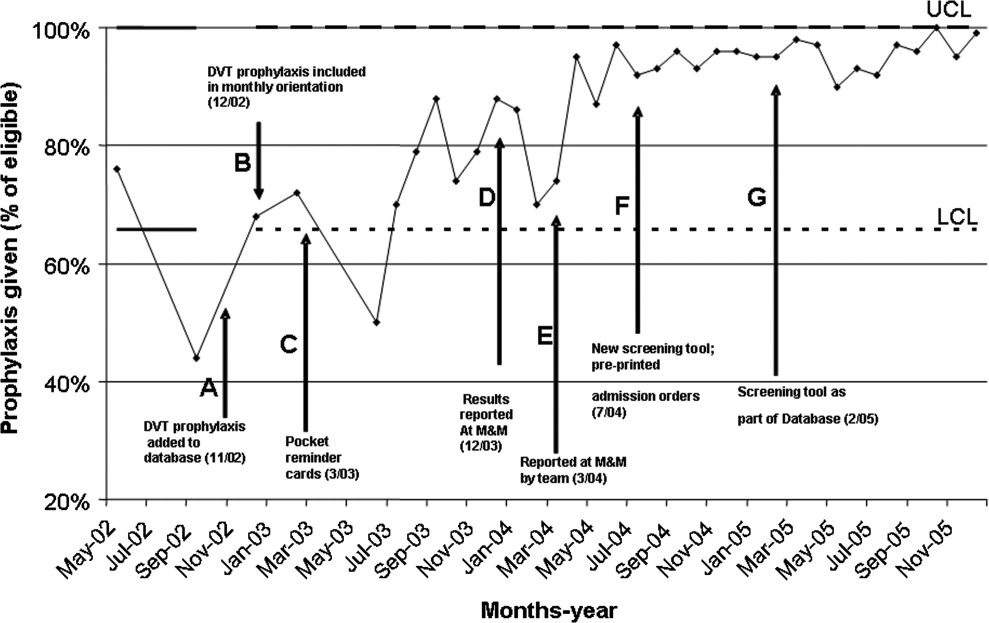
In December 2002, DVT prophylaxis was included in the learning goals and objectives handout given to house staff at the start of each inpatient rotation (Fig. 1, arrow B).
Pocket DVT prophylaxis guideline cards that outlined the indications and suggested regimens for DVT prophylaxis in the medically ill patient were issued to members of the house staff and their supervising faculty attendings at the start of each month beginning in March 2003 (Fig. 1, arrow C).
Preprinted admission orders were developed in July 2004 (Fig. 1, arrow F) that included DVT prophylaxis options. The admitting physician was required to indicate the need for DVT prophylaxis and type of prophylaxis chosen for every patient admitted.
In February 2005, a standardized DVT algorithm was included in the preprinted admission database (Figs. 13). The admitting physician was required to follow this algorithm to assess for indications for DVT prophylaxis and, if needed, type of prophylaxis chosen. The signatures of the house officer who completed the form and the supervising attending were required.
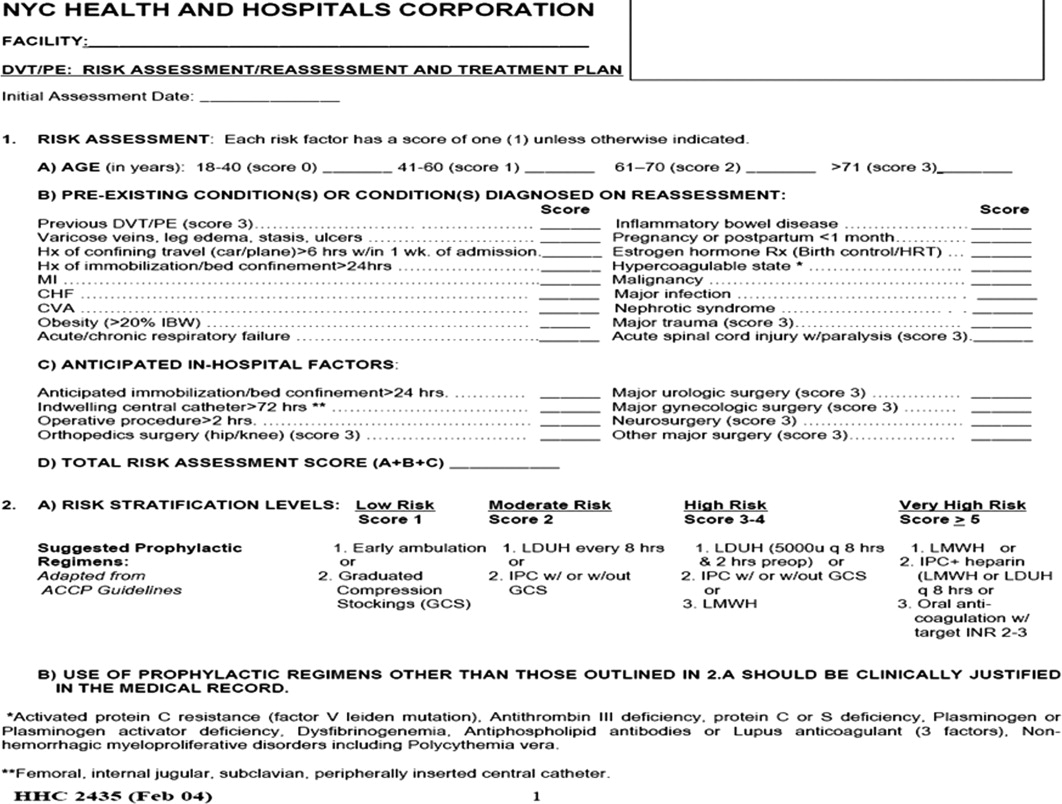
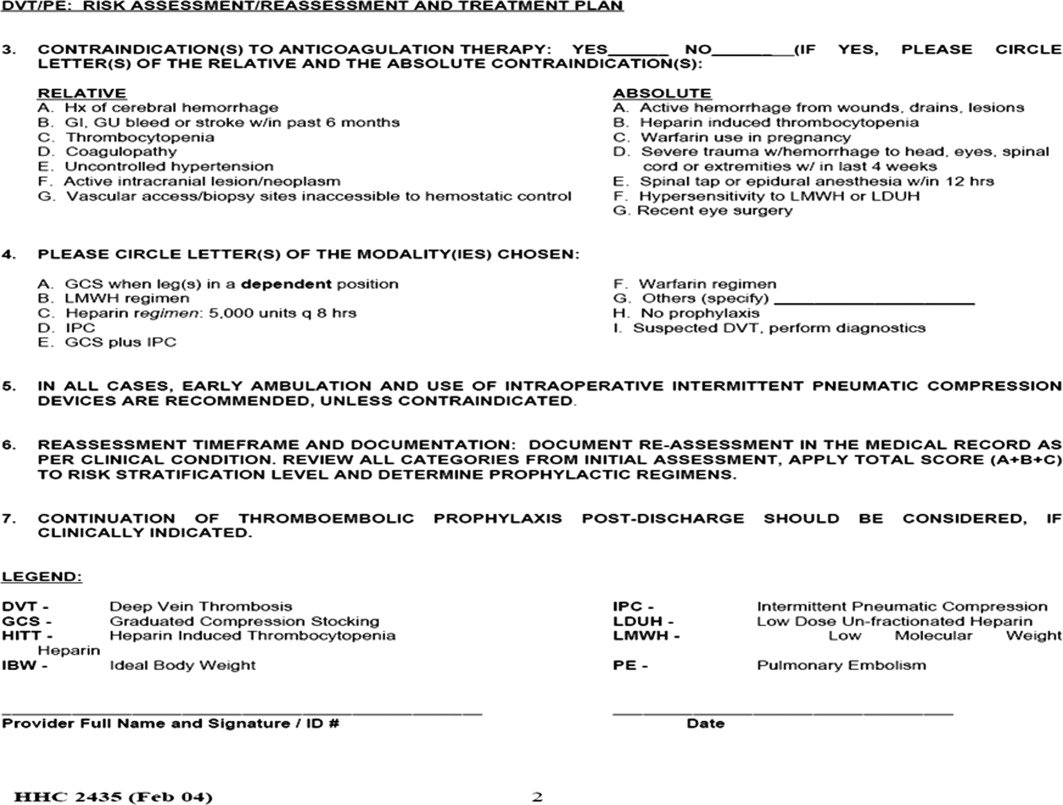
Auditing
Starting in December 2003, each house‐staff medicine service team was assessed for its rate of DVT prophylaxis on a monthly basis. Two chief residents would review 7 randomly selected charts from each of the 10 general medicine house‐staff teams. The data collected included number of patients at increased risk of developing DVT who were receiving DVT prophylaxis defined as UFH, LMWH, and/or intermittent pneumatic compression. Patients were considered at increased risk of developing DVT if they had heart failure, myocardial infarction, cancer, systemic infection, a hypercoagulable state, respiratory failure, chronic obstructive pulmonary disease, pulmonary hypertension, hip or other lower extremity fracture, stroke, catatonia, or a history of previous DVT or PE. In addition, patients for whom bed rest was ordered or who were expected or observed to be immobile (ie, not get out of bed) were considered at increased risk of DVT. The DVT prophylaxis rate was the percentage of patients identified as at increased risk of developing DVT who were receiving DVT prophylaxis. The reviewers used standardized review sheets to collect the data. Each month they reviewed a total of 70 charts, which represented approximately 14% of all medicine house‐staff discharges and approximately 60% of the average daily census of the house‐staff service.
Hospital‐acquired DVT cases were actively identified and reviewed independently by the Department of Risk Management. This activity resulted from the requirement to report all hospital‐acquired DVTs to the New York State Department of Health Patient Outcome Reporting Tracking System. The risk management staff systematically reviewed all discharge International Classification of Diseases Ninth Edition (ICD‐9) codes, as well as the reports of all duplex scans of the upper and lower extremities. A review of the medical record was triggered by report of a DVT on an imaging study or the finding of either ICD‐9 code 453.8 (embolism and thrombosis of the vein) or ICD‐9 code 453.9 (embolism and thrombosis of unspecified site). The purpose of the chart review was to confirm the diagnosis of DVT and to determine if the DVT was indeed hospital acquired. Hospital‐acquired DVTs were defined as those that developed while admitted or within 30 days of discharge from Kings County Hospital Center. Developed during admission was defined as a diagnosis of DVT not being suspected at original presentation at the hospital. If DVT was suspected on admission and the patient had not been hospitalized at Kings County Hospital Center in the preceding 30 days, the case was excluded.
Feedback
Reporting of the DVT prophylaxis rate was initiated in December 2003 (Fig. 1, arrow D). During the last week of each month, the rate of DVT prophylaxis on the general medicine house‐staff service was reported at the morbidity and mortality (M&M) conference. Attendance at the M&M conference was expected of all members of the house staff and faculty attendings assigned to the general medicine service for that particular month. The conference was chaired by the chief medical residents and was attended by the chief of the department of medicine and the director of inpatient services, who actively supported the project and acknowledged the importance of DVT prophylaxis.
Starting in March 2004, team‐specific DVT prophylaxis rates were reported at the monthly M&M conference rather than a single rate for the entire house‐staff service (Fig. 1, arrow E). This feedback enabled assessment of individual team performance and created a sense of competition. Each team was composed of an attending physician, a postgraduate year 3 resident, and a postgraduate year 1 resident. The feedback was presented as a focal point of the conference each month, one of the department's continuous quality improvement measures. The annual hospital‐acquired DVT rates observed from 2002 onward were also reported at this conference. Opportunity was given for discussion, comments, and feedback at the end of each conference, and occasionally new ideas for improvement were generated.
Statistical Analysis
A generalized linear model was used to estimate 95% confidence intervals for annual DVT incidence rates and to compare rates for 20032005 with that of 2002. Bonferroni corrections were applied to P values in order to control the overall type I error rate. Calendar year was the only independent variable in the analysis.
RESULTS
Table 1 provides cumulative yearly data for DVT prophylaxis rate, number of hospital‐acquired DVTs, and number of discharges from the general medicine house‐staff service for the 4 years of the observational period, 20022005. The baseline DVT prophylaxis rate from May 2002 to December 2002 was 63% (Fig. 4 and Table 1) and increased to 73%, 90%, and 96% over the succeeding years. In 2002, the number of hospital‐acquired DVTs on the general medicine house‐staff service was 14, followed by 16, 7, and 1 for 2003 through 2005 (Table 1). Twenty‐four of these 38 cases had not received DVT prophylaxis.
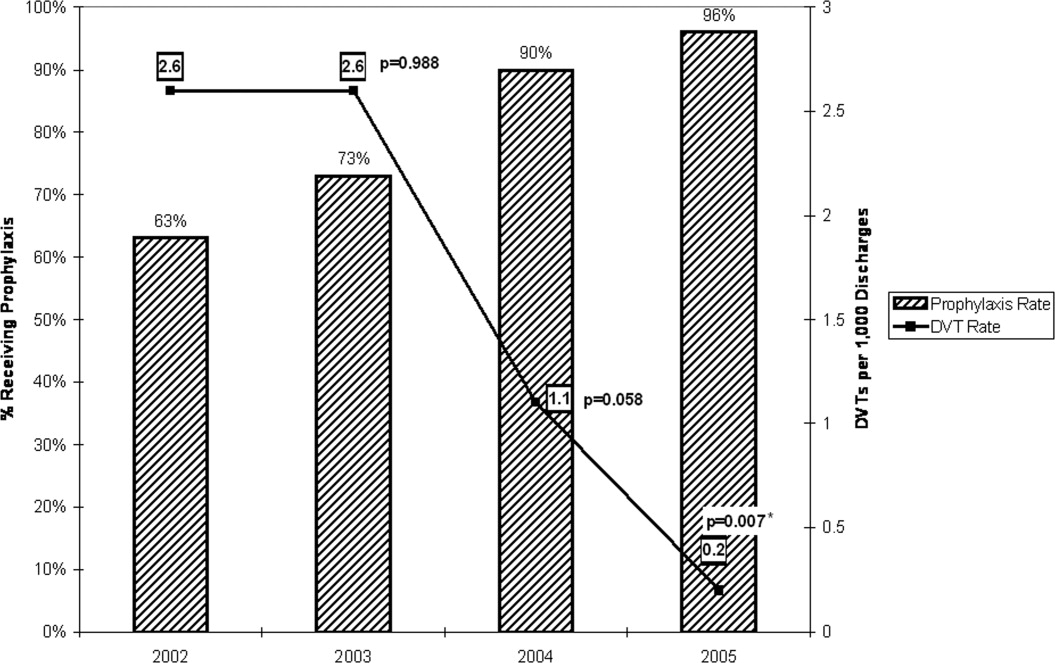
Year | DVT prophylaxis rate | Hospital‐acquired DVTs (n) | Discharges (n) | Hospital‐acquired DVT rate (DVTs per 1000 discharges) | 95% Confidence interval | P value |
---|---|---|---|---|---|---|
| ||||||
2002 (Year 1) | 63% | 14 | 5366 | 2.6 | 1.54.4 | |
2003 (Year 2) | 73% | 16 | 6098 | 2.6 | 1.64.3 | .988 |
2004 (Year 3) | 90% | 7 | 6460 | 1.1 | 0.52.3 | .058 |
2005 (Year 4) | 96% | 1 | 6296 | 0.2 | 0.01.1 | .007* |
When adjusted for each 1000 discharges (Fig. 4 and Table 1), the rates of hospital‐acquired DVT significantly decreased, from 2.6 per 1000 discharges (95% CI 1.54.4) in 2002 to 1.1 per 1000 discharges (95% CI 0.52.3, P = .058) in 2004 and to 0.2 per 1000 discharges (95% CI 0.01.1, P = .007) in 2005. During these years, particularly 20042005, the monthly DVT prophylaxis rate in the sample reviewed was consistently 90% or better (Fig. 1).
DISCUSSION
Our study involved active multifaceted interventions with a layered combination of provider education, provider reminders with decision support, and audit and feedback. This layered approach increased the DVT prophylaxis rate in our department, resulting in a significant decline in clinically evident hospital‐acquired DVTs from a baseline rate of 2.6 per 1000 discharges (0.26%) in 2002 to a rate of 0.2 per 1000 discharges (0.02%) in 2005 (95% CI 0.01.1, P = .007). Our baseline rate was low compared to the nationally reported incidence of 1.3%.4 Had the study not been extended over 4 years, it is likely that the statistical significance of this decline would have been missed. The rate of decrease in hospital0acquired DVTs accelerated with each year, showing no decline between 2002 and 2003, a 58% decline from 2003 to 2004, and an 82% decline from 2004 to 2005.
The acceleration in this decline coincided with the use of pocket DVT prophylaxis guideline cards and monthly audits with feedback starting in 2003 and the implementation of preprinted admission orders in 2004. This acceleration peaked in 2005 with the addition of the DVT algorithm to the admission database.
Despite the ACCP guidelines on thromboprophylaxis,8 several studies have suggested that only approximately 15%16% of medically ill patients at increased risk of VTE receive adequate thromboprophylaxis.9, 10 The 3 barriers identified by Kakkar et al. were lack of physician awareness, disagreement with the guidelines, and lack of outcome data.11 Our interventions addressed these barriers. Lack of physician awareness was addressed by provider education and reminder systems with decision support. Audit with team‐specific feedback provided the outcome data needed to demonstrate and reinforce effective change.
A critical analysis by Shojania et al. analyzed the effectiveness of each of these strategies when implemented alone. Provider education was effective in increasing provider knowledge but was generally ineffective when judged on the basis of improving patient outcomes. When provider reminders were well integrated with work flow, they were more likely to be effective. The effectiveness of decision support was variable, as it sometimes brought about change but was less likely to do so in complex situations. Various forms of audit and feedback produced small to modest effective changes.15 A systematic review by Oxman et al. suggested that there were some benefits when these strategies were implemented in a layered manner compared with single‐faceted strategies and that effective interventions were more likely to involve active rather than passive strategies.16
The major strengths of this study were the large number of patients reviewed, the sustained interventions over a 4‐year period, and the consistency of our results. The Department of Risk Management systematically reviewed all discharge ICD‐9 codes and all relevant imaging studies of 32,293 patients for the presence of hospital‐acquired DVTs. The data provided was all‐inclusive regardless of outcome and was acquired continuously over a 4‐year period rather than at a single point.
Our department continues to seek methods to increase the rate of thromboprophylaxis, and so, noting that computer alert programs have been shown to increase the appropriate use of thromboprophylaxis,14 our institution has designed an admission computer order entry program specific for DVT prophylaxis. This program prompts physicians to perform a DVT risk assessment of each admitted patient and to order the appropriate thromboprophylaxis regimen if indicated. This program was implemented in May 2006.
Limitations
Several weaknesses are inherent in a before‐and‐after study such as ours. These include the presence of background factors that can produce significant changes in processes or outcomes of interest regardless of quality improvement interventions. Another weakness is that for any given period studied, multiple unaccounted changes typically occur in a health care system and in its socioeconomic environment that might also produce the desired improvements.17 An attempt was made to mitigate the presence of background factors by including a large number of patients in our analysis, by conducting the study over 4 years rather than over a shorter period, and by analyzing data continuously throughout each year rather than at a single point. These weaknesses could be further ameliorated by a controlled before‐and‐after study design in which there would be analysis of data from 2 hospitals, one that implemented the quality improvement interventions and one that did not.
Several authority gradients were used in this initiative, including the supervising faculty attending assigned to each medical team, who was required to cosign each of the DVT risk assessment sheets in the admitting database, providing a powerful tool in the promotion of provider reminder and decision support in this initiative. The other authority gradients included the chief of medicine and the director of inpatient services, who both presided over the monthly morbidity and mortality conferences where performance feedback of the initiative was presented. The authority gradients at these performance feedback sessions were useful in promoting discussion and gaining valuable feedback, as well as in garnering ideas for improvement from the house staff. However, although these authority gradients were important in carrying out this initiative, having them may not be possible at other institutions, particularly nonacademic hospitals, and as such could potentially limit reproducibility.
Another limitation of our study is that we included only patients with DVT. Patients with PE without an identified DVT were not included. In addition, there was no specific, systematic review of complications such as heparin‐induced thrombocytopenia (HIT) and bleeding. However, our departmental and hospitalwide quality improvement programs do attempt to identify complications of treatment and adverse drug reactions. There was no observed increase in bleeding complications and only 2 cases of HIT were identified in our department during the 4‐year period studied. Reported rates of HIT and bleeding in medical patients receiving LMWH or UFH for DVT prophylaxis have been low.57, 18
The DVT cases identified from 2002 to 2005 were those that were clinically relevant. Asymptomatic, clinically silent cases were not included in this report because routine screening for subclinical DVT is not presently a part of routine general medicine inpatient care.
CONCLUSIONS
The multifaceted layered combination of provider education, provider reminders with decision support, and audit and feedback implemented at our institution could be reproduced and utilized at other institutions, particularly teaching hospitals, to address the underutilization of DVT prophylaxis in medically ill patients and to bring about a decrease in hospital‐acquired DVTs.
- Fatal pulmonary embolism in hospitalised patients: a necropsy review.J Clin Pathol.2004;57:1254–1257. , , , .
- New onset of venous thromboembolism among hospitalized patients at Brigham and Women's Hospital is caused more often by prophylaxis failure than by withholding treatment.Chest.2000;118:1680–1684. , , .
- Preventing venous thromboembolism in medical patients.Circulation.2004;110(24 Suppl 1):IV13–IV19. , .
- Trends in the incidence of pulmonary embolism and deep venous thrombosis in hospitalized patients.Am J Cardiol.2005;95:1525–1526. , , .
- A comparison of enoxaparin with placebo for the prevention of venous thromboembolism in acutely ill medical patients.N Engl J Med.1999;341:793–800. , , , et al.
- Prevention of venous thromboembolism in internal medicine with unfractionated or low‐molecular‐weight heparins: a meta‐analysis of randomised clinical trials.Thromb Haemost.2000;83(1):14–19. , , , et al.
- Randomized, placebo‐controlled trial of dalteparin for the prevention of venous thromboembolism in acutely ill medical patients.Circulation.2004;110:874–879. , , , et al.
- Prevention of venous thromboembolism: the Seventh ACCP Conference on Antithrombotic and Thrombolytic Therapy.Chest.2004;126(3 Suppl):338S–400S. , , , et al.
- Hospitals' compliance with prophylaxis guidelines for venous thromboembolism.Am J Health Syst Pharm.2007;64(1):69–76. , , , .
- Multicenter evaluation of the use of venous thromboembolism prophylaxis in acutely ill medical patients in Canada.Thromb Res.2007;119(2):145–155. , , , et al.
- Compliance with recommended prophylaxis for venous thromboembolism: improving the use and rate of uptake of clinical practice guidelines.J Thromb Haemost.2004;2:221–227. , , .
- Effect of a clinical pharmacy education program on improvement in the quantity and quality of venous thromboembolism prophylaxis for medically ill patients.J Manag Care Pharm.2005;11:755–762. , .
- Improved use of thromboprophylaxis for deep vein thrombosis following an educational intervention.J Hosp Med.2006;1:331–338. , , .
- Electronic alerts to prevent venous thromboembolism among hospitalized patients.N Engl J Med.2005;352:969–977. , , , et al.
- Closing the Quality Gap: A Critical Analysis of Quality Improvement Strategies.Rockville, MD:Agency for Healthcare Research and Quality;2004. , , , .
- No magic bullets: a systematic review of 102 trials of interventions to improve professional practice.CMAJ.1995;153:1423–1431. , , , .
- Evidence‐based quality improvement: the state of the science.Health Aff (Millwood).2005;24(1):138–150. , .
- Incidence and economic implications of heparin‐induced thrombocytopenia in medical patients receiving prophylaxis for venous thromboembolism.Pharmacotherapy.2006;26:1438–1445. , , , .
Hospital‐acquired venous thromboembolic events (VTEs) in medically ill patients account for a significant percentage of in‐hospital mortality.1 There have been reports that 50%75% of symptomatic VTEs related to hospitalization occur in medical patients.2, 3 The incidence of hospital‐acquired pulmonary embolism (PE) and deep vein thrombosis (DVT) has been reported nationally as 0.4% and 1.3% of all hospital admissions, respectively.4 The incidence of hospital‐acquired VTEs in patients not on prophylaxis reported in the MEDENOX trial was approximately 15%, although these were predominantly asymptomatic cases.5
Several studies including the MEDENOX and PREVENT trials have supported the use of low‐molecular‐weight heparin (LMWH) and unfractionated heparin (UFH) in the prevention of VTEs.57 Based on this evidence, the American College of Chest Physicians (ACCP) developed guidelines for the use of LMWH and UFH in the prevention of VTEs in patients with acute medical illnesses.8 Despite the promulgation of these guidelines, several studies have indicated that the use of these medications remains suboptimal. Two recent studies showed that the rate of thromboprophylaxis in hospitalized medically ill patients at risk for VTEs was 15%16%.9, 10
A review by Kakkar et al. stated that the underutilization of thromboprophylaxis may be a result of lack of awareness by physicians, disagreement with the guidelines, and lack of outcome data.11 Studies have demonstrated improvement in the use of thromboprophylaxis in hospitalized patients using several strategies. One of these strategies was the use of a hospitalwide clinical pharmacy education program, resulting in the thromboprophylaxis rate improving from 11% to 44% (P < .001).12 Another strategy studied was the use of a combination of physician education, a decision support tool, and regular audit and feedback, which resulted in the rate of thromboprophylaxis increasing from 43% to 85% after 18 months.13 The use of computer alert programs was also studied and was shown to increase the use of thromboprophylaxis among hospitalized patients from 13% to 23.6% (P < 0.001).14
The objective of this article is to report the impact of a continuous quality improvement (CQI) project on adherence to the DVT prophylaxis guidelines as well as the subsequent incidence of hospital‐acquired DVT in medical patients at a large urban teaching hospital between 2002 and 2005.
METHODS
In November 2002, the Kings County Hospital Center Department of Medicine embarked on a CQI project to increase adherence to thromboprophylaxis guidelines. A 3‐tiered approach of provider education, provider reminders with decision support, and audit and feedback was taken. This cycle was repeated each month with the start of a new group of house staff and faculty attendings. This 3‐tiered approach was developed, implemented, and maintained over a 4‐year period from 2002 to 2005. The measured outcomes were rate of DVT prophylaxis and incidence of hospital‐acquired DVT.
Provider Education and Reminders with Decision Support
The first approach was the inclusion of DVT prophylaxis in the Assessment and Plan section of the preprinted admission database. This section on DVT prophylaxis, which required a physician to indicate if a patient required prophylaxis and the type of prophylaxis chosen, was initiated in November 2002 (Fig. 1, arrow A).
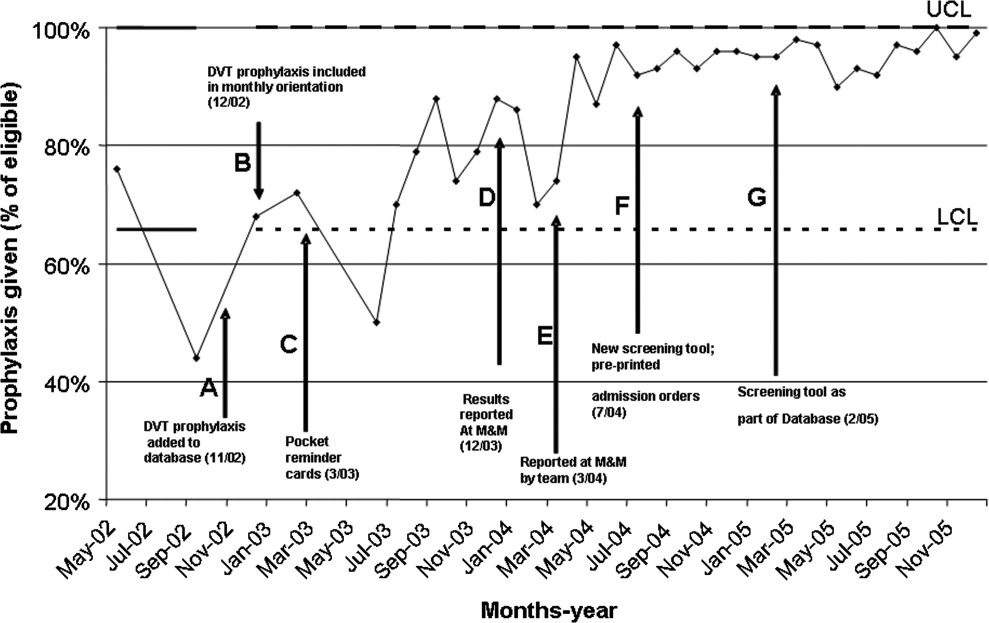
In December 2002, DVT prophylaxis was included in the learning goals and objectives handout given to house staff at the start of each inpatient rotation (Fig. 1, arrow B).
Pocket DVT prophylaxis guideline cards that outlined the indications and suggested regimens for DVT prophylaxis in the medically ill patient were issued to members of the house staff and their supervising faculty attendings at the start of each month beginning in March 2003 (Fig. 1, arrow C).
Preprinted admission orders were developed in July 2004 (Fig. 1, arrow F) that included DVT prophylaxis options. The admitting physician was required to indicate the need for DVT prophylaxis and type of prophylaxis chosen for every patient admitted.
In February 2005, a standardized DVT algorithm was included in the preprinted admission database (Figs. 13). The admitting physician was required to follow this algorithm to assess for indications for DVT prophylaxis and, if needed, type of prophylaxis chosen. The signatures of the house officer who completed the form and the supervising attending were required.
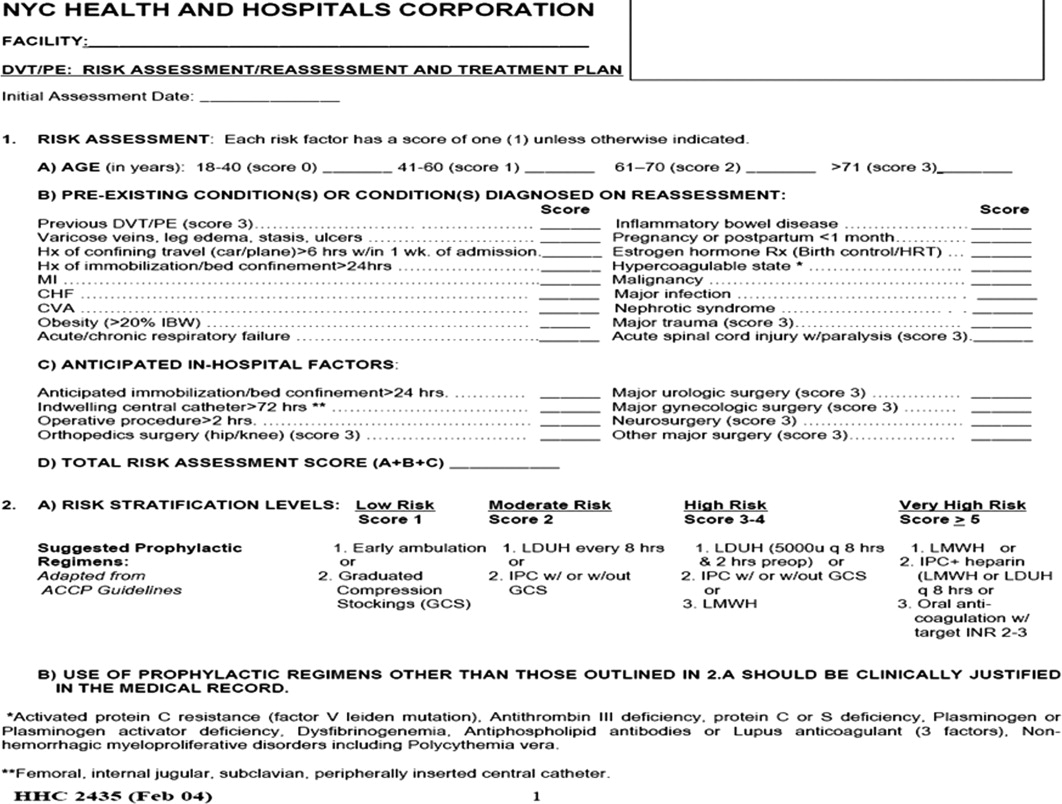
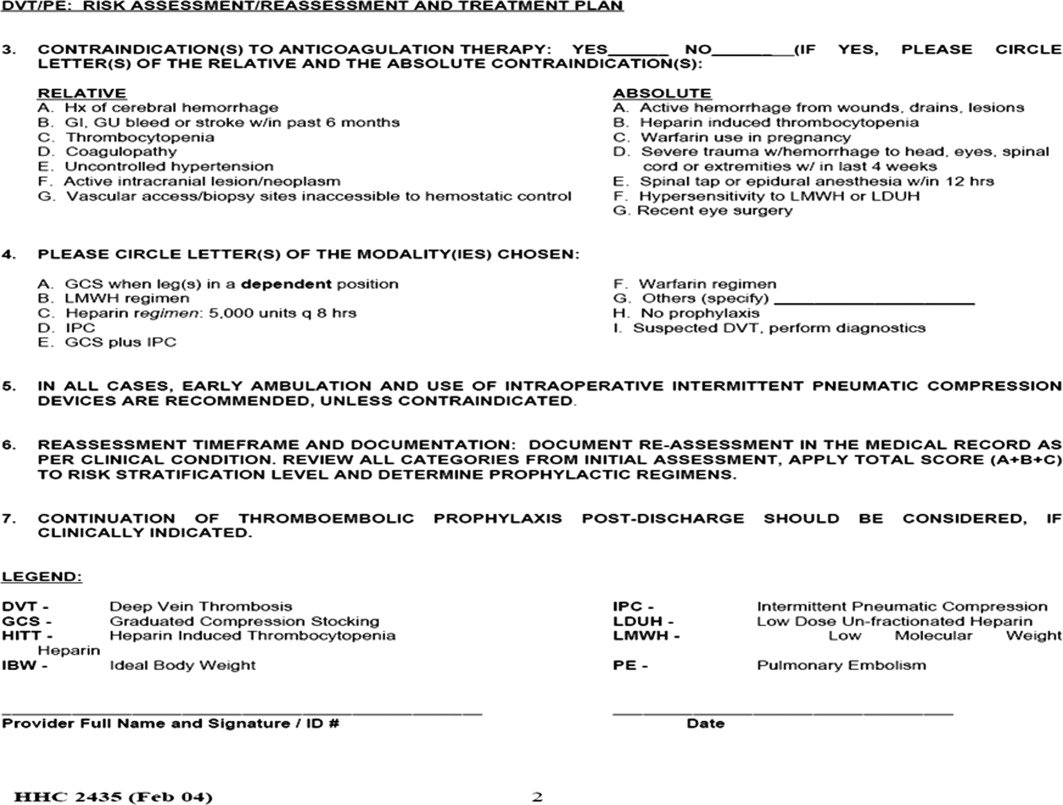
Auditing
Starting in December 2003, each house‐staff medicine service team was assessed for its rate of DVT prophylaxis on a monthly basis. Two chief residents would review 7 randomly selected charts from each of the 10 general medicine house‐staff teams. The data collected included number of patients at increased risk of developing DVT who were receiving DVT prophylaxis defined as UFH, LMWH, and/or intermittent pneumatic compression. Patients were considered at increased risk of developing DVT if they had heart failure, myocardial infarction, cancer, systemic infection, a hypercoagulable state, respiratory failure, chronic obstructive pulmonary disease, pulmonary hypertension, hip or other lower extremity fracture, stroke, catatonia, or a history of previous DVT or PE. In addition, patients for whom bed rest was ordered or who were expected or observed to be immobile (ie, not get out of bed) were considered at increased risk of DVT. The DVT prophylaxis rate was the percentage of patients identified as at increased risk of developing DVT who were receiving DVT prophylaxis. The reviewers used standardized review sheets to collect the data. Each month they reviewed a total of 70 charts, which represented approximately 14% of all medicine house‐staff discharges and approximately 60% of the average daily census of the house‐staff service.
Hospital‐acquired DVT cases were actively identified and reviewed independently by the Department of Risk Management. This activity resulted from the requirement to report all hospital‐acquired DVTs to the New York State Department of Health Patient Outcome Reporting Tracking System. The risk management staff systematically reviewed all discharge International Classification of Diseases Ninth Edition (ICD‐9) codes, as well as the reports of all duplex scans of the upper and lower extremities. A review of the medical record was triggered by report of a DVT on an imaging study or the finding of either ICD‐9 code 453.8 (embolism and thrombosis of the vein) or ICD‐9 code 453.9 (embolism and thrombosis of unspecified site). The purpose of the chart review was to confirm the diagnosis of DVT and to determine if the DVT was indeed hospital acquired. Hospital‐acquired DVTs were defined as those that developed while admitted or within 30 days of discharge from Kings County Hospital Center. Developed during admission was defined as a diagnosis of DVT not being suspected at original presentation at the hospital. If DVT was suspected on admission and the patient had not been hospitalized at Kings County Hospital Center in the preceding 30 days, the case was excluded.
Feedback
Reporting of the DVT prophylaxis rate was initiated in December 2003 (Fig. 1, arrow D). During the last week of each month, the rate of DVT prophylaxis on the general medicine house‐staff service was reported at the morbidity and mortality (M&M) conference. Attendance at the M&M conference was expected of all members of the house staff and faculty attendings assigned to the general medicine service for that particular month. The conference was chaired by the chief medical residents and was attended by the chief of the department of medicine and the director of inpatient services, who actively supported the project and acknowledged the importance of DVT prophylaxis.
Starting in March 2004, team‐specific DVT prophylaxis rates were reported at the monthly M&M conference rather than a single rate for the entire house‐staff service (Fig. 1, arrow E). This feedback enabled assessment of individual team performance and created a sense of competition. Each team was composed of an attending physician, a postgraduate year 3 resident, and a postgraduate year 1 resident. The feedback was presented as a focal point of the conference each month, one of the department's continuous quality improvement measures. The annual hospital‐acquired DVT rates observed from 2002 onward were also reported at this conference. Opportunity was given for discussion, comments, and feedback at the end of each conference, and occasionally new ideas for improvement were generated.
Statistical Analysis
A generalized linear model was used to estimate 95% confidence intervals for annual DVT incidence rates and to compare rates for 20032005 with that of 2002. Bonferroni corrections were applied to P values in order to control the overall type I error rate. Calendar year was the only independent variable in the analysis.
RESULTS
Table 1 provides cumulative yearly data for DVT prophylaxis rate, number of hospital‐acquired DVTs, and number of discharges from the general medicine house‐staff service for the 4 years of the observational period, 20022005. The baseline DVT prophylaxis rate from May 2002 to December 2002 was 63% (Fig. 4 and Table 1) and increased to 73%, 90%, and 96% over the succeeding years. In 2002, the number of hospital‐acquired DVTs on the general medicine house‐staff service was 14, followed by 16, 7, and 1 for 2003 through 2005 (Table 1). Twenty‐four of these 38 cases had not received DVT prophylaxis.
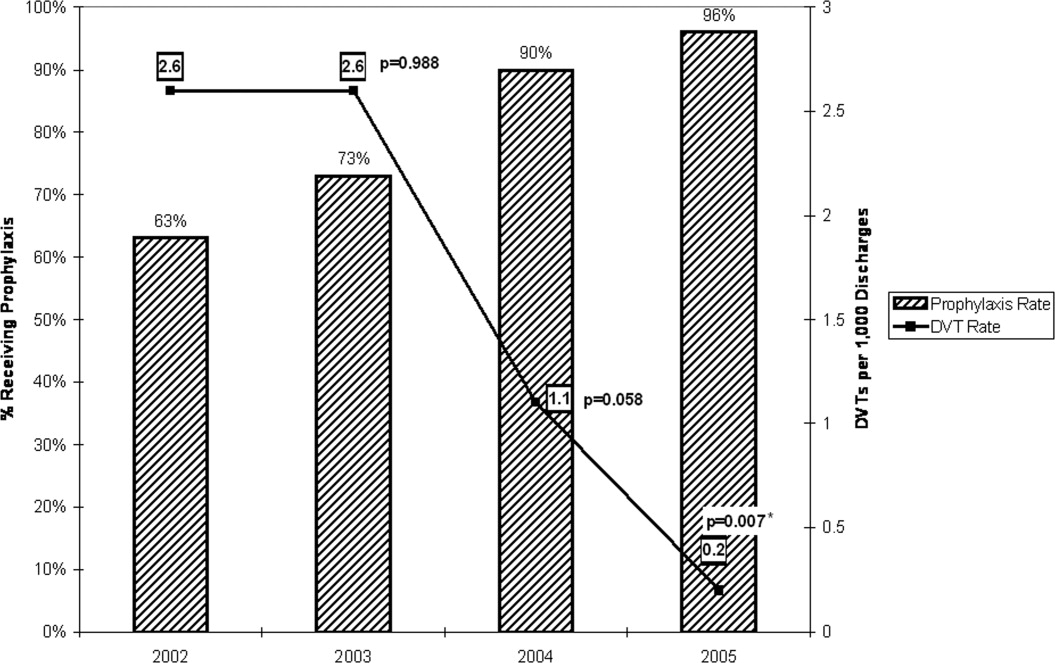
Year | DVT prophylaxis rate | Hospital‐acquired DVTs (n) | Discharges (n) | Hospital‐acquired DVT rate (DVTs per 1000 discharges) | 95% Confidence interval | P value |
---|---|---|---|---|---|---|
| ||||||
2002 (Year 1) | 63% | 14 | 5366 | 2.6 | 1.54.4 | |
2003 (Year 2) | 73% | 16 | 6098 | 2.6 | 1.64.3 | .988 |
2004 (Year 3) | 90% | 7 | 6460 | 1.1 | 0.52.3 | .058 |
2005 (Year 4) | 96% | 1 | 6296 | 0.2 | 0.01.1 | .007* |
When adjusted for each 1000 discharges (Fig. 4 and Table 1), the rates of hospital‐acquired DVT significantly decreased, from 2.6 per 1000 discharges (95% CI 1.54.4) in 2002 to 1.1 per 1000 discharges (95% CI 0.52.3, P = .058) in 2004 and to 0.2 per 1000 discharges (95% CI 0.01.1, P = .007) in 2005. During these years, particularly 20042005, the monthly DVT prophylaxis rate in the sample reviewed was consistently 90% or better (Fig. 1).
DISCUSSION
Our study involved active multifaceted interventions with a layered combination of provider education, provider reminders with decision support, and audit and feedback. This layered approach increased the DVT prophylaxis rate in our department, resulting in a significant decline in clinically evident hospital‐acquired DVTs from a baseline rate of 2.6 per 1000 discharges (0.26%) in 2002 to a rate of 0.2 per 1000 discharges (0.02%) in 2005 (95% CI 0.01.1, P = .007). Our baseline rate was low compared to the nationally reported incidence of 1.3%.4 Had the study not been extended over 4 years, it is likely that the statistical significance of this decline would have been missed. The rate of decrease in hospital0acquired DVTs accelerated with each year, showing no decline between 2002 and 2003, a 58% decline from 2003 to 2004, and an 82% decline from 2004 to 2005.
The acceleration in this decline coincided with the use of pocket DVT prophylaxis guideline cards and monthly audits with feedback starting in 2003 and the implementation of preprinted admission orders in 2004. This acceleration peaked in 2005 with the addition of the DVT algorithm to the admission database.
Despite the ACCP guidelines on thromboprophylaxis,8 several studies have suggested that only approximately 15%16% of medically ill patients at increased risk of VTE receive adequate thromboprophylaxis.9, 10 The 3 barriers identified by Kakkar et al. were lack of physician awareness, disagreement with the guidelines, and lack of outcome data.11 Our interventions addressed these barriers. Lack of physician awareness was addressed by provider education and reminder systems with decision support. Audit with team‐specific feedback provided the outcome data needed to demonstrate and reinforce effective change.
A critical analysis by Shojania et al. analyzed the effectiveness of each of these strategies when implemented alone. Provider education was effective in increasing provider knowledge but was generally ineffective when judged on the basis of improving patient outcomes. When provider reminders were well integrated with work flow, they were more likely to be effective. The effectiveness of decision support was variable, as it sometimes brought about change but was less likely to do so in complex situations. Various forms of audit and feedback produced small to modest effective changes.15 A systematic review by Oxman et al. suggested that there were some benefits when these strategies were implemented in a layered manner compared with single‐faceted strategies and that effective interventions were more likely to involve active rather than passive strategies.16
The major strengths of this study were the large number of patients reviewed, the sustained interventions over a 4‐year period, and the consistency of our results. The Department of Risk Management systematically reviewed all discharge ICD‐9 codes and all relevant imaging studies of 32,293 patients for the presence of hospital‐acquired DVTs. The data provided was all‐inclusive regardless of outcome and was acquired continuously over a 4‐year period rather than at a single point.
Our department continues to seek methods to increase the rate of thromboprophylaxis, and so, noting that computer alert programs have been shown to increase the appropriate use of thromboprophylaxis,14 our institution has designed an admission computer order entry program specific for DVT prophylaxis. This program prompts physicians to perform a DVT risk assessment of each admitted patient and to order the appropriate thromboprophylaxis regimen if indicated. This program was implemented in May 2006.
Limitations
Several weaknesses are inherent in a before‐and‐after study such as ours. These include the presence of background factors that can produce significant changes in processes or outcomes of interest regardless of quality improvement interventions. Another weakness is that for any given period studied, multiple unaccounted changes typically occur in a health care system and in its socioeconomic environment that might also produce the desired improvements.17 An attempt was made to mitigate the presence of background factors by including a large number of patients in our analysis, by conducting the study over 4 years rather than over a shorter period, and by analyzing data continuously throughout each year rather than at a single point. These weaknesses could be further ameliorated by a controlled before‐and‐after study design in which there would be analysis of data from 2 hospitals, one that implemented the quality improvement interventions and one that did not.
Several authority gradients were used in this initiative, including the supervising faculty attending assigned to each medical team, who was required to cosign each of the DVT risk assessment sheets in the admitting database, providing a powerful tool in the promotion of provider reminder and decision support in this initiative. The other authority gradients included the chief of medicine and the director of inpatient services, who both presided over the monthly morbidity and mortality conferences where performance feedback of the initiative was presented. The authority gradients at these performance feedback sessions were useful in promoting discussion and gaining valuable feedback, as well as in garnering ideas for improvement from the house staff. However, although these authority gradients were important in carrying out this initiative, having them may not be possible at other institutions, particularly nonacademic hospitals, and as such could potentially limit reproducibility.
Another limitation of our study is that we included only patients with DVT. Patients with PE without an identified DVT were not included. In addition, there was no specific, systematic review of complications such as heparin‐induced thrombocytopenia (HIT) and bleeding. However, our departmental and hospitalwide quality improvement programs do attempt to identify complications of treatment and adverse drug reactions. There was no observed increase in bleeding complications and only 2 cases of HIT were identified in our department during the 4‐year period studied. Reported rates of HIT and bleeding in medical patients receiving LMWH or UFH for DVT prophylaxis have been low.57, 18
The DVT cases identified from 2002 to 2005 were those that were clinically relevant. Asymptomatic, clinically silent cases were not included in this report because routine screening for subclinical DVT is not presently a part of routine general medicine inpatient care.
CONCLUSIONS
The multifaceted layered combination of provider education, provider reminders with decision support, and audit and feedback implemented at our institution could be reproduced and utilized at other institutions, particularly teaching hospitals, to address the underutilization of DVT prophylaxis in medically ill patients and to bring about a decrease in hospital‐acquired DVTs.
Hospital‐acquired venous thromboembolic events (VTEs) in medically ill patients account for a significant percentage of in‐hospital mortality.1 There have been reports that 50%75% of symptomatic VTEs related to hospitalization occur in medical patients.2, 3 The incidence of hospital‐acquired pulmonary embolism (PE) and deep vein thrombosis (DVT) has been reported nationally as 0.4% and 1.3% of all hospital admissions, respectively.4 The incidence of hospital‐acquired VTEs in patients not on prophylaxis reported in the MEDENOX trial was approximately 15%, although these were predominantly asymptomatic cases.5
Several studies including the MEDENOX and PREVENT trials have supported the use of low‐molecular‐weight heparin (LMWH) and unfractionated heparin (UFH) in the prevention of VTEs.57 Based on this evidence, the American College of Chest Physicians (ACCP) developed guidelines for the use of LMWH and UFH in the prevention of VTEs in patients with acute medical illnesses.8 Despite the promulgation of these guidelines, several studies have indicated that the use of these medications remains suboptimal. Two recent studies showed that the rate of thromboprophylaxis in hospitalized medically ill patients at risk for VTEs was 15%16%.9, 10
A review by Kakkar et al. stated that the underutilization of thromboprophylaxis may be a result of lack of awareness by physicians, disagreement with the guidelines, and lack of outcome data.11 Studies have demonstrated improvement in the use of thromboprophylaxis in hospitalized patients using several strategies. One of these strategies was the use of a hospitalwide clinical pharmacy education program, resulting in the thromboprophylaxis rate improving from 11% to 44% (P < .001).12 Another strategy studied was the use of a combination of physician education, a decision support tool, and regular audit and feedback, which resulted in the rate of thromboprophylaxis increasing from 43% to 85% after 18 months.13 The use of computer alert programs was also studied and was shown to increase the use of thromboprophylaxis among hospitalized patients from 13% to 23.6% (P < 0.001).14
The objective of this article is to report the impact of a continuous quality improvement (CQI) project on adherence to the DVT prophylaxis guidelines as well as the subsequent incidence of hospital‐acquired DVT in medical patients at a large urban teaching hospital between 2002 and 2005.
METHODS
In November 2002, the Kings County Hospital Center Department of Medicine embarked on a CQI project to increase adherence to thromboprophylaxis guidelines. A 3‐tiered approach of provider education, provider reminders with decision support, and audit and feedback was taken. This cycle was repeated each month with the start of a new group of house staff and faculty attendings. This 3‐tiered approach was developed, implemented, and maintained over a 4‐year period from 2002 to 2005. The measured outcomes were rate of DVT prophylaxis and incidence of hospital‐acquired DVT.
Provider Education and Reminders with Decision Support
The first approach was the inclusion of DVT prophylaxis in the Assessment and Plan section of the preprinted admission database. This section on DVT prophylaxis, which required a physician to indicate if a patient required prophylaxis and the type of prophylaxis chosen, was initiated in November 2002 (Fig. 1, arrow A).
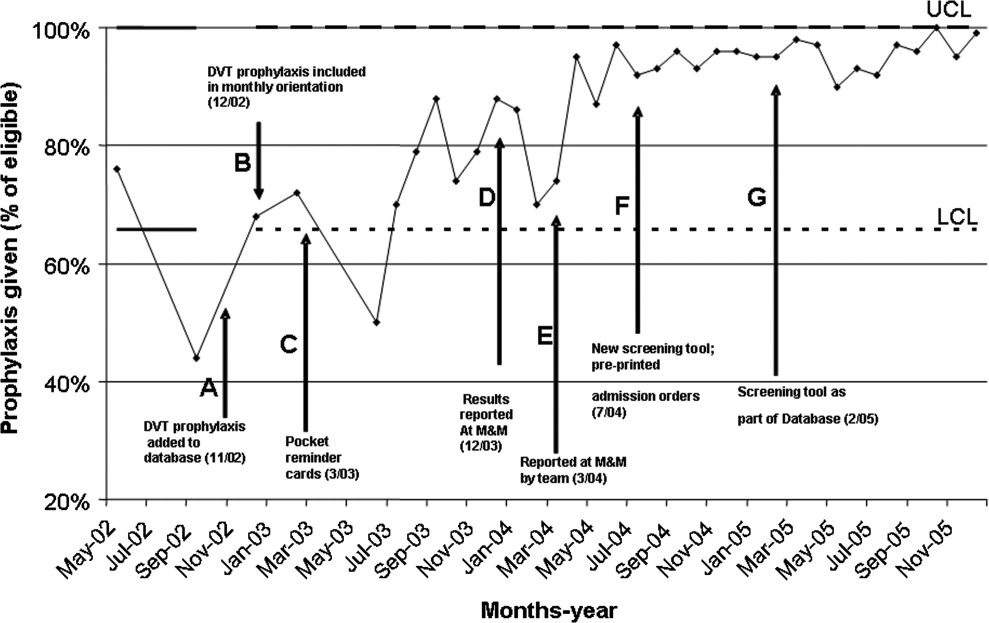
In December 2002, DVT prophylaxis was included in the learning goals and objectives handout given to house staff at the start of each inpatient rotation (Fig. 1, arrow B).
Pocket DVT prophylaxis guideline cards that outlined the indications and suggested regimens for DVT prophylaxis in the medically ill patient were issued to members of the house staff and their supervising faculty attendings at the start of each month beginning in March 2003 (Fig. 1, arrow C).
Preprinted admission orders were developed in July 2004 (Fig. 1, arrow F) that included DVT prophylaxis options. The admitting physician was required to indicate the need for DVT prophylaxis and type of prophylaxis chosen for every patient admitted.
In February 2005, a standardized DVT algorithm was included in the preprinted admission database (Figs. 13). The admitting physician was required to follow this algorithm to assess for indications for DVT prophylaxis and, if needed, type of prophylaxis chosen. The signatures of the house officer who completed the form and the supervising attending were required.
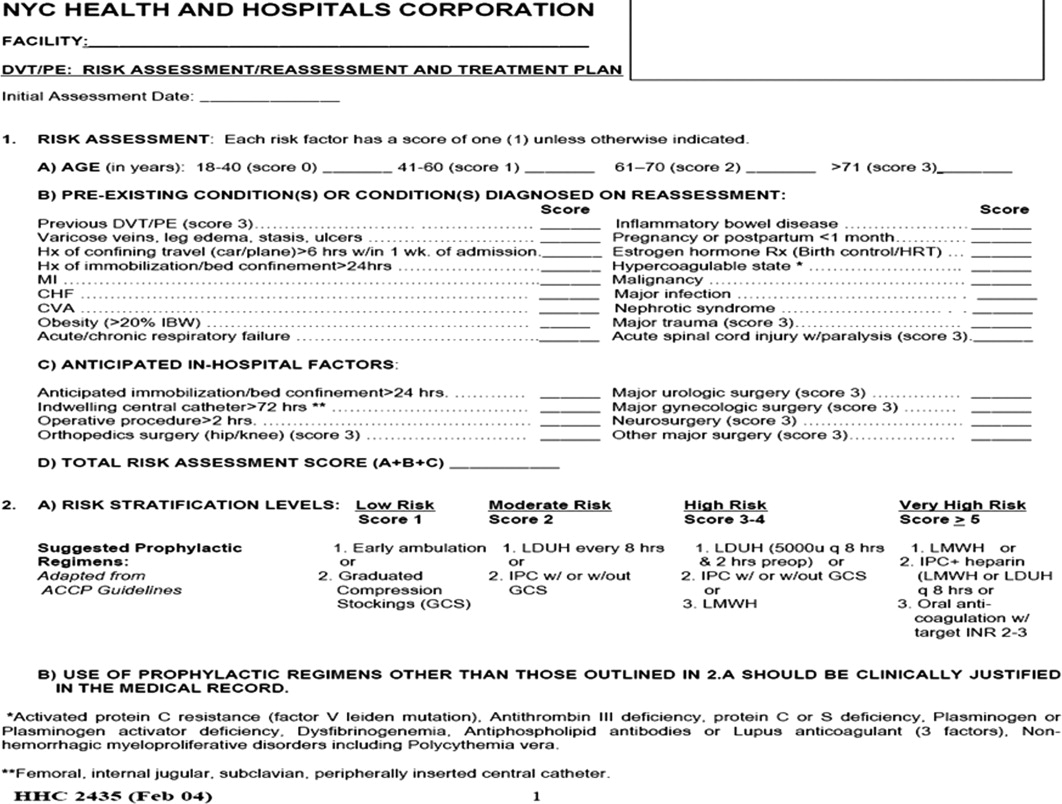
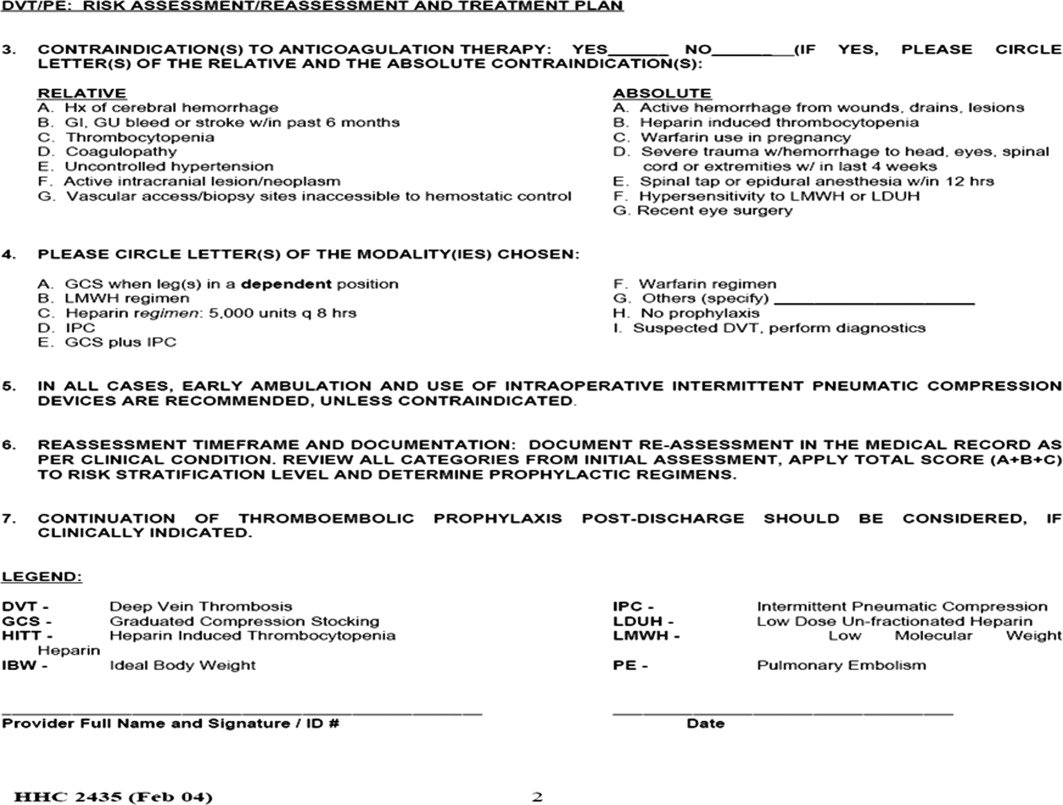
Auditing
Starting in December 2003, each house‐staff medicine service team was assessed for its rate of DVT prophylaxis on a monthly basis. Two chief residents would review 7 randomly selected charts from each of the 10 general medicine house‐staff teams. The data collected included number of patients at increased risk of developing DVT who were receiving DVT prophylaxis defined as UFH, LMWH, and/or intermittent pneumatic compression. Patients were considered at increased risk of developing DVT if they had heart failure, myocardial infarction, cancer, systemic infection, a hypercoagulable state, respiratory failure, chronic obstructive pulmonary disease, pulmonary hypertension, hip or other lower extremity fracture, stroke, catatonia, or a history of previous DVT or PE. In addition, patients for whom bed rest was ordered or who were expected or observed to be immobile (ie, not get out of bed) were considered at increased risk of DVT. The DVT prophylaxis rate was the percentage of patients identified as at increased risk of developing DVT who were receiving DVT prophylaxis. The reviewers used standardized review sheets to collect the data. Each month they reviewed a total of 70 charts, which represented approximately 14% of all medicine house‐staff discharges and approximately 60% of the average daily census of the house‐staff service.
Hospital‐acquired DVT cases were actively identified and reviewed independently by the Department of Risk Management. This activity resulted from the requirement to report all hospital‐acquired DVTs to the New York State Department of Health Patient Outcome Reporting Tracking System. The risk management staff systematically reviewed all discharge International Classification of Diseases Ninth Edition (ICD‐9) codes, as well as the reports of all duplex scans of the upper and lower extremities. A review of the medical record was triggered by report of a DVT on an imaging study or the finding of either ICD‐9 code 453.8 (embolism and thrombosis of the vein) or ICD‐9 code 453.9 (embolism and thrombosis of unspecified site). The purpose of the chart review was to confirm the diagnosis of DVT and to determine if the DVT was indeed hospital acquired. Hospital‐acquired DVTs were defined as those that developed while admitted or within 30 days of discharge from Kings County Hospital Center. Developed during admission was defined as a diagnosis of DVT not being suspected at original presentation at the hospital. If DVT was suspected on admission and the patient had not been hospitalized at Kings County Hospital Center in the preceding 30 days, the case was excluded.
Feedback
Reporting of the DVT prophylaxis rate was initiated in December 2003 (Fig. 1, arrow D). During the last week of each month, the rate of DVT prophylaxis on the general medicine house‐staff service was reported at the morbidity and mortality (M&M) conference. Attendance at the M&M conference was expected of all members of the house staff and faculty attendings assigned to the general medicine service for that particular month. The conference was chaired by the chief medical residents and was attended by the chief of the department of medicine and the director of inpatient services, who actively supported the project and acknowledged the importance of DVT prophylaxis.
Starting in March 2004, team‐specific DVT prophylaxis rates were reported at the monthly M&M conference rather than a single rate for the entire house‐staff service (Fig. 1, arrow E). This feedback enabled assessment of individual team performance and created a sense of competition. Each team was composed of an attending physician, a postgraduate year 3 resident, and a postgraduate year 1 resident. The feedback was presented as a focal point of the conference each month, one of the department's continuous quality improvement measures. The annual hospital‐acquired DVT rates observed from 2002 onward were also reported at this conference. Opportunity was given for discussion, comments, and feedback at the end of each conference, and occasionally new ideas for improvement were generated.
Statistical Analysis
A generalized linear model was used to estimate 95% confidence intervals for annual DVT incidence rates and to compare rates for 20032005 with that of 2002. Bonferroni corrections were applied to P values in order to control the overall type I error rate. Calendar year was the only independent variable in the analysis.
RESULTS
Table 1 provides cumulative yearly data for DVT prophylaxis rate, number of hospital‐acquired DVTs, and number of discharges from the general medicine house‐staff service for the 4 years of the observational period, 20022005. The baseline DVT prophylaxis rate from May 2002 to December 2002 was 63% (Fig. 4 and Table 1) and increased to 73%, 90%, and 96% over the succeeding years. In 2002, the number of hospital‐acquired DVTs on the general medicine house‐staff service was 14, followed by 16, 7, and 1 for 2003 through 2005 (Table 1). Twenty‐four of these 38 cases had not received DVT prophylaxis.
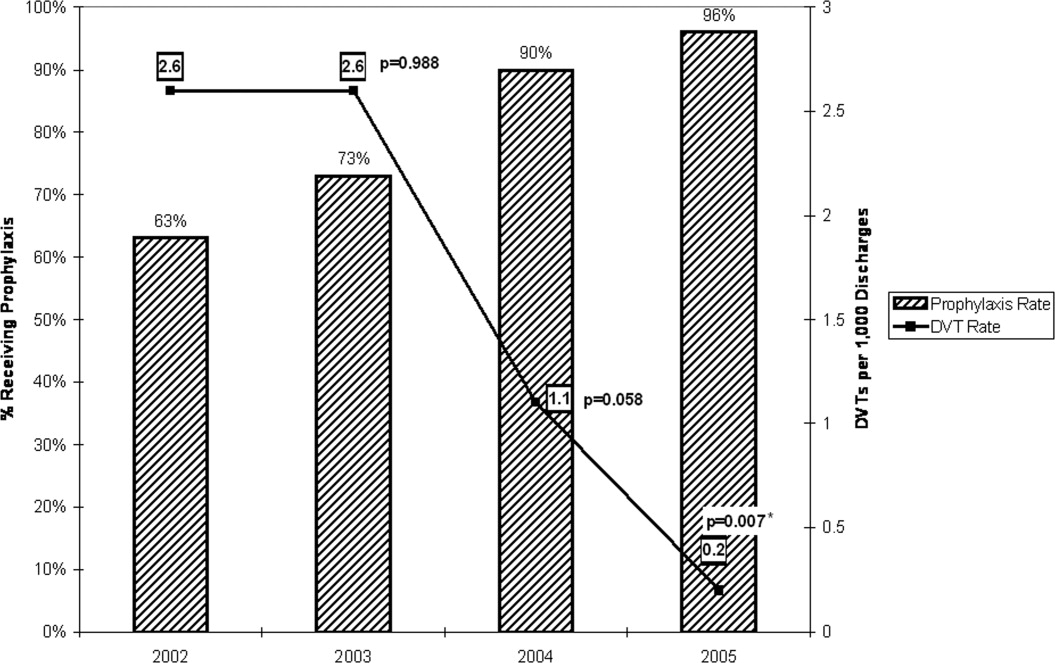
Year | DVT prophylaxis rate | Hospital‐acquired DVTs (n) | Discharges (n) | Hospital‐acquired DVT rate (DVTs per 1000 discharges) | 95% Confidence interval | P value |
---|---|---|---|---|---|---|
| ||||||
2002 (Year 1) | 63% | 14 | 5366 | 2.6 | 1.54.4 | |
2003 (Year 2) | 73% | 16 | 6098 | 2.6 | 1.64.3 | .988 |
2004 (Year 3) | 90% | 7 | 6460 | 1.1 | 0.52.3 | .058 |
2005 (Year 4) | 96% | 1 | 6296 | 0.2 | 0.01.1 | .007* |
When adjusted for each 1000 discharges (Fig. 4 and Table 1), the rates of hospital‐acquired DVT significantly decreased, from 2.6 per 1000 discharges (95% CI 1.54.4) in 2002 to 1.1 per 1000 discharges (95% CI 0.52.3, P = .058) in 2004 and to 0.2 per 1000 discharges (95% CI 0.01.1, P = .007) in 2005. During these years, particularly 20042005, the monthly DVT prophylaxis rate in the sample reviewed was consistently 90% or better (Fig. 1).
DISCUSSION
Our study involved active multifaceted interventions with a layered combination of provider education, provider reminders with decision support, and audit and feedback. This layered approach increased the DVT prophylaxis rate in our department, resulting in a significant decline in clinically evident hospital‐acquired DVTs from a baseline rate of 2.6 per 1000 discharges (0.26%) in 2002 to a rate of 0.2 per 1000 discharges (0.02%) in 2005 (95% CI 0.01.1, P = .007). Our baseline rate was low compared to the nationally reported incidence of 1.3%.4 Had the study not been extended over 4 years, it is likely that the statistical significance of this decline would have been missed. The rate of decrease in hospital0acquired DVTs accelerated with each year, showing no decline between 2002 and 2003, a 58% decline from 2003 to 2004, and an 82% decline from 2004 to 2005.
The acceleration in this decline coincided with the use of pocket DVT prophylaxis guideline cards and monthly audits with feedback starting in 2003 and the implementation of preprinted admission orders in 2004. This acceleration peaked in 2005 with the addition of the DVT algorithm to the admission database.
Despite the ACCP guidelines on thromboprophylaxis,8 several studies have suggested that only approximately 15%16% of medically ill patients at increased risk of VTE receive adequate thromboprophylaxis.9, 10 The 3 barriers identified by Kakkar et al. were lack of physician awareness, disagreement with the guidelines, and lack of outcome data.11 Our interventions addressed these barriers. Lack of physician awareness was addressed by provider education and reminder systems with decision support. Audit with team‐specific feedback provided the outcome data needed to demonstrate and reinforce effective change.
A critical analysis by Shojania et al. analyzed the effectiveness of each of these strategies when implemented alone. Provider education was effective in increasing provider knowledge but was generally ineffective when judged on the basis of improving patient outcomes. When provider reminders were well integrated with work flow, they were more likely to be effective. The effectiveness of decision support was variable, as it sometimes brought about change but was less likely to do so in complex situations. Various forms of audit and feedback produced small to modest effective changes.15 A systematic review by Oxman et al. suggested that there were some benefits when these strategies were implemented in a layered manner compared with single‐faceted strategies and that effective interventions were more likely to involve active rather than passive strategies.16
The major strengths of this study were the large number of patients reviewed, the sustained interventions over a 4‐year period, and the consistency of our results. The Department of Risk Management systematically reviewed all discharge ICD‐9 codes and all relevant imaging studies of 32,293 patients for the presence of hospital‐acquired DVTs. The data provided was all‐inclusive regardless of outcome and was acquired continuously over a 4‐year period rather than at a single point.
Our department continues to seek methods to increase the rate of thromboprophylaxis, and so, noting that computer alert programs have been shown to increase the appropriate use of thromboprophylaxis,14 our institution has designed an admission computer order entry program specific for DVT prophylaxis. This program prompts physicians to perform a DVT risk assessment of each admitted patient and to order the appropriate thromboprophylaxis regimen if indicated. This program was implemented in May 2006.
Limitations
Several weaknesses are inherent in a before‐and‐after study such as ours. These include the presence of background factors that can produce significant changes in processes or outcomes of interest regardless of quality improvement interventions. Another weakness is that for any given period studied, multiple unaccounted changes typically occur in a health care system and in its socioeconomic environment that might also produce the desired improvements.17 An attempt was made to mitigate the presence of background factors by including a large number of patients in our analysis, by conducting the study over 4 years rather than over a shorter period, and by analyzing data continuously throughout each year rather than at a single point. These weaknesses could be further ameliorated by a controlled before‐and‐after study design in which there would be analysis of data from 2 hospitals, one that implemented the quality improvement interventions and one that did not.
Several authority gradients were used in this initiative, including the supervising faculty attending assigned to each medical team, who was required to cosign each of the DVT risk assessment sheets in the admitting database, providing a powerful tool in the promotion of provider reminder and decision support in this initiative. The other authority gradients included the chief of medicine and the director of inpatient services, who both presided over the monthly morbidity and mortality conferences where performance feedback of the initiative was presented. The authority gradients at these performance feedback sessions were useful in promoting discussion and gaining valuable feedback, as well as in garnering ideas for improvement from the house staff. However, although these authority gradients were important in carrying out this initiative, having them may not be possible at other institutions, particularly nonacademic hospitals, and as such could potentially limit reproducibility.
Another limitation of our study is that we included only patients with DVT. Patients with PE without an identified DVT were not included. In addition, there was no specific, systematic review of complications such as heparin‐induced thrombocytopenia (HIT) and bleeding. However, our departmental and hospitalwide quality improvement programs do attempt to identify complications of treatment and adverse drug reactions. There was no observed increase in bleeding complications and only 2 cases of HIT were identified in our department during the 4‐year period studied. Reported rates of HIT and bleeding in medical patients receiving LMWH or UFH for DVT prophylaxis have been low.57, 18
The DVT cases identified from 2002 to 2005 were those that were clinically relevant. Asymptomatic, clinically silent cases were not included in this report because routine screening for subclinical DVT is not presently a part of routine general medicine inpatient care.
CONCLUSIONS
The multifaceted layered combination of provider education, provider reminders with decision support, and audit and feedback implemented at our institution could be reproduced and utilized at other institutions, particularly teaching hospitals, to address the underutilization of DVT prophylaxis in medically ill patients and to bring about a decrease in hospital‐acquired DVTs.
- Fatal pulmonary embolism in hospitalised patients: a necropsy review.J Clin Pathol.2004;57:1254–1257. , , , .
- New onset of venous thromboembolism among hospitalized patients at Brigham and Women's Hospital is caused more often by prophylaxis failure than by withholding treatment.Chest.2000;118:1680–1684. , , .
- Preventing venous thromboembolism in medical patients.Circulation.2004;110(24 Suppl 1):IV13–IV19. , .
- Trends in the incidence of pulmonary embolism and deep venous thrombosis in hospitalized patients.Am J Cardiol.2005;95:1525–1526. , , .
- A comparison of enoxaparin with placebo for the prevention of venous thromboembolism in acutely ill medical patients.N Engl J Med.1999;341:793–800. , , , et al.
- Prevention of venous thromboembolism in internal medicine with unfractionated or low‐molecular‐weight heparins: a meta‐analysis of randomised clinical trials.Thromb Haemost.2000;83(1):14–19. , , , et al.
- Randomized, placebo‐controlled trial of dalteparin for the prevention of venous thromboembolism in acutely ill medical patients.Circulation.2004;110:874–879. , , , et al.
- Prevention of venous thromboembolism: the Seventh ACCP Conference on Antithrombotic and Thrombolytic Therapy.Chest.2004;126(3 Suppl):338S–400S. , , , et al.
- Hospitals' compliance with prophylaxis guidelines for venous thromboembolism.Am J Health Syst Pharm.2007;64(1):69–76. , , , .
- Multicenter evaluation of the use of venous thromboembolism prophylaxis in acutely ill medical patients in Canada.Thromb Res.2007;119(2):145–155. , , , et al.
- Compliance with recommended prophylaxis for venous thromboembolism: improving the use and rate of uptake of clinical practice guidelines.J Thromb Haemost.2004;2:221–227. , , .
- Effect of a clinical pharmacy education program on improvement in the quantity and quality of venous thromboembolism prophylaxis for medically ill patients.J Manag Care Pharm.2005;11:755–762. , .
- Improved use of thromboprophylaxis for deep vein thrombosis following an educational intervention.J Hosp Med.2006;1:331–338. , , .
- Electronic alerts to prevent venous thromboembolism among hospitalized patients.N Engl J Med.2005;352:969–977. , , , et al.
- Closing the Quality Gap: A Critical Analysis of Quality Improvement Strategies.Rockville, MD:Agency for Healthcare Research and Quality;2004. , , , .
- No magic bullets: a systematic review of 102 trials of interventions to improve professional practice.CMAJ.1995;153:1423–1431. , , , .
- Evidence‐based quality improvement: the state of the science.Health Aff (Millwood).2005;24(1):138–150. , .
- Incidence and economic implications of heparin‐induced thrombocytopenia in medical patients receiving prophylaxis for venous thromboembolism.Pharmacotherapy.2006;26:1438–1445. , , , .
- Fatal pulmonary embolism in hospitalised patients: a necropsy review.J Clin Pathol.2004;57:1254–1257. , , , .
- New onset of venous thromboembolism among hospitalized patients at Brigham and Women's Hospital is caused more often by prophylaxis failure than by withholding treatment.Chest.2000;118:1680–1684. , , .
- Preventing venous thromboembolism in medical patients.Circulation.2004;110(24 Suppl 1):IV13–IV19. , .
- Trends in the incidence of pulmonary embolism and deep venous thrombosis in hospitalized patients.Am J Cardiol.2005;95:1525–1526. , , .
- A comparison of enoxaparin with placebo for the prevention of venous thromboembolism in acutely ill medical patients.N Engl J Med.1999;341:793–800. , , , et al.
- Prevention of venous thromboembolism in internal medicine with unfractionated or low‐molecular‐weight heparins: a meta‐analysis of randomised clinical trials.Thromb Haemost.2000;83(1):14–19. , , , et al.
- Randomized, placebo‐controlled trial of dalteparin for the prevention of venous thromboembolism in acutely ill medical patients.Circulation.2004;110:874–879. , , , et al.
- Prevention of venous thromboembolism: the Seventh ACCP Conference on Antithrombotic and Thrombolytic Therapy.Chest.2004;126(3 Suppl):338S–400S. , , , et al.
- Hospitals' compliance with prophylaxis guidelines for venous thromboembolism.Am J Health Syst Pharm.2007;64(1):69–76. , , , .
- Multicenter evaluation of the use of venous thromboembolism prophylaxis in acutely ill medical patients in Canada.Thromb Res.2007;119(2):145–155. , , , et al.
- Compliance with recommended prophylaxis for venous thromboembolism: improving the use and rate of uptake of clinical practice guidelines.J Thromb Haemost.2004;2:221–227. , , .
- Effect of a clinical pharmacy education program on improvement in the quantity and quality of venous thromboembolism prophylaxis for medically ill patients.J Manag Care Pharm.2005;11:755–762. , .
- Improved use of thromboprophylaxis for deep vein thrombosis following an educational intervention.J Hosp Med.2006;1:331–338. , , .
- Electronic alerts to prevent venous thromboembolism among hospitalized patients.N Engl J Med.2005;352:969–977. , , , et al.
- Closing the Quality Gap: A Critical Analysis of Quality Improvement Strategies.Rockville, MD:Agency for Healthcare Research and Quality;2004. , , , .
- No magic bullets: a systematic review of 102 trials of interventions to improve professional practice.CMAJ.1995;153:1423–1431. , , , .
- Evidence‐based quality improvement: the state of the science.Health Aff (Millwood).2005;24(1):138–150. , .
- Incidence and economic implications of heparin‐induced thrombocytopenia in medical patients receiving prophylaxis for venous thromboembolism.Pharmacotherapy.2006;26:1438–1445. , , , .
Copyright © 2008 Society of Hospital Medicine
Delays in Diagnosis and Treatment
Clostridium difficileassociated disease (CDAD) is a well‐known complication of hospitalization and is the most frequently identified cause of nosocomial diarrhea that hospitalists encounter. Despite widespread epidemiologic attempts to control the disease, its prevalence and clinical severity appear to be increasing.1 The resulting social and economic consequences are profound. The estimated 3 million inpatient cases of CDAD a year result in an average increase in the length of stay of 3.6 days at a cost in inpatient health care of more than $1 billion.2
Early diagnosis of index cases is crucial. A diagnostic delay can result in a treatment delay for the index case, as well as in a delay in implementing isolation procedures to prevent horizontal transmission. Acquisition of CDAD is time dependent and occurs in 20% to 30% of hospitalized patients at a rate of approximately 8% per week.3, 4 This transmission is primarily a result of environmental contamination with CDAD spores, found on 59% of the hands of hospital personnel caring for infected patients, in 49% of rooms of symptomatic patients, and in 29% of rooms of asymptomatic carriers.5 Despite the need for early diagnosis, a study from the United Kingdom documented that the average time from the onset of diarrhea to sampling of CDAD patients is 4.7 days.6 An additional challenge for early diagnosis is the delay in microbiological confirmation of CDAD in a suspected patient. Cytotoxic assays, which have become the standard diagnostic technique for CDAD, exhibit excellent sensitivity and specificity but have a lengthy processing time, between 2 and 4 days. Although antigen detection assays can be rapidly performed, many have inadequate sensitivity and specificity.7
These issues of diagnostic and treatment delays are compounded in patients with recurrent CDAD. As many as 15%35% of patients with an initial CDAD infection will experience a recurrence, usually within 2 months. At least half these infections are a result of reinfection, not relapse.8 This implies that early detection and strict isolation of infected patients is essential for reducing the exposure of at‐risk patients to the disease. There is evidence that the burden of patients on the same ward simultaneously having CDAD increases a patient's risk of acquiring the disease.9 It is currently unknown if recurrent CDAD cases are diagnosed or treated earlier than initial cases. If not, this is a potentially important patient population for hospitalists to target for aggressive containment strategies. This study sought to determine the mean time to sampling and treatment in patients with recurrent CDAD infection compared with those in patients who are initially infected.
Design
The study cohort consisted of all adult patients more than 18 years old with CDAD (by ICD9 code) who had been hospitalized at Brigham and Women's Hospital between 1997 and 2004. Retrospectively, patients were identified through the Partners Healthcare Research Patient Data Repository (RPDR). The RPDR is a centralized clinical data registry that gathers data from various hospital legacy systems and was used to determine the patient demographics and first date of treatment (with vancomycin or metronidazole). Medical and microbiologic records were reviewed to determine the dates of cytotoxic assay submission and symptom onset. Symptoms were defined as diarrhea, abdominal pain/cramping, or radiological/colonoscopic evidence of colitis. Recurrence was defined as any repeat inpatient CDAD diagnosis within 2 months (regardless of admission diagnosis). Baseline characteristics in the recurrence and no‐recurrence populations were compared by the 2‐sided Student t test or the chi‐square test (for continuous and categorical variables, respectively). Mean time from symptom to sampling, from symptom to treatment, and from sampling to treatment were compared between initial and recurrent disease episodes by the 2‐sided Student t test. All P values < .05 were considered significant. Institutional review board approval was obtained by Partners Healthcare.
RESULTS
Between 1997 and 2004 there were 1309 patients with an ICD9 code for CDAD, 151 of whom (12%) had a recurrence. Of these, 125 had 1 recurrence, 23 had 2 recurrences, and 3 had 3 recurrences. There were no significant differences between the groups in basic demographics (Table 1). The mean time to sampling was not significantly different between initial and recurrent CDAD hospital episodes (Table 2). However, the mean time to treatment (from symptoms and sampling) was shorter in recurrent episodes (Table 2). From 1997 to 2004 there was no significant reduction in time to sampling, but there was a significant reduction in time to treatment, from 3.89 days (19972000) to 2.30 days (2001 2004), P = .0012.
Characteristic | Patients without recurrent disease (n = 1158) | Patients with recurrent disease (n = 151) | P value |
---|---|---|---|
Sex (% male) | 45% | 45% | .98 |
Age (mean) | 68.3 years | 69.9 years | .72 |
Race (% white) | 80% | 80% | .97 |
Language (English) | 94% | 92% | .83 |
First episode (n = 1309) | Recurrence (n = 180)* | P value | |
---|---|---|---|
| |||
Symptoms to sampling | 2.24 days (117 days) | 2.09 days (116 days) | 0.700 |
Symptoms to treatment | 3.64 days (118 days) | 2.52 days (119 days) | 0.024 |
Sampling to treatment | 3.76 days (119 days) | 2.57 days (119 days) | 0.006 |
DISCUSSION
Clostridium difficileassociated disease (CDAD) has become a significant nosocomial infection in medical institutions, and recurrent CDAD is emerging as a disease of concern for hospitalists. Diagnostic delays represent a major epidemiologic problem, resulting in both delay of treatment delay of the index case and delay in implementing isolation procedures to prevent horizontal transmission. In this study, patients with recurrent disease did not have stool collected any earlier than did patients with their initial episode of CDAD, and these diagnostic delays did not change in successive eras. Recurrent disease patients did receive treatment earlier than did patients with initial episodes. Although this empiric treatment strategy is encouraging and likely reflects heightened awareness of the disease over time, the 2.5‐day span from symptoms to treatment is still a clinically significant delay. Also of concern is the range of time from symptoms to treatment, as long as 19 days in the recurrent treatment group. Although most patients were treated within 12 days, this variability represents the burden of infectious patients with the potential for infecting others. Targeting recurrent CDAD populations for early diagnosis, treatment, and isolation would almost certainly reduce the morbidity associated with horizontal transmission rates.9
This study had several limitations. Our data found a lower incidence of recurrent CDAD than previously published in the literature. This can be accounted for by the identification of cases by ICD9 code, which previously has been documented to underestimate true disease.10, 11 We also were not able to capture recurrent episodes in outpatients or episodes that occurred after the 2004 cohort, which underestimated the true frequency of recurrence. At worst, this underestimation could bias the results toward the null hypothesis. An additional limitation of the study was the assumption that time to treatment was accurately reflected by time to prescription of either vancomycin or flagyl. Some patients may have been treated by suspending treatment with the offending antibiotic along with watchful waiting, which is a reasonable strategy for patients with mild disease and is endorsed by the American College of Gastroenterology and the Society for Healthcare Epidemiology of America.12, 13 This would overestimate time to treatment for those individuals and would make time to treatment appear longer, but would not affect time to sampling. In addition, the symptoms collected from chart review were assumed to be a result of the patient's CDAD, but there is a chance that these symptoms such as diarrhea, abdominal pain, and cramping may have been a result of a different diagnosis. These data were also limited to a cohort from a single institution and may not reflect the patient characteristics or practice patterns at other institutions.
In conclusion, CDAD is a major contributor to morbidity from nosocomial infections, and recurrent CDAD patients are a likely source of horizontal disease transmission. This study documented that there are significant diagnostic and treatment delays, even in populations with recurrent disease. It is especially important that hospitalists take measures to improve the early diagnosis, treatment, and isolation of these patients in order to improve these deficiencies in care.
- Ten years of prospective Clostridium difficile‐associated disease surveillance and treatment at the Minneapolis VA Medical Center, 1982–1991.Infect Control Hosp Epidemiol.1994;15:371–381. , , , .
- Health care costs and mortality associated with nosocomial diarrhea due to clostridium difficile.Clin Infect Dis.2002;34:346–353. , , , .
- Nosocomial Clostridium difficile colonization and disease.Lancet.1990;336:97–100. , , , et al.
- Asymptomatic carriage of Clostridium difficile and serum levels of IgG antibody against Toxin A.N Engl J Med.2000;342:390–397. , , , .
- Nosocomial acquisition of Clostridium difficile infection.N Engl J Med.1989;320:204–210. , , , .
- Reducing delays in the diagnosis and treatment of Clostridium difficile diarrhoea.QJM.2003;96:579–582. , .
- Multicenter evaluation of four methods for Clostridium difficile detection: ImmunoCard C. difficile, cytotoxin assay, culture, and latex agglutination.J Clin Microbiol.1996;34:2718–2721. , , , et al.
- Epidemiology of recurrences or reinfections of Clostridium difficile–associated diarrhea.J Clin Microbiol.2000;38:2386–2388. , , , et al.
- Evaluation of Clostridium difficile‐associated disease pressure as a risk factor for C difficile–associated disease.Arch Intern Med.2007;167:1092–1097. , , , et al.
- Accuracy of ICD9 coding for Clostridium difficile infections.Epidemiol Infect.2006;135:1010–1013. , , , .
- Emerging Infectious Diseases online publication Available at: http://www.cdc.gov/ncidod/EID/vol12no10/06‐0016.htm. Accessed July 12,2007.
- Guidelines for the diagnosis and management of Clostridium difficile–associated diarrhea and colitis.American College of Gastroenterology, Practice Parameters Committee.Am J Gastroenterol.1997;92:739–750. .
- SHEA position paper. Clostridium difficile associated diarrhea and colitis.Infect Control Hosp Epidemiol.1995;16:459–477. , , , , .
Clostridium difficileassociated disease (CDAD) is a well‐known complication of hospitalization and is the most frequently identified cause of nosocomial diarrhea that hospitalists encounter. Despite widespread epidemiologic attempts to control the disease, its prevalence and clinical severity appear to be increasing.1 The resulting social and economic consequences are profound. The estimated 3 million inpatient cases of CDAD a year result in an average increase in the length of stay of 3.6 days at a cost in inpatient health care of more than $1 billion.2
Early diagnosis of index cases is crucial. A diagnostic delay can result in a treatment delay for the index case, as well as in a delay in implementing isolation procedures to prevent horizontal transmission. Acquisition of CDAD is time dependent and occurs in 20% to 30% of hospitalized patients at a rate of approximately 8% per week.3, 4 This transmission is primarily a result of environmental contamination with CDAD spores, found on 59% of the hands of hospital personnel caring for infected patients, in 49% of rooms of symptomatic patients, and in 29% of rooms of asymptomatic carriers.5 Despite the need for early diagnosis, a study from the United Kingdom documented that the average time from the onset of diarrhea to sampling of CDAD patients is 4.7 days.6 An additional challenge for early diagnosis is the delay in microbiological confirmation of CDAD in a suspected patient. Cytotoxic assays, which have become the standard diagnostic technique for CDAD, exhibit excellent sensitivity and specificity but have a lengthy processing time, between 2 and 4 days. Although antigen detection assays can be rapidly performed, many have inadequate sensitivity and specificity.7
These issues of diagnostic and treatment delays are compounded in patients with recurrent CDAD. As many as 15%35% of patients with an initial CDAD infection will experience a recurrence, usually within 2 months. At least half these infections are a result of reinfection, not relapse.8 This implies that early detection and strict isolation of infected patients is essential for reducing the exposure of at‐risk patients to the disease. There is evidence that the burden of patients on the same ward simultaneously having CDAD increases a patient's risk of acquiring the disease.9 It is currently unknown if recurrent CDAD cases are diagnosed or treated earlier than initial cases. If not, this is a potentially important patient population for hospitalists to target for aggressive containment strategies. This study sought to determine the mean time to sampling and treatment in patients with recurrent CDAD infection compared with those in patients who are initially infected.
Design
The study cohort consisted of all adult patients more than 18 years old with CDAD (by ICD9 code) who had been hospitalized at Brigham and Women's Hospital between 1997 and 2004. Retrospectively, patients were identified through the Partners Healthcare Research Patient Data Repository (RPDR). The RPDR is a centralized clinical data registry that gathers data from various hospital legacy systems and was used to determine the patient demographics and first date of treatment (with vancomycin or metronidazole). Medical and microbiologic records were reviewed to determine the dates of cytotoxic assay submission and symptom onset. Symptoms were defined as diarrhea, abdominal pain/cramping, or radiological/colonoscopic evidence of colitis. Recurrence was defined as any repeat inpatient CDAD diagnosis within 2 months (regardless of admission diagnosis). Baseline characteristics in the recurrence and no‐recurrence populations were compared by the 2‐sided Student t test or the chi‐square test (for continuous and categorical variables, respectively). Mean time from symptom to sampling, from symptom to treatment, and from sampling to treatment were compared between initial and recurrent disease episodes by the 2‐sided Student t test. All P values < .05 were considered significant. Institutional review board approval was obtained by Partners Healthcare.
RESULTS
Between 1997 and 2004 there were 1309 patients with an ICD9 code for CDAD, 151 of whom (12%) had a recurrence. Of these, 125 had 1 recurrence, 23 had 2 recurrences, and 3 had 3 recurrences. There were no significant differences between the groups in basic demographics (Table 1). The mean time to sampling was not significantly different between initial and recurrent CDAD hospital episodes (Table 2). However, the mean time to treatment (from symptoms and sampling) was shorter in recurrent episodes (Table 2). From 1997 to 2004 there was no significant reduction in time to sampling, but there was a significant reduction in time to treatment, from 3.89 days (19972000) to 2.30 days (2001 2004), P = .0012.
Characteristic | Patients without recurrent disease (n = 1158) | Patients with recurrent disease (n = 151) | P value |
---|---|---|---|
Sex (% male) | 45% | 45% | .98 |
Age (mean) | 68.3 years | 69.9 years | .72 |
Race (% white) | 80% | 80% | .97 |
Language (English) | 94% | 92% | .83 |
First episode (n = 1309) | Recurrence (n = 180)* | P value | |
---|---|---|---|
| |||
Symptoms to sampling | 2.24 days (117 days) | 2.09 days (116 days) | 0.700 |
Symptoms to treatment | 3.64 days (118 days) | 2.52 days (119 days) | 0.024 |
Sampling to treatment | 3.76 days (119 days) | 2.57 days (119 days) | 0.006 |
DISCUSSION
Clostridium difficileassociated disease (CDAD) has become a significant nosocomial infection in medical institutions, and recurrent CDAD is emerging as a disease of concern for hospitalists. Diagnostic delays represent a major epidemiologic problem, resulting in both delay of treatment delay of the index case and delay in implementing isolation procedures to prevent horizontal transmission. In this study, patients with recurrent disease did not have stool collected any earlier than did patients with their initial episode of CDAD, and these diagnostic delays did not change in successive eras. Recurrent disease patients did receive treatment earlier than did patients with initial episodes. Although this empiric treatment strategy is encouraging and likely reflects heightened awareness of the disease over time, the 2.5‐day span from symptoms to treatment is still a clinically significant delay. Also of concern is the range of time from symptoms to treatment, as long as 19 days in the recurrent treatment group. Although most patients were treated within 12 days, this variability represents the burden of infectious patients with the potential for infecting others. Targeting recurrent CDAD populations for early diagnosis, treatment, and isolation would almost certainly reduce the morbidity associated with horizontal transmission rates.9
This study had several limitations. Our data found a lower incidence of recurrent CDAD than previously published in the literature. This can be accounted for by the identification of cases by ICD9 code, which previously has been documented to underestimate true disease.10, 11 We also were not able to capture recurrent episodes in outpatients or episodes that occurred after the 2004 cohort, which underestimated the true frequency of recurrence. At worst, this underestimation could bias the results toward the null hypothesis. An additional limitation of the study was the assumption that time to treatment was accurately reflected by time to prescription of either vancomycin or flagyl. Some patients may have been treated by suspending treatment with the offending antibiotic along with watchful waiting, which is a reasonable strategy for patients with mild disease and is endorsed by the American College of Gastroenterology and the Society for Healthcare Epidemiology of America.12, 13 This would overestimate time to treatment for those individuals and would make time to treatment appear longer, but would not affect time to sampling. In addition, the symptoms collected from chart review were assumed to be a result of the patient's CDAD, but there is a chance that these symptoms such as diarrhea, abdominal pain, and cramping may have been a result of a different diagnosis. These data were also limited to a cohort from a single institution and may not reflect the patient characteristics or practice patterns at other institutions.
In conclusion, CDAD is a major contributor to morbidity from nosocomial infections, and recurrent CDAD patients are a likely source of horizontal disease transmission. This study documented that there are significant diagnostic and treatment delays, even in populations with recurrent disease. It is especially important that hospitalists take measures to improve the early diagnosis, treatment, and isolation of these patients in order to improve these deficiencies in care.
Clostridium difficileassociated disease (CDAD) is a well‐known complication of hospitalization and is the most frequently identified cause of nosocomial diarrhea that hospitalists encounter. Despite widespread epidemiologic attempts to control the disease, its prevalence and clinical severity appear to be increasing.1 The resulting social and economic consequences are profound. The estimated 3 million inpatient cases of CDAD a year result in an average increase in the length of stay of 3.6 days at a cost in inpatient health care of more than $1 billion.2
Early diagnosis of index cases is crucial. A diagnostic delay can result in a treatment delay for the index case, as well as in a delay in implementing isolation procedures to prevent horizontal transmission. Acquisition of CDAD is time dependent and occurs in 20% to 30% of hospitalized patients at a rate of approximately 8% per week.3, 4 This transmission is primarily a result of environmental contamination with CDAD spores, found on 59% of the hands of hospital personnel caring for infected patients, in 49% of rooms of symptomatic patients, and in 29% of rooms of asymptomatic carriers.5 Despite the need for early diagnosis, a study from the United Kingdom documented that the average time from the onset of diarrhea to sampling of CDAD patients is 4.7 days.6 An additional challenge for early diagnosis is the delay in microbiological confirmation of CDAD in a suspected patient. Cytotoxic assays, which have become the standard diagnostic technique for CDAD, exhibit excellent sensitivity and specificity but have a lengthy processing time, between 2 and 4 days. Although antigen detection assays can be rapidly performed, many have inadequate sensitivity and specificity.7
These issues of diagnostic and treatment delays are compounded in patients with recurrent CDAD. As many as 15%35% of patients with an initial CDAD infection will experience a recurrence, usually within 2 months. At least half these infections are a result of reinfection, not relapse.8 This implies that early detection and strict isolation of infected patients is essential for reducing the exposure of at‐risk patients to the disease. There is evidence that the burden of patients on the same ward simultaneously having CDAD increases a patient's risk of acquiring the disease.9 It is currently unknown if recurrent CDAD cases are diagnosed or treated earlier than initial cases. If not, this is a potentially important patient population for hospitalists to target for aggressive containment strategies. This study sought to determine the mean time to sampling and treatment in patients with recurrent CDAD infection compared with those in patients who are initially infected.
Design
The study cohort consisted of all adult patients more than 18 years old with CDAD (by ICD9 code) who had been hospitalized at Brigham and Women's Hospital between 1997 and 2004. Retrospectively, patients were identified through the Partners Healthcare Research Patient Data Repository (RPDR). The RPDR is a centralized clinical data registry that gathers data from various hospital legacy systems and was used to determine the patient demographics and first date of treatment (with vancomycin or metronidazole). Medical and microbiologic records were reviewed to determine the dates of cytotoxic assay submission and symptom onset. Symptoms were defined as diarrhea, abdominal pain/cramping, or radiological/colonoscopic evidence of colitis. Recurrence was defined as any repeat inpatient CDAD diagnosis within 2 months (regardless of admission diagnosis). Baseline characteristics in the recurrence and no‐recurrence populations were compared by the 2‐sided Student t test or the chi‐square test (for continuous and categorical variables, respectively). Mean time from symptom to sampling, from symptom to treatment, and from sampling to treatment were compared between initial and recurrent disease episodes by the 2‐sided Student t test. All P values < .05 were considered significant. Institutional review board approval was obtained by Partners Healthcare.
RESULTS
Between 1997 and 2004 there were 1309 patients with an ICD9 code for CDAD, 151 of whom (12%) had a recurrence. Of these, 125 had 1 recurrence, 23 had 2 recurrences, and 3 had 3 recurrences. There were no significant differences between the groups in basic demographics (Table 1). The mean time to sampling was not significantly different between initial and recurrent CDAD hospital episodes (Table 2). However, the mean time to treatment (from symptoms and sampling) was shorter in recurrent episodes (Table 2). From 1997 to 2004 there was no significant reduction in time to sampling, but there was a significant reduction in time to treatment, from 3.89 days (19972000) to 2.30 days (2001 2004), P = .0012.
Characteristic | Patients without recurrent disease (n = 1158) | Patients with recurrent disease (n = 151) | P value |
---|---|---|---|
Sex (% male) | 45% | 45% | .98 |
Age (mean) | 68.3 years | 69.9 years | .72 |
Race (% white) | 80% | 80% | .97 |
Language (English) | 94% | 92% | .83 |
First episode (n = 1309) | Recurrence (n = 180)* | P value | |
---|---|---|---|
| |||
Symptoms to sampling | 2.24 days (117 days) | 2.09 days (116 days) | 0.700 |
Symptoms to treatment | 3.64 days (118 days) | 2.52 days (119 days) | 0.024 |
Sampling to treatment | 3.76 days (119 days) | 2.57 days (119 days) | 0.006 |
DISCUSSION
Clostridium difficileassociated disease (CDAD) has become a significant nosocomial infection in medical institutions, and recurrent CDAD is emerging as a disease of concern for hospitalists. Diagnostic delays represent a major epidemiologic problem, resulting in both delay of treatment delay of the index case and delay in implementing isolation procedures to prevent horizontal transmission. In this study, patients with recurrent disease did not have stool collected any earlier than did patients with their initial episode of CDAD, and these diagnostic delays did not change in successive eras. Recurrent disease patients did receive treatment earlier than did patients with initial episodes. Although this empiric treatment strategy is encouraging and likely reflects heightened awareness of the disease over time, the 2.5‐day span from symptoms to treatment is still a clinically significant delay. Also of concern is the range of time from symptoms to treatment, as long as 19 days in the recurrent treatment group. Although most patients were treated within 12 days, this variability represents the burden of infectious patients with the potential for infecting others. Targeting recurrent CDAD populations for early diagnosis, treatment, and isolation would almost certainly reduce the morbidity associated with horizontal transmission rates.9
This study had several limitations. Our data found a lower incidence of recurrent CDAD than previously published in the literature. This can be accounted for by the identification of cases by ICD9 code, which previously has been documented to underestimate true disease.10, 11 We also were not able to capture recurrent episodes in outpatients or episodes that occurred after the 2004 cohort, which underestimated the true frequency of recurrence. At worst, this underestimation could bias the results toward the null hypothesis. An additional limitation of the study was the assumption that time to treatment was accurately reflected by time to prescription of either vancomycin or flagyl. Some patients may have been treated by suspending treatment with the offending antibiotic along with watchful waiting, which is a reasonable strategy for patients with mild disease and is endorsed by the American College of Gastroenterology and the Society for Healthcare Epidemiology of America.12, 13 This would overestimate time to treatment for those individuals and would make time to treatment appear longer, but would not affect time to sampling. In addition, the symptoms collected from chart review were assumed to be a result of the patient's CDAD, but there is a chance that these symptoms such as diarrhea, abdominal pain, and cramping may have been a result of a different diagnosis. These data were also limited to a cohort from a single institution and may not reflect the patient characteristics or practice patterns at other institutions.
In conclusion, CDAD is a major contributor to morbidity from nosocomial infections, and recurrent CDAD patients are a likely source of horizontal disease transmission. This study documented that there are significant diagnostic and treatment delays, even in populations with recurrent disease. It is especially important that hospitalists take measures to improve the early diagnosis, treatment, and isolation of these patients in order to improve these deficiencies in care.
- Ten years of prospective Clostridium difficile‐associated disease surveillance and treatment at the Minneapolis VA Medical Center, 1982–1991.Infect Control Hosp Epidemiol.1994;15:371–381. , , , .
- Health care costs and mortality associated with nosocomial diarrhea due to clostridium difficile.Clin Infect Dis.2002;34:346–353. , , , .
- Nosocomial Clostridium difficile colonization and disease.Lancet.1990;336:97–100. , , , et al.
- Asymptomatic carriage of Clostridium difficile and serum levels of IgG antibody against Toxin A.N Engl J Med.2000;342:390–397. , , , .
- Nosocomial acquisition of Clostridium difficile infection.N Engl J Med.1989;320:204–210. , , , .
- Reducing delays in the diagnosis and treatment of Clostridium difficile diarrhoea.QJM.2003;96:579–582. , .
- Multicenter evaluation of four methods for Clostridium difficile detection: ImmunoCard C. difficile, cytotoxin assay, culture, and latex agglutination.J Clin Microbiol.1996;34:2718–2721. , , , et al.
- Epidemiology of recurrences or reinfections of Clostridium difficile–associated diarrhea.J Clin Microbiol.2000;38:2386–2388. , , , et al.
- Evaluation of Clostridium difficile‐associated disease pressure as a risk factor for C difficile–associated disease.Arch Intern Med.2007;167:1092–1097. , , , et al.
- Accuracy of ICD9 coding for Clostridium difficile infections.Epidemiol Infect.2006;135:1010–1013. , , , .
- Emerging Infectious Diseases online publication Available at: http://www.cdc.gov/ncidod/EID/vol12no10/06‐0016.htm. Accessed July 12,2007.
- Guidelines for the diagnosis and management of Clostridium difficile–associated diarrhea and colitis.American College of Gastroenterology, Practice Parameters Committee.Am J Gastroenterol.1997;92:739–750. .
- SHEA position paper. Clostridium difficile associated diarrhea and colitis.Infect Control Hosp Epidemiol.1995;16:459–477. , , , , .
- Ten years of prospective Clostridium difficile‐associated disease surveillance and treatment at the Minneapolis VA Medical Center, 1982–1991.Infect Control Hosp Epidemiol.1994;15:371–381. , , , .
- Health care costs and mortality associated with nosocomial diarrhea due to clostridium difficile.Clin Infect Dis.2002;34:346–353. , , , .
- Nosocomial Clostridium difficile colonization and disease.Lancet.1990;336:97–100. , , , et al.
- Asymptomatic carriage of Clostridium difficile and serum levels of IgG antibody against Toxin A.N Engl J Med.2000;342:390–397. , , , .
- Nosocomial acquisition of Clostridium difficile infection.N Engl J Med.1989;320:204–210. , , , .
- Reducing delays in the diagnosis and treatment of Clostridium difficile diarrhoea.QJM.2003;96:579–582. , .
- Multicenter evaluation of four methods for Clostridium difficile detection: ImmunoCard C. difficile, cytotoxin assay, culture, and latex agglutination.J Clin Microbiol.1996;34:2718–2721. , , , et al.
- Epidemiology of recurrences or reinfections of Clostridium difficile–associated diarrhea.J Clin Microbiol.2000;38:2386–2388. , , , et al.
- Evaluation of Clostridium difficile‐associated disease pressure as a risk factor for C difficile–associated disease.Arch Intern Med.2007;167:1092–1097. , , , et al.
- Accuracy of ICD9 coding for Clostridium difficile infections.Epidemiol Infect.2006;135:1010–1013. , , , .
- Emerging Infectious Diseases online publication Available at: http://www.cdc.gov/ncidod/EID/vol12no10/06‐0016.htm. Accessed July 12,2007.
- Guidelines for the diagnosis and management of Clostridium difficile–associated diarrhea and colitis.American College of Gastroenterology, Practice Parameters Committee.Am J Gastroenterol.1997;92:739–750. .
- SHEA position paper. Clostridium difficile associated diarrhea and colitis.Infect Control Hosp Epidemiol.1995;16:459–477. , , , , .
Asthmatic Inpatient Influenza Immunization
Influenza is estimated to infect up to 40%‐54% of school‐age children during an influenza season.1, 2 Influenza infections result in missed school days, visits to primary care providers, prescriptions for antibiotics, hospitalizations, and secondary infections.310 Although influenza‐related mortality of pediatric patients is rare, influenza‐related morbidity of children and those secondarily infected is common and partially preventable.11
For at‐risk individuals, immunization against influenza is the best method of preventing morbidity. The Centers for Disease Control's Advisory Committee on Immunization Practice (CDC/ACIP) considers children with asthma at high risk of morbidity from influenza and since 1964 has recommended delivery of inactivated influenza vaccination to this population of patients. Even with this recommendation, the current level of adherence to yearly influenza vaccination of children with asthma is approximately 29%, according to the CDC analysis of the National Health Interview Survey.12 Although pediatric providers may prefer to provide preventive care in an ambulatory setting, the CDC/ACIP emphasizes the need for vaccination delivery wherever possible, including in acute‐care hospitals.11 Particularly in an acute‐care setting, vaccinating patients who are having an exacerbation of their asthma and who may have a compromised immune response may raise concerns. However, the vaccine has been shown to be efficacious even with pediatric asthmatics on a short course of corticosteroids.13, 14 Utilization of the inpatient visit as an opportunity to implement evidence‐based preventive care to pediatric asthmatics could have a beneficial effect on patient outcomes. Group‐based settings (school or health fair) are more cost effective than individual‐initiated settings (primary care) for influenza vaccination of pediatric patients.15 However, we found no studies investigating the effectiveness of vaccinating high‐risk patients in an acute‐care setting.
This study used established modeling techniques (decision and cost‐effectiveness analyses) to determine the potential clinical and cost benefits of vaccinating children against influenza during an asthma exacerbation in an acute‐care setting. We used decision analysis to determine the effectiveness of the intervention in improving the up‐to‐date (UTD) status of a hypothetical population. Based on this improvement in vaccination delivery, we completed a cost‐effectiveness analysis to determine direct and indirect costs, improvement in clinical outcomes attributable to influenza (clinic visits, antibiotic use, hospitalization, and secondary infections), and cost savings attributable to the intervention.
METHODS
Decision Analysis
We generated a decision tree to represent an inpatient intervention to assess and deliver influenza vaccination to children with asthma in acute‐care hospitals (Fig. 1). The design of the decision tree intentionally began with a decision node in order to introduce different assessment rates. This approach was used in order to accurately represent an inpatient intervention (where 100% assessment is not always attained) and to assess how less‐than‐perfect rates of assessment of influenza vaccine status would affect the success of the intervention. Once the decision tree was created, we surveyed the literature to obtain data on the assumptions of the decision tree (See Table 1 for assumptions of decision analysis with corresponding numbering in Figure 1 Decision Tree) including percentage of children with asthma who receive the influenza vaccine (29%),12 percentages of patients and caregivers who would agree to be vaccinated in an acute‐care settings (71%),16 and rates of response to vaccine reminder recall systems in a primary care setting (30%)17 in order to account for populations that need a second dose of vaccine to become UTD. To estimate the percentage of children who would require a second vaccination at 4 weeks, we utilized the Healthcare Cost and Utilization Project Kids Inpatient Database (HCUP KID).18 Seventy‐three percent of children younger than 9 years old in the HCUP KID were discharged with a diagnosis of asthma. These patients would require a second vaccination after 4 weeks if they had not previously been immunized. After all the estimates were placed in the decision tree, a hypothetical cohort of children with asthma was introduced, and improvement in UTD status after the intervention was determined.
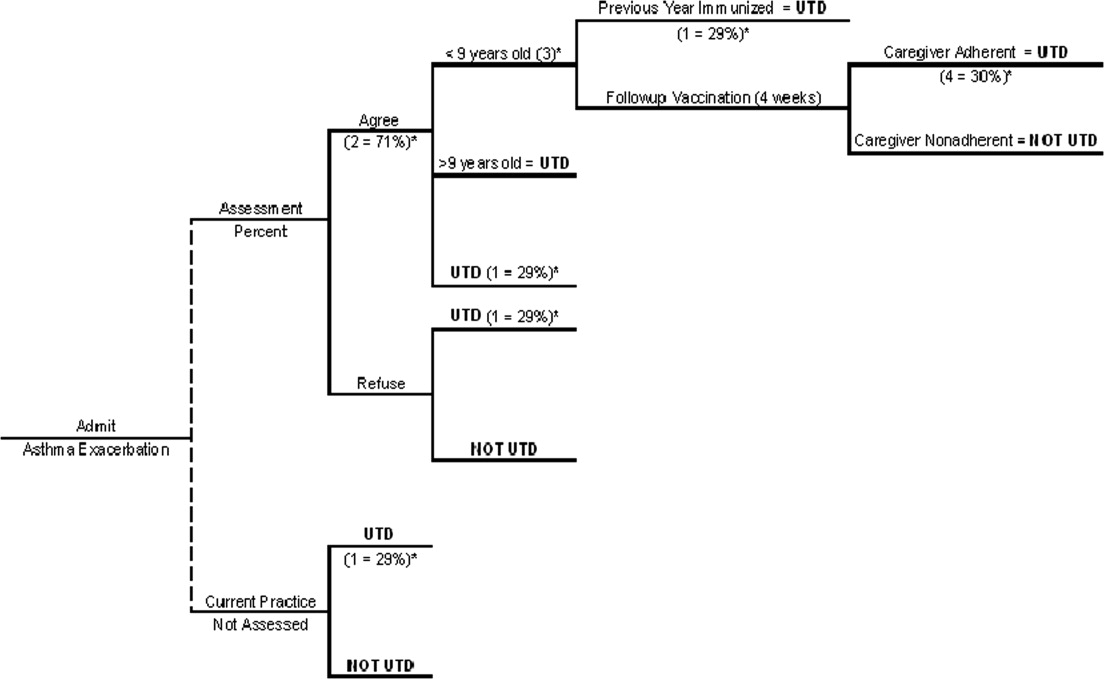
Reference | ||
---|---|---|
Decision analysis | ||
1. Percentage of asthmatics UTD with influenza vaccination without increased inpatient assessment | 29% | 12 |
2. Percentage of caregivers who agree with vaccination in an acute‐care setting | 71% | 16 |
3. Percentage of asthma exacerbation discharges <9 years old | 73% | 18 |
4. Percentage of caregivers who respond to reminder‐recall systems for outpatient vaccination | 30% | 17 |
Cost‐effectiveness analysis | ||
5. Prevalence of influenza in school‐age children over 1 season | 45% | 1 |
6. Vaccine efficacy | 15 | |
Best case | 43% | |
Base case | 56% | |
Worst case | 75% | |
7. Risk of secondary transmission | 18% | 31, 32 |
8. Missed school days (per child per year) attributed to influenza | 0.79 | 29, 30 |
9. Percentage of caregivers who miss work to care for sick child | 53% | 6 |
Cost‐Effectiveness Analysis
The purpose of the cost‐effectiveness analysis was to evaluate potential cost savings attributable to improvement in vaccination delivery (UTD status). Direct and indirect costs were obtained by review of the current published literature pertaining to costs of influenza and influenza vaccination. The key assumptions for the cost‐effectiveness analysis are present in Table 1.
Vaccine Efficacy and Cost
Studies of influenza vaccine efficacy in those with asthma have had widely variable results on clinical outcomes.1923 Therefore, for the purpose of these analyses, we used a best‐case, worst‐case, base‐case vaccine efficacy approach as previously described.15 The vaccine efficacy base case was estimated as 56%, best case as 75%, and worst case as 43%. The primary cost‐effectiveness analysis was performed with the base‐case efficacy. Sensitivity analysis was performed by varying the vaccine efficacy between worst‐case efficacy and best‐case efficacy in order to determine effects on clinical outcomes and cost savings.
We determined the cost associated with vaccination assessment and delivery. Cost of only assessing a patient for vaccination without delivery was calculated as $5.32 on the basis of the assumption that a registered nurse (RN) would print a form (2 minutes), ask screening questions and wait for replies (5 minutes), and fax the form/order to the pharmacy and/or place it in the medical record (1 minute) at an RN salary of $40/hour. Assessment and administration of the vaccination was assumed to cost $18.84. This was based on additional nursing time of 10 minutes (1 minute to check arrival of the vaccination, 2 minutes to prepare it, 5 minutes to inject and dispose of it, and 1 minute to record delivery of the vaccination in the medical record) plus the cost of the vaccine (estimated as $6.75)24 and related supplies (estimated as $0.67 for the safety syringe, $0.01 for cotton balls, $0.01 for alcohol preps, and $0.065 for rubber gloves).25 The cost of vaccine and supplies was determined by reviewing pharmaceutical reference books and medical equipment billing Web sites. The indirect cost of delivery of the second vaccination in the outpatient setting to patients requiring 2 shots to become UTD was 3 hours of missed work per caregiver.
Influenza Prevalence and Morbidity
The prevalence of influenza in school‐age children is estimated at 40%54% among those not at high risk during influenza season.1 For this analysis we used 45%. Morbidity associated with influenza has been estimated as 120200 clinic visits and 65140 antibiotic prescriptions per 1000 children per year.9 This analysis used 150 clinic visits and 100 antibiotic prescriptions per 1000 children. The rate of hospitalization for influenza of high‐risk children per 100,000 is 1900 for those aged 0‐11 months, 800 for those aged 12 years, 320 for those aged 34 years, and 92 for those aged 514 years.8, 11 The risk of hospitalization for the model's hypothetical cohort was age‐adjusted using the age distribution found in the HCUP KID for children discharged with a primary diagnosis of asthma. The age distribution of children in the HCUP KID was 34% were 12 years, 15% were 34 years, and 51% were 514 years.18 Children aged 011 months were excluded because of the low likelihood that this age group was at risk for influenza complicated by asthma. The age‐adjusted risk for the hypothetical cohort in this analysis was 360 hospitalizations/100,000 children.
To investigate the effect of year‐to‐year variation in influenza prevalence and morbidity, the lowest and then highest estimates for these measures from previous studies were used in the model to determine the effect on the outcomes of cost per vaccination and cost per assessment. During this analysis all the measures were simultaneously entered at their lowest estimates to simulate a less active influenza season and then at their highest estimates to simulate a more active influenza season. The lowest and highest estimates of these assumptions were: influenza prevalence, 31% and 54%3; number of clinic visits, 120 to 200 per 1000 children8; and number of antibiotic prescriptions, 65 to 140 per 1000 children.8 No range was found in the literature for risk of hospitalization. Therefore, the standard 25% decrease and 25% increase were used as the lowest and highest estimates. The results of this analysis demonstrated the range of cost savings per year based on the prevalence and morbidity of influenza.
Direct and Indirect Cost of Influenza
Cost data were collected from multiple studies and inflated to 2006 dollars using the Bureau of Labor Statistics consumer price index for medical care and medical care services.26 The costs of a clinic visit and antibiotic prescription were assumed to be $5127 and $9.91,28 which were inflated to 2006 dollars: $70.09 and $13.76, respectively. Used as the mean cost for hospitalization in this analysis ($15,2695) was the more conservative estimate from 2 recent articles on determining the cost of hospitalization of high‐risk pediatric patients with influenza‐related illness.4, 5 Number of school days missed by children attributed to influenza has been previously established as 0.79 days per child per year.29, 30 The cost of a caregiver missing work to care for a child was assumed to be the median hourly wage of a child care provider according to the Bureau of Labor and Statistics 2005, which was $9.47/hour, or $9.94/hour in 2006 dollars. The model assumed that there was a 53% chance that a caregiver would need to miss work for a sick child.6 In addition, there was a risk of secondary transmission of 18%31, 32 to an average of 1.72 adults (percentage of 2‐parent households = 72%15). The cost of an adult secondary infection was $65.25, inflated to $99.49 in 2006 dollars. The estimate for the cost of a secondary infection was derived from a article by Nichol et al., who investigated the cost of influenza for healthy working adults.33 The total savings per vaccination of a healthy working adult was estimated as $46.85. This estimate included $10, the cost of the vaccination; $0.69, the cost of the side effects of the vaccine; and $7.71, the cost of missing work in order to receive the vaccination. A secondary case of influenza would be prevented without incurring these additional costs of vaccination, and therefore $65.25 (inflated to $99.49) was used in this analysis.
RESULTS
With existing data showing that only 29% of those with asthma are UTD on influenza vaccine in a given year, our decision analysis demonstrated that even modest increases in the rate of screening for influenza vaccine status among hospitalized patients with asthma can result in clinically significant increases in the rate of vaccine delivery. For example, screening of just 20% of hospitalized patients with asthma would result in 35% of the children ultimately being up to date for that influenza season, a 20% overall improvement. In the same manner, a 40% assessment rate would result in 41% of children ultimately becoming UTD, a 60% assessment rate in 47% ultimately becoming UTD, an 80% assessment rate in 53% ultimately becoming UTD, and a 100% assessment in 59% ultimately becoming UTD, which is double the baseline rate (Table 2 ).
Percent assessment | Vaccinations delivered (per 1000 patients) | Postintervention UTD |
---|---|---|
| ||
No increased assessment | None inpatient | 29% |
10% | 59 | 32% |
20% | 119 | 35% |
30% | 178 | 38% |
40% | 237 | 41% |
50% | 252 | 44% |
60% | 356 | 47% |
70% | 415 | 50% |
80% | 475 | 53% |
90% | 534 | 56% |
100% | 593 | 59% |
Direct cost of influenza | Indirect cost of influenza | Cost of inpatient vaccination | Total | Savings | |
---|---|---|---|---|---|
No increased assessment | $56.48 | $39.51 | $ 0 | $95.99 | Base |
Intervention to increase assessment | $45.24 | $31.64 | $13.66 | $90.54 | $5.45 |
This cost analysis demonstrated cost savings of $5.45 per child assessed and $9.19 per child vaccinated (Table 3). The cost savings per child assessed was lower than per child vaccinated because of including the costs of assessment without delivery of vaccination. This estimated savings is lower than the previously published $34.79 per vaccination of school‐age children in a group setting but more comparable to the cost savings of $3.99 in an individual‐initiated setting.15
The cost savings with this intervention depends on the vaccine's efficacy, and efficacy can vary as a function of whether it is an epidemic season and the degree of matching between the prevalent influenza strains and the vaccine strains. At higher predicted levels of vaccination efficacy, UTD patients have improved, less negative outcomes from influenza, and the intervention results in higher cost savings (cost savings with base‐case vaccine efficacy of $5.45/child assessed increases to $11.93/child assessed with best‐case vaccine efficacy). No current research accurately predicts vaccine efficacy for each outcome in pediatric patients with asthma. Therefore, this study design varied vaccine efficacy during sensitivity analyses among 3 potential vaccine efficacy scenarios (best‐, base‐, and worst‐case scenarios).15 Varying the vaccine efficacy to the best‐case scenario resulted in a cost savings of $11.93 per child assessed and $20.13 per child vaccinated. Dropping the efficacy to the worst‐case scenario resulted in cost savings of $1.01 per child assessed and $1.71 per child vaccinated. If the vaccine efficacy was 40%, the intervention was cost neutral. It should be remembered that the goal of the health care system is to generate good health. A project that is cost neutral (cost of vaccination intervention = cost savings from illness prevention), such as this intervention with a vaccine efficacy of 40%, may still be considered cost effective because of the improvement in clinical outcomes. At a vaccine efficacy of 40%, this model predicts that clinic visits will decrease by 19/1000 children, unwarranted antibiotics will decrease by 12/1000 children, hospitalizations will decrease by 4/10,000 children, and secondary infections of adult caregivers will decrease by 17/1000 children, suggesting an overall benefit to the health care system and to patients.
The clinical improvement predicted by this model with base‐case vaccine efficacy and 100% assessment include: a decrease in clinic visits of 27/1000 children, decrease in antibiotic use of 75/1000 children, decrease in hospitalizations of 6/10,000 children, decrease in missed school days of 132/1000 children, and a decrease in secondary infections of adult caregivers of 23/1000 children assessed.
The total cost of vaccination in this intervention was estimated as $10,593 per 1000 children. A hospital that discharges fewer than 1000 children with asthma per year would accrue less direct cost yearly with this intervention ($1059 per 100 children). A portion of this cost could be recuperated through reimbursement for influenza vaccination via insurance payers and/or government programs to reimburse for childhood vaccinations.
The results from the investigation into the effect of year‐to‐year variation in influenza prevalence and morbidity (by using the lowest and highest estimates found in the literature) demonstrated the model to be sensitive to these estimates, but the intervention maintained a cost savings. In a year with low influenza prevalence and morbidity, the cost savings decreases to $1.89 per child assessed and $3.20 per child vaccinated. In a year with high influenza prevalence and morbidity, the cost savings would increase to $8.75 per child assessed and $14.77 per child vaccinated. This finding of cost savings even with low influenza prevalence and morbidity is consistent with previous studies of influenza vaccination of pediatric patients in group‐based settings.15
For all other estimates, traditional sensitivity analysis was performed, and the results continued to show cost savings during this procedure, suggesting that the conclusions based on this model are generalizable and robust.
DISCUSSION
Universal screening for influenza vaccine status and then delivery of the vaccine to those not UTD among hospitalized children with asthma has the potential to increase the percentage of these children receiving the influenza vaccine and to reduce costs. This model suggests that with 100% assessment of this difficult‐to‐reach population for being UTD with the influenza vaccine, it would be possible to achieve a vaccination rate of 59%, doubling the current yearly receipt of influenza vaccination of children admitted secondary to asthma. However, universal screening is not imperative to achieve significant clinical improvement and cost savings. The cost‐effectiveness analysis demonstrated that the cost savings would be $5.45 per child assessed and $9.19 per child vaccinated.
The cost savings in this analysis ($9.19/child vaccinated) is favorable but lower than a previous analysis of influenza vaccination of children in an unspecified group‐based setting ($34.79/child vaccinated).15 Multiple factors contributed to our lower cost savings. First, our model accounts for the cost of children becoming partially vaccinated, incurring the full cost for 1 vaccination without the resulting clinical benefit. Second, the model accommodates for nursing assessment without vaccination delivery. Most importantly, this model estimates direct cost of vaccination delivery more conservatively than other group‐based estimates ($18.84 vs. $4.31).15 Previous cost‐effective analyses of influenza vaccination referenced 2 articles from the mid‐1990s that used a direct cost for 1 vaccination of $4 for group‐based vaccination in an HMO34 and $10 for individual‐initiated vaccination.33 Our estimate of vaccine cost is more conservative than these studies but more likely to represent the cost of vaccination assessment and delivery in an acute‐care hospital setting.
As the model was created, it became apparent that vaccination in the inpatient setting would accrue less indirect costs as compared with an individual‐initiated outpatient setting. This is a result of there not being any incrementally increased loss of work by the family/caregiver in order to be vaccinated above that lost because of hospitalization of the child for asthma. This finding supports that there is cost savings by vaccinating pediatric patients while hospitalized and is consistent with previous literature on this subject.15
The use of modeling techniques to evaluate inpatient interventions has many benefits. Modeling techniques permit the generation of synthetic trials by utilizing the combined published data from multiple studies. This permits the investigator to apply findings to other populations with different risks or prognoses and to other settings, to extend the impact over time, and to display multiple outcomes together. This technique has the potential to be used in investigations of the theoretical benefit of a specific quality improvement intervention in an inpatient setting without the extensive cost of a clinical trial. In fact, preliminary analysis with modeling could improve the cost efficiency of quality improvement research. Modeling studies could demonstrate which interventions are most likely to generate cost savings and direct clinical investigation and funding.
Limitations of this project included the possibility of vaccine efficacy changing from year to year depending on the similarity of the viruses in the vaccine with the predominant infectious strains circulating that year, as observed in previous studies.11 In a year with a poor match, the cost savings would be reduced compared with that in years with a good match. In addition, influenza prevalence and morbidity affects this model and the cost savings of the intervention, but the analysis using the lowest estimates of prevalence and morbidity continued to demonstrate that the intervention was saving costs. This continued cost savings for a group‐based vaccination intervention during years when there is low prevalence and morbidity is consistent with previous reports in the literature for pediatric patients.15 Modeling techniques decrease the effect of year‐to‐year variation in vaccination match, prevalence, and morbidity and estimate results based on an average over multiple influenza seasons.
For children with asthma, the data are not well established about influenza vaccination's ability to improve specific clinical outcomes attributed to influenza illness (missed school days, clinic visits, antibiotic prescriptions, hospitalizations, and secondary infections). Acknowledging the limitations of these data, our model estimates the intervention's improvement in clinical outcomes by using published data on vaccine efficacy and varying the vaccine efficacy from worst case to base case to best case. In addition, with a vaccine efficacy of 40%, this intervention would be cost neutral. Regardless of concerns about vaccine efficacy, the CDC/ACIP recommends finding missed opportunities for vaccination to improve vaccination delivery and the ultimate UTD status of high‐risk children. Recently, the CDC/ACIP stated that influenza vaccination coverage among children with asthma is inadequate and that opportunities for vaccination during health‐care provider visits likely are being missed.12 This intervention to assess and deliver influenza vaccination to pediatric patients while hospitalized for an asthma exacerbation would allow all hospitals that treat children to participate in reaching the goal of the CDC/ACIP to reduce missed opportunities for influenza vaccination.
The direct cost of inpatient vaccination in this intervention would fall primarily on the hospital implementing the intervention ($10,593 per 1000 children). However, the Vaccines for Children program exists to offset the cost of immunizations to both patients and providers for the uninsured and children receiving Medicaid.11 If properly implemented by participating hospitals, this program would improve reimbursement for vaccination and therefore shift the cost away from the individual hospital. For example, if the intervention cost borne by a hospital were decreased by the direct cost of vaccination alone ($6.75), this model predicts the cost of a program per 1000 children would decrease from $10,593 to $7190. This cost reduction would depend on the percentage of patients uninsured or on Medicaid at a given hospital. Another approach to shifting cost from individual hospitals would be for policy makers to consider influenza vaccination of these high‐risk pediatric patients as a performance/quality measure and associate the measure with improved reimbursement to hospitals in order to compensate for the cost of the vaccination intervention.
CONCLUSIONS
Influenza immunization is an accepted method of prevention and is underutilized in children with asthma.12 This model suggests that an inpatient program to deliver influenza vaccination to hospitalized pediatric patients with asthma would be beneficial by producing better health outcomes and reducing health care costs. Further research should be performed to verify the assumptions used in this analysis for children with asthma.
Acknowledgements
The authors acknowledge all the individuals who participated in the Academic Generalist Fellowship in Health Services Research at the Medical University of South Carolina for their direct and indirect participation in the study.
- Longitudinal studies of types a and b influenza among Seattle schoolchildren and families.J Infect Dis.1976;134:362–369. , , .
- Influenza virus infections in infants.Pediatr Infect Dis J.1997;16:1065–1068. , , , , .
- Economic impact of influenza vaccination in preschool children.Pediatrics.2000;106:973–976. , .
- Cost of influenza hospitalization at a tertiary care children's hospital and its impact on the cost‐benefit analysis of the recommendation for universal influenza immunization in children age 6 to 23 months.J Pediatr.2005;147:807–811. , .
- Direct medical cost of influenza‐related hospitalizations in children.Pediatrics.2006;118:e1321–e1327. , , , , .
- School absenteeism, parental work loss, and acceptance of childhood influenza vaccination.Am J Med Sci.2001;321:178–180. , , , .
- Illness among schoolchildren during influenza season: effect on school absenteeism, parental absenteeism from work, and secondary illness in families.Arch Pediatr Adolesc Med.2002;156:986–991. , , .
- The effect of influenza on hospitalizations, outpatient visits, and courses of antibiotics in children.N Engl J Med.2000;342:225–231. , , , , .
- The burden of influenza illness in children with asthma and other chronic medical conditions.J Pediatr.2000;137:856–864. , , , .
- The underrecognized burden of influenza in young children.N Engl J Med.2006;355(1):31–40. , , , et al.
- Prevention and control of influenza: recommendations of the Advisory Committee on Immunization Practices (ACIP).MMWR Recomm Rep.2006;55(RR‐10):1–42. , , , , , .
- Influenza vaccination coverage among children with asthma—United States, 2004–05 influenza season.MMWR Weekly.2007;56(9):193–196. , , , .
- Effect of prednisone on response to influenza virus vaccine in asthmatic children.Arch Pediatr Adolesc Med.1998;152:1191–1195. , , , , .
- Influenza vaccination of children during acute asthma exacerbation and concurrent prednisone therapy.Pediatrics.1996;98(2 Pt 1):196–200. , , , , .
- Potential cost savings attributable to influenza vaccination of school‐aged children.Pediatrics.1999;103:e73. , , .
- Providing immunizations in a pediatric emergency department: underimmunization rates and parental acceptance.Pediatr Emerg Care.1999;15:255–259. .
- Improving influenza vaccination rates in children with asthma: a test of a computerized reminder system and an analysis of factors predicting vaccination compliance.Pediatrics.1992;90:871–875. , , , .
- HealthCare Cost and Utilization Project: Kids' Inpatient Database (KID)2000. Agency for Healthcare Research and Quality.
- Influenza vaccination in patients with asthma: effect on the frequency of upper respiratory tract infections and exacerbations.J Asthma.2004;41:279–283. , , , , .
- Clinical effectiveness of conventional influenza vaccination in asthmatic children.Epidemiol Infect.2002;128:205–211. , , , , , .
- Influenza vaccination in asthmatic children: effects on quality of life and symptoms.Eur Respir J.2004;24:925–931. , , , et al.
- Influenza vaccination in children with asthma: randomized double‐blind placebo‐controlled trial.Am J Respir Crit Care Med.2004;169:488–493. , , , et al.
- Does influenza vaccination prevent asthma exacerbations in children?J Pediatr.2001;138:306–310. , , , et al.
- Red Book: Pharmacy's Fundamental Reference.2006 ed: Thomson Healthcare2006.
- Flu Vaccine Pre‐Book 2007‐08. Dubin Medical Incorporation. Available at: http://www.dubinmedical.com/news/index.htm. Accessed June 21,2007.
- Bureau of Labor Statistics Data. U.S. Department of Labor: Bureau of Labor Statistics; 2006. Available at: http://www.bls.gov. Accessed May 18,2007.
- Association Medical Association.Socioeconomics of medical practice, 1997.Am Med Assoc.1997;69(89):107.
- The Cost of antibiotics in treating respiratory tract infections in a Medicaid population.Arch Fam Med.1998;7:45–49. , .
- Comparison of US inactivated split‐virus and Russian attenuated, cold‐adapted trivalent influenza vaccines in Russian schoolchildren.JInfect Dis.1996;173:453–456. , , .
- Current estimates from the National Health Interview Survey, 1994. National Health Statistics.Vital Health Stat.1995;10:193. , .
- Influenza B virus infections in the community and the family. The epidemics of 1976‐1977 and 1979‐1980 in Houston, Texas.Am J Epidemiol.1983;118:313–325. , , , , , .
- Emergence and apparent transmission of rimantidine‐resistant influenza a virus in families.N Engl J Med.1989;321:1696–1702. , , , , , .
- The effectiveness ofvaccination against influenza in healthy, working adults.N Engl J Med.1995;333:889–893. , , , et al.
- The efficacy and cost effectiveness of vaccination against influenza among elderly persons living in the community.N Engl J Med.1994;331:778–784. , , , .
Influenza is estimated to infect up to 40%‐54% of school‐age children during an influenza season.1, 2 Influenza infections result in missed school days, visits to primary care providers, prescriptions for antibiotics, hospitalizations, and secondary infections.310 Although influenza‐related mortality of pediatric patients is rare, influenza‐related morbidity of children and those secondarily infected is common and partially preventable.11
For at‐risk individuals, immunization against influenza is the best method of preventing morbidity. The Centers for Disease Control's Advisory Committee on Immunization Practice (CDC/ACIP) considers children with asthma at high risk of morbidity from influenza and since 1964 has recommended delivery of inactivated influenza vaccination to this population of patients. Even with this recommendation, the current level of adherence to yearly influenza vaccination of children with asthma is approximately 29%, according to the CDC analysis of the National Health Interview Survey.12 Although pediatric providers may prefer to provide preventive care in an ambulatory setting, the CDC/ACIP emphasizes the need for vaccination delivery wherever possible, including in acute‐care hospitals.11 Particularly in an acute‐care setting, vaccinating patients who are having an exacerbation of their asthma and who may have a compromised immune response may raise concerns. However, the vaccine has been shown to be efficacious even with pediatric asthmatics on a short course of corticosteroids.13, 14 Utilization of the inpatient visit as an opportunity to implement evidence‐based preventive care to pediatric asthmatics could have a beneficial effect on patient outcomes. Group‐based settings (school or health fair) are more cost effective than individual‐initiated settings (primary care) for influenza vaccination of pediatric patients.15 However, we found no studies investigating the effectiveness of vaccinating high‐risk patients in an acute‐care setting.
This study used established modeling techniques (decision and cost‐effectiveness analyses) to determine the potential clinical and cost benefits of vaccinating children against influenza during an asthma exacerbation in an acute‐care setting. We used decision analysis to determine the effectiveness of the intervention in improving the up‐to‐date (UTD) status of a hypothetical population. Based on this improvement in vaccination delivery, we completed a cost‐effectiveness analysis to determine direct and indirect costs, improvement in clinical outcomes attributable to influenza (clinic visits, antibiotic use, hospitalization, and secondary infections), and cost savings attributable to the intervention.
METHODS
Decision Analysis
We generated a decision tree to represent an inpatient intervention to assess and deliver influenza vaccination to children with asthma in acute‐care hospitals (Fig. 1). The design of the decision tree intentionally began with a decision node in order to introduce different assessment rates. This approach was used in order to accurately represent an inpatient intervention (where 100% assessment is not always attained) and to assess how less‐than‐perfect rates of assessment of influenza vaccine status would affect the success of the intervention. Once the decision tree was created, we surveyed the literature to obtain data on the assumptions of the decision tree (See Table 1 for assumptions of decision analysis with corresponding numbering in Figure 1 Decision Tree) including percentage of children with asthma who receive the influenza vaccine (29%),12 percentages of patients and caregivers who would agree to be vaccinated in an acute‐care settings (71%),16 and rates of response to vaccine reminder recall systems in a primary care setting (30%)17 in order to account for populations that need a second dose of vaccine to become UTD. To estimate the percentage of children who would require a second vaccination at 4 weeks, we utilized the Healthcare Cost and Utilization Project Kids Inpatient Database (HCUP KID).18 Seventy‐three percent of children younger than 9 years old in the HCUP KID were discharged with a diagnosis of asthma. These patients would require a second vaccination after 4 weeks if they had not previously been immunized. After all the estimates were placed in the decision tree, a hypothetical cohort of children with asthma was introduced, and improvement in UTD status after the intervention was determined.
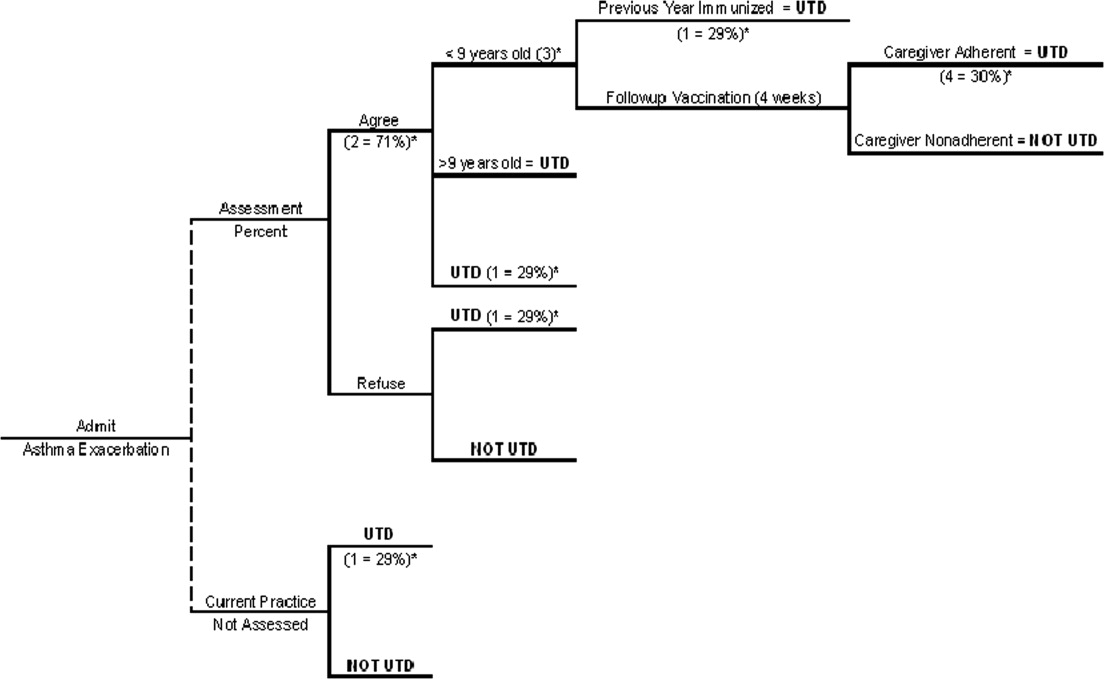
Reference | ||
---|---|---|
Decision analysis | ||
1. Percentage of asthmatics UTD with influenza vaccination without increased inpatient assessment | 29% | 12 |
2. Percentage of caregivers who agree with vaccination in an acute‐care setting | 71% | 16 |
3. Percentage of asthma exacerbation discharges <9 years old | 73% | 18 |
4. Percentage of caregivers who respond to reminder‐recall systems for outpatient vaccination | 30% | 17 |
Cost‐effectiveness analysis | ||
5. Prevalence of influenza in school‐age children over 1 season | 45% | 1 |
6. Vaccine efficacy | 15 | |
Best case | 43% | |
Base case | 56% | |
Worst case | 75% | |
7. Risk of secondary transmission | 18% | 31, 32 |
8. Missed school days (per child per year) attributed to influenza | 0.79 | 29, 30 |
9. Percentage of caregivers who miss work to care for sick child | 53% | 6 |
Cost‐Effectiveness Analysis
The purpose of the cost‐effectiveness analysis was to evaluate potential cost savings attributable to improvement in vaccination delivery (UTD status). Direct and indirect costs were obtained by review of the current published literature pertaining to costs of influenza and influenza vaccination. The key assumptions for the cost‐effectiveness analysis are present in Table 1.
Vaccine Efficacy and Cost
Studies of influenza vaccine efficacy in those with asthma have had widely variable results on clinical outcomes.1923 Therefore, for the purpose of these analyses, we used a best‐case, worst‐case, base‐case vaccine efficacy approach as previously described.15 The vaccine efficacy base case was estimated as 56%, best case as 75%, and worst case as 43%. The primary cost‐effectiveness analysis was performed with the base‐case efficacy. Sensitivity analysis was performed by varying the vaccine efficacy between worst‐case efficacy and best‐case efficacy in order to determine effects on clinical outcomes and cost savings.
We determined the cost associated with vaccination assessment and delivery. Cost of only assessing a patient for vaccination without delivery was calculated as $5.32 on the basis of the assumption that a registered nurse (RN) would print a form (2 minutes), ask screening questions and wait for replies (5 minutes), and fax the form/order to the pharmacy and/or place it in the medical record (1 minute) at an RN salary of $40/hour. Assessment and administration of the vaccination was assumed to cost $18.84. This was based on additional nursing time of 10 minutes (1 minute to check arrival of the vaccination, 2 minutes to prepare it, 5 minutes to inject and dispose of it, and 1 minute to record delivery of the vaccination in the medical record) plus the cost of the vaccine (estimated as $6.75)24 and related supplies (estimated as $0.67 for the safety syringe, $0.01 for cotton balls, $0.01 for alcohol preps, and $0.065 for rubber gloves).25 The cost of vaccine and supplies was determined by reviewing pharmaceutical reference books and medical equipment billing Web sites. The indirect cost of delivery of the second vaccination in the outpatient setting to patients requiring 2 shots to become UTD was 3 hours of missed work per caregiver.
Influenza Prevalence and Morbidity
The prevalence of influenza in school‐age children is estimated at 40%54% among those not at high risk during influenza season.1 For this analysis we used 45%. Morbidity associated with influenza has been estimated as 120200 clinic visits and 65140 antibiotic prescriptions per 1000 children per year.9 This analysis used 150 clinic visits and 100 antibiotic prescriptions per 1000 children. The rate of hospitalization for influenza of high‐risk children per 100,000 is 1900 for those aged 0‐11 months, 800 for those aged 12 years, 320 for those aged 34 years, and 92 for those aged 514 years.8, 11 The risk of hospitalization for the model's hypothetical cohort was age‐adjusted using the age distribution found in the HCUP KID for children discharged with a primary diagnosis of asthma. The age distribution of children in the HCUP KID was 34% were 12 years, 15% were 34 years, and 51% were 514 years.18 Children aged 011 months were excluded because of the low likelihood that this age group was at risk for influenza complicated by asthma. The age‐adjusted risk for the hypothetical cohort in this analysis was 360 hospitalizations/100,000 children.
To investigate the effect of year‐to‐year variation in influenza prevalence and morbidity, the lowest and then highest estimates for these measures from previous studies were used in the model to determine the effect on the outcomes of cost per vaccination and cost per assessment. During this analysis all the measures were simultaneously entered at their lowest estimates to simulate a less active influenza season and then at their highest estimates to simulate a more active influenza season. The lowest and highest estimates of these assumptions were: influenza prevalence, 31% and 54%3; number of clinic visits, 120 to 200 per 1000 children8; and number of antibiotic prescriptions, 65 to 140 per 1000 children.8 No range was found in the literature for risk of hospitalization. Therefore, the standard 25% decrease and 25% increase were used as the lowest and highest estimates. The results of this analysis demonstrated the range of cost savings per year based on the prevalence and morbidity of influenza.
Direct and Indirect Cost of Influenza
Cost data were collected from multiple studies and inflated to 2006 dollars using the Bureau of Labor Statistics consumer price index for medical care and medical care services.26 The costs of a clinic visit and antibiotic prescription were assumed to be $5127 and $9.91,28 which were inflated to 2006 dollars: $70.09 and $13.76, respectively. Used as the mean cost for hospitalization in this analysis ($15,2695) was the more conservative estimate from 2 recent articles on determining the cost of hospitalization of high‐risk pediatric patients with influenza‐related illness.4, 5 Number of school days missed by children attributed to influenza has been previously established as 0.79 days per child per year.29, 30 The cost of a caregiver missing work to care for a child was assumed to be the median hourly wage of a child care provider according to the Bureau of Labor and Statistics 2005, which was $9.47/hour, or $9.94/hour in 2006 dollars. The model assumed that there was a 53% chance that a caregiver would need to miss work for a sick child.6 In addition, there was a risk of secondary transmission of 18%31, 32 to an average of 1.72 adults (percentage of 2‐parent households = 72%15). The cost of an adult secondary infection was $65.25, inflated to $99.49 in 2006 dollars. The estimate for the cost of a secondary infection was derived from a article by Nichol et al., who investigated the cost of influenza for healthy working adults.33 The total savings per vaccination of a healthy working adult was estimated as $46.85. This estimate included $10, the cost of the vaccination; $0.69, the cost of the side effects of the vaccine; and $7.71, the cost of missing work in order to receive the vaccination. A secondary case of influenza would be prevented without incurring these additional costs of vaccination, and therefore $65.25 (inflated to $99.49) was used in this analysis.
RESULTS
With existing data showing that only 29% of those with asthma are UTD on influenza vaccine in a given year, our decision analysis demonstrated that even modest increases in the rate of screening for influenza vaccine status among hospitalized patients with asthma can result in clinically significant increases in the rate of vaccine delivery. For example, screening of just 20% of hospitalized patients with asthma would result in 35% of the children ultimately being up to date for that influenza season, a 20% overall improvement. In the same manner, a 40% assessment rate would result in 41% of children ultimately becoming UTD, a 60% assessment rate in 47% ultimately becoming UTD, an 80% assessment rate in 53% ultimately becoming UTD, and a 100% assessment in 59% ultimately becoming UTD, which is double the baseline rate (Table 2 ).
Percent assessment | Vaccinations delivered (per 1000 patients) | Postintervention UTD |
---|---|---|
| ||
No increased assessment | None inpatient | 29% |
10% | 59 | 32% |
20% | 119 | 35% |
30% | 178 | 38% |
40% | 237 | 41% |
50% | 252 | 44% |
60% | 356 | 47% |
70% | 415 | 50% |
80% | 475 | 53% |
90% | 534 | 56% |
100% | 593 | 59% |
Direct cost of influenza | Indirect cost of influenza | Cost of inpatient vaccination | Total | Savings | |
---|---|---|---|---|---|
No increased assessment | $56.48 | $39.51 | $ 0 | $95.99 | Base |
Intervention to increase assessment | $45.24 | $31.64 | $13.66 | $90.54 | $5.45 |
This cost analysis demonstrated cost savings of $5.45 per child assessed and $9.19 per child vaccinated (Table 3). The cost savings per child assessed was lower than per child vaccinated because of including the costs of assessment without delivery of vaccination. This estimated savings is lower than the previously published $34.79 per vaccination of school‐age children in a group setting but more comparable to the cost savings of $3.99 in an individual‐initiated setting.15
The cost savings with this intervention depends on the vaccine's efficacy, and efficacy can vary as a function of whether it is an epidemic season and the degree of matching between the prevalent influenza strains and the vaccine strains. At higher predicted levels of vaccination efficacy, UTD patients have improved, less negative outcomes from influenza, and the intervention results in higher cost savings (cost savings with base‐case vaccine efficacy of $5.45/child assessed increases to $11.93/child assessed with best‐case vaccine efficacy). No current research accurately predicts vaccine efficacy for each outcome in pediatric patients with asthma. Therefore, this study design varied vaccine efficacy during sensitivity analyses among 3 potential vaccine efficacy scenarios (best‐, base‐, and worst‐case scenarios).15 Varying the vaccine efficacy to the best‐case scenario resulted in a cost savings of $11.93 per child assessed and $20.13 per child vaccinated. Dropping the efficacy to the worst‐case scenario resulted in cost savings of $1.01 per child assessed and $1.71 per child vaccinated. If the vaccine efficacy was 40%, the intervention was cost neutral. It should be remembered that the goal of the health care system is to generate good health. A project that is cost neutral (cost of vaccination intervention = cost savings from illness prevention), such as this intervention with a vaccine efficacy of 40%, may still be considered cost effective because of the improvement in clinical outcomes. At a vaccine efficacy of 40%, this model predicts that clinic visits will decrease by 19/1000 children, unwarranted antibiotics will decrease by 12/1000 children, hospitalizations will decrease by 4/10,000 children, and secondary infections of adult caregivers will decrease by 17/1000 children, suggesting an overall benefit to the health care system and to patients.
The clinical improvement predicted by this model with base‐case vaccine efficacy and 100% assessment include: a decrease in clinic visits of 27/1000 children, decrease in antibiotic use of 75/1000 children, decrease in hospitalizations of 6/10,000 children, decrease in missed school days of 132/1000 children, and a decrease in secondary infections of adult caregivers of 23/1000 children assessed.
The total cost of vaccination in this intervention was estimated as $10,593 per 1000 children. A hospital that discharges fewer than 1000 children with asthma per year would accrue less direct cost yearly with this intervention ($1059 per 100 children). A portion of this cost could be recuperated through reimbursement for influenza vaccination via insurance payers and/or government programs to reimburse for childhood vaccinations.
The results from the investigation into the effect of year‐to‐year variation in influenza prevalence and morbidity (by using the lowest and highest estimates found in the literature) demonstrated the model to be sensitive to these estimates, but the intervention maintained a cost savings. In a year with low influenza prevalence and morbidity, the cost savings decreases to $1.89 per child assessed and $3.20 per child vaccinated. In a year with high influenza prevalence and morbidity, the cost savings would increase to $8.75 per child assessed and $14.77 per child vaccinated. This finding of cost savings even with low influenza prevalence and morbidity is consistent with previous studies of influenza vaccination of pediatric patients in group‐based settings.15
For all other estimates, traditional sensitivity analysis was performed, and the results continued to show cost savings during this procedure, suggesting that the conclusions based on this model are generalizable and robust.
DISCUSSION
Universal screening for influenza vaccine status and then delivery of the vaccine to those not UTD among hospitalized children with asthma has the potential to increase the percentage of these children receiving the influenza vaccine and to reduce costs. This model suggests that with 100% assessment of this difficult‐to‐reach population for being UTD with the influenza vaccine, it would be possible to achieve a vaccination rate of 59%, doubling the current yearly receipt of influenza vaccination of children admitted secondary to asthma. However, universal screening is not imperative to achieve significant clinical improvement and cost savings. The cost‐effectiveness analysis demonstrated that the cost savings would be $5.45 per child assessed and $9.19 per child vaccinated.
The cost savings in this analysis ($9.19/child vaccinated) is favorable but lower than a previous analysis of influenza vaccination of children in an unspecified group‐based setting ($34.79/child vaccinated).15 Multiple factors contributed to our lower cost savings. First, our model accounts for the cost of children becoming partially vaccinated, incurring the full cost for 1 vaccination without the resulting clinical benefit. Second, the model accommodates for nursing assessment without vaccination delivery. Most importantly, this model estimates direct cost of vaccination delivery more conservatively than other group‐based estimates ($18.84 vs. $4.31).15 Previous cost‐effective analyses of influenza vaccination referenced 2 articles from the mid‐1990s that used a direct cost for 1 vaccination of $4 for group‐based vaccination in an HMO34 and $10 for individual‐initiated vaccination.33 Our estimate of vaccine cost is more conservative than these studies but more likely to represent the cost of vaccination assessment and delivery in an acute‐care hospital setting.
As the model was created, it became apparent that vaccination in the inpatient setting would accrue less indirect costs as compared with an individual‐initiated outpatient setting. This is a result of there not being any incrementally increased loss of work by the family/caregiver in order to be vaccinated above that lost because of hospitalization of the child for asthma. This finding supports that there is cost savings by vaccinating pediatric patients while hospitalized and is consistent with previous literature on this subject.15
The use of modeling techniques to evaluate inpatient interventions has many benefits. Modeling techniques permit the generation of synthetic trials by utilizing the combined published data from multiple studies. This permits the investigator to apply findings to other populations with different risks or prognoses and to other settings, to extend the impact over time, and to display multiple outcomes together. This technique has the potential to be used in investigations of the theoretical benefit of a specific quality improvement intervention in an inpatient setting without the extensive cost of a clinical trial. In fact, preliminary analysis with modeling could improve the cost efficiency of quality improvement research. Modeling studies could demonstrate which interventions are most likely to generate cost savings and direct clinical investigation and funding.
Limitations of this project included the possibility of vaccine efficacy changing from year to year depending on the similarity of the viruses in the vaccine with the predominant infectious strains circulating that year, as observed in previous studies.11 In a year with a poor match, the cost savings would be reduced compared with that in years with a good match. In addition, influenza prevalence and morbidity affects this model and the cost savings of the intervention, but the analysis using the lowest estimates of prevalence and morbidity continued to demonstrate that the intervention was saving costs. This continued cost savings for a group‐based vaccination intervention during years when there is low prevalence and morbidity is consistent with previous reports in the literature for pediatric patients.15 Modeling techniques decrease the effect of year‐to‐year variation in vaccination match, prevalence, and morbidity and estimate results based on an average over multiple influenza seasons.
For children with asthma, the data are not well established about influenza vaccination's ability to improve specific clinical outcomes attributed to influenza illness (missed school days, clinic visits, antibiotic prescriptions, hospitalizations, and secondary infections). Acknowledging the limitations of these data, our model estimates the intervention's improvement in clinical outcomes by using published data on vaccine efficacy and varying the vaccine efficacy from worst case to base case to best case. In addition, with a vaccine efficacy of 40%, this intervention would be cost neutral. Regardless of concerns about vaccine efficacy, the CDC/ACIP recommends finding missed opportunities for vaccination to improve vaccination delivery and the ultimate UTD status of high‐risk children. Recently, the CDC/ACIP stated that influenza vaccination coverage among children with asthma is inadequate and that opportunities for vaccination during health‐care provider visits likely are being missed.12 This intervention to assess and deliver influenza vaccination to pediatric patients while hospitalized for an asthma exacerbation would allow all hospitals that treat children to participate in reaching the goal of the CDC/ACIP to reduce missed opportunities for influenza vaccination.
The direct cost of inpatient vaccination in this intervention would fall primarily on the hospital implementing the intervention ($10,593 per 1000 children). However, the Vaccines for Children program exists to offset the cost of immunizations to both patients and providers for the uninsured and children receiving Medicaid.11 If properly implemented by participating hospitals, this program would improve reimbursement for vaccination and therefore shift the cost away from the individual hospital. For example, if the intervention cost borne by a hospital were decreased by the direct cost of vaccination alone ($6.75), this model predicts the cost of a program per 1000 children would decrease from $10,593 to $7190. This cost reduction would depend on the percentage of patients uninsured or on Medicaid at a given hospital. Another approach to shifting cost from individual hospitals would be for policy makers to consider influenza vaccination of these high‐risk pediatric patients as a performance/quality measure and associate the measure with improved reimbursement to hospitals in order to compensate for the cost of the vaccination intervention.
CONCLUSIONS
Influenza immunization is an accepted method of prevention and is underutilized in children with asthma.12 This model suggests that an inpatient program to deliver influenza vaccination to hospitalized pediatric patients with asthma would be beneficial by producing better health outcomes and reducing health care costs. Further research should be performed to verify the assumptions used in this analysis for children with asthma.
Acknowledgements
The authors acknowledge all the individuals who participated in the Academic Generalist Fellowship in Health Services Research at the Medical University of South Carolina for their direct and indirect participation in the study.
Influenza is estimated to infect up to 40%‐54% of school‐age children during an influenza season.1, 2 Influenza infections result in missed school days, visits to primary care providers, prescriptions for antibiotics, hospitalizations, and secondary infections.310 Although influenza‐related mortality of pediatric patients is rare, influenza‐related morbidity of children and those secondarily infected is common and partially preventable.11
For at‐risk individuals, immunization against influenza is the best method of preventing morbidity. The Centers for Disease Control's Advisory Committee on Immunization Practice (CDC/ACIP) considers children with asthma at high risk of morbidity from influenza and since 1964 has recommended delivery of inactivated influenza vaccination to this population of patients. Even with this recommendation, the current level of adherence to yearly influenza vaccination of children with asthma is approximately 29%, according to the CDC analysis of the National Health Interview Survey.12 Although pediatric providers may prefer to provide preventive care in an ambulatory setting, the CDC/ACIP emphasizes the need for vaccination delivery wherever possible, including in acute‐care hospitals.11 Particularly in an acute‐care setting, vaccinating patients who are having an exacerbation of their asthma and who may have a compromised immune response may raise concerns. However, the vaccine has been shown to be efficacious even with pediatric asthmatics on a short course of corticosteroids.13, 14 Utilization of the inpatient visit as an opportunity to implement evidence‐based preventive care to pediatric asthmatics could have a beneficial effect on patient outcomes. Group‐based settings (school or health fair) are more cost effective than individual‐initiated settings (primary care) for influenza vaccination of pediatric patients.15 However, we found no studies investigating the effectiveness of vaccinating high‐risk patients in an acute‐care setting.
This study used established modeling techniques (decision and cost‐effectiveness analyses) to determine the potential clinical and cost benefits of vaccinating children against influenza during an asthma exacerbation in an acute‐care setting. We used decision analysis to determine the effectiveness of the intervention in improving the up‐to‐date (UTD) status of a hypothetical population. Based on this improvement in vaccination delivery, we completed a cost‐effectiveness analysis to determine direct and indirect costs, improvement in clinical outcomes attributable to influenza (clinic visits, antibiotic use, hospitalization, and secondary infections), and cost savings attributable to the intervention.
METHODS
Decision Analysis
We generated a decision tree to represent an inpatient intervention to assess and deliver influenza vaccination to children with asthma in acute‐care hospitals (Fig. 1). The design of the decision tree intentionally began with a decision node in order to introduce different assessment rates. This approach was used in order to accurately represent an inpatient intervention (where 100% assessment is not always attained) and to assess how less‐than‐perfect rates of assessment of influenza vaccine status would affect the success of the intervention. Once the decision tree was created, we surveyed the literature to obtain data on the assumptions of the decision tree (See Table 1 for assumptions of decision analysis with corresponding numbering in Figure 1 Decision Tree) including percentage of children with asthma who receive the influenza vaccine (29%),12 percentages of patients and caregivers who would agree to be vaccinated in an acute‐care settings (71%),16 and rates of response to vaccine reminder recall systems in a primary care setting (30%)17 in order to account for populations that need a second dose of vaccine to become UTD. To estimate the percentage of children who would require a second vaccination at 4 weeks, we utilized the Healthcare Cost and Utilization Project Kids Inpatient Database (HCUP KID).18 Seventy‐three percent of children younger than 9 years old in the HCUP KID were discharged with a diagnosis of asthma. These patients would require a second vaccination after 4 weeks if they had not previously been immunized. After all the estimates were placed in the decision tree, a hypothetical cohort of children with asthma was introduced, and improvement in UTD status after the intervention was determined.
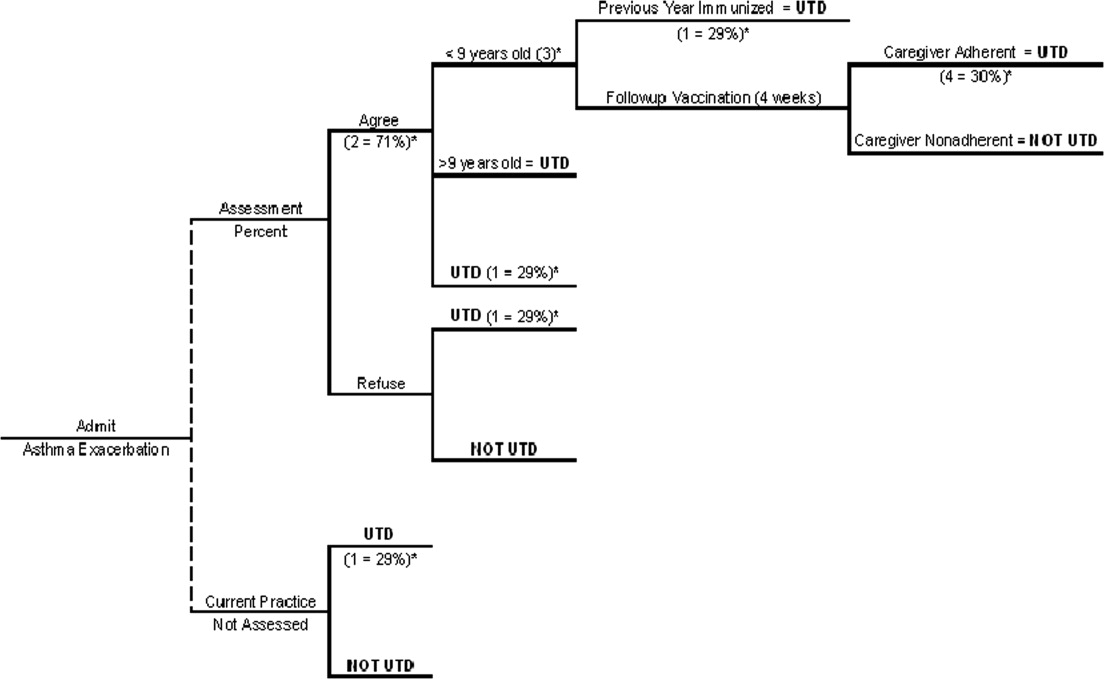
Reference | ||
---|---|---|
Decision analysis | ||
1. Percentage of asthmatics UTD with influenza vaccination without increased inpatient assessment | 29% | 12 |
2. Percentage of caregivers who agree with vaccination in an acute‐care setting | 71% | 16 |
3. Percentage of asthma exacerbation discharges <9 years old | 73% | 18 |
4. Percentage of caregivers who respond to reminder‐recall systems for outpatient vaccination | 30% | 17 |
Cost‐effectiveness analysis | ||
5. Prevalence of influenza in school‐age children over 1 season | 45% | 1 |
6. Vaccine efficacy | 15 | |
Best case | 43% | |
Base case | 56% | |
Worst case | 75% | |
7. Risk of secondary transmission | 18% | 31, 32 |
8. Missed school days (per child per year) attributed to influenza | 0.79 | 29, 30 |
9. Percentage of caregivers who miss work to care for sick child | 53% | 6 |
Cost‐Effectiveness Analysis
The purpose of the cost‐effectiveness analysis was to evaluate potential cost savings attributable to improvement in vaccination delivery (UTD status). Direct and indirect costs were obtained by review of the current published literature pertaining to costs of influenza and influenza vaccination. The key assumptions for the cost‐effectiveness analysis are present in Table 1.
Vaccine Efficacy and Cost
Studies of influenza vaccine efficacy in those with asthma have had widely variable results on clinical outcomes.1923 Therefore, for the purpose of these analyses, we used a best‐case, worst‐case, base‐case vaccine efficacy approach as previously described.15 The vaccine efficacy base case was estimated as 56%, best case as 75%, and worst case as 43%. The primary cost‐effectiveness analysis was performed with the base‐case efficacy. Sensitivity analysis was performed by varying the vaccine efficacy between worst‐case efficacy and best‐case efficacy in order to determine effects on clinical outcomes and cost savings.
We determined the cost associated with vaccination assessment and delivery. Cost of only assessing a patient for vaccination without delivery was calculated as $5.32 on the basis of the assumption that a registered nurse (RN) would print a form (2 minutes), ask screening questions and wait for replies (5 minutes), and fax the form/order to the pharmacy and/or place it in the medical record (1 minute) at an RN salary of $40/hour. Assessment and administration of the vaccination was assumed to cost $18.84. This was based on additional nursing time of 10 minutes (1 minute to check arrival of the vaccination, 2 minutes to prepare it, 5 minutes to inject and dispose of it, and 1 minute to record delivery of the vaccination in the medical record) plus the cost of the vaccine (estimated as $6.75)24 and related supplies (estimated as $0.67 for the safety syringe, $0.01 for cotton balls, $0.01 for alcohol preps, and $0.065 for rubber gloves).25 The cost of vaccine and supplies was determined by reviewing pharmaceutical reference books and medical equipment billing Web sites. The indirect cost of delivery of the second vaccination in the outpatient setting to patients requiring 2 shots to become UTD was 3 hours of missed work per caregiver.
Influenza Prevalence and Morbidity
The prevalence of influenza in school‐age children is estimated at 40%54% among those not at high risk during influenza season.1 For this analysis we used 45%. Morbidity associated with influenza has been estimated as 120200 clinic visits and 65140 antibiotic prescriptions per 1000 children per year.9 This analysis used 150 clinic visits and 100 antibiotic prescriptions per 1000 children. The rate of hospitalization for influenza of high‐risk children per 100,000 is 1900 for those aged 0‐11 months, 800 for those aged 12 years, 320 for those aged 34 years, and 92 for those aged 514 years.8, 11 The risk of hospitalization for the model's hypothetical cohort was age‐adjusted using the age distribution found in the HCUP KID for children discharged with a primary diagnosis of asthma. The age distribution of children in the HCUP KID was 34% were 12 years, 15% were 34 years, and 51% were 514 years.18 Children aged 011 months were excluded because of the low likelihood that this age group was at risk for influenza complicated by asthma. The age‐adjusted risk for the hypothetical cohort in this analysis was 360 hospitalizations/100,000 children.
To investigate the effect of year‐to‐year variation in influenza prevalence and morbidity, the lowest and then highest estimates for these measures from previous studies were used in the model to determine the effect on the outcomes of cost per vaccination and cost per assessment. During this analysis all the measures were simultaneously entered at their lowest estimates to simulate a less active influenza season and then at their highest estimates to simulate a more active influenza season. The lowest and highest estimates of these assumptions were: influenza prevalence, 31% and 54%3; number of clinic visits, 120 to 200 per 1000 children8; and number of antibiotic prescriptions, 65 to 140 per 1000 children.8 No range was found in the literature for risk of hospitalization. Therefore, the standard 25% decrease and 25% increase were used as the lowest and highest estimates. The results of this analysis demonstrated the range of cost savings per year based on the prevalence and morbidity of influenza.
Direct and Indirect Cost of Influenza
Cost data were collected from multiple studies and inflated to 2006 dollars using the Bureau of Labor Statistics consumer price index for medical care and medical care services.26 The costs of a clinic visit and antibiotic prescription were assumed to be $5127 and $9.91,28 which were inflated to 2006 dollars: $70.09 and $13.76, respectively. Used as the mean cost for hospitalization in this analysis ($15,2695) was the more conservative estimate from 2 recent articles on determining the cost of hospitalization of high‐risk pediatric patients with influenza‐related illness.4, 5 Number of school days missed by children attributed to influenza has been previously established as 0.79 days per child per year.29, 30 The cost of a caregiver missing work to care for a child was assumed to be the median hourly wage of a child care provider according to the Bureau of Labor and Statistics 2005, which was $9.47/hour, or $9.94/hour in 2006 dollars. The model assumed that there was a 53% chance that a caregiver would need to miss work for a sick child.6 In addition, there was a risk of secondary transmission of 18%31, 32 to an average of 1.72 adults (percentage of 2‐parent households = 72%15). The cost of an adult secondary infection was $65.25, inflated to $99.49 in 2006 dollars. The estimate for the cost of a secondary infection was derived from a article by Nichol et al., who investigated the cost of influenza for healthy working adults.33 The total savings per vaccination of a healthy working adult was estimated as $46.85. This estimate included $10, the cost of the vaccination; $0.69, the cost of the side effects of the vaccine; and $7.71, the cost of missing work in order to receive the vaccination. A secondary case of influenza would be prevented without incurring these additional costs of vaccination, and therefore $65.25 (inflated to $99.49) was used in this analysis.
RESULTS
With existing data showing that only 29% of those with asthma are UTD on influenza vaccine in a given year, our decision analysis demonstrated that even modest increases in the rate of screening for influenza vaccine status among hospitalized patients with asthma can result in clinically significant increases in the rate of vaccine delivery. For example, screening of just 20% of hospitalized patients with asthma would result in 35% of the children ultimately being up to date for that influenza season, a 20% overall improvement. In the same manner, a 40% assessment rate would result in 41% of children ultimately becoming UTD, a 60% assessment rate in 47% ultimately becoming UTD, an 80% assessment rate in 53% ultimately becoming UTD, and a 100% assessment in 59% ultimately becoming UTD, which is double the baseline rate (Table 2 ).
Percent assessment | Vaccinations delivered (per 1000 patients) | Postintervention UTD |
---|---|---|
| ||
No increased assessment | None inpatient | 29% |
10% | 59 | 32% |
20% | 119 | 35% |
30% | 178 | 38% |
40% | 237 | 41% |
50% | 252 | 44% |
60% | 356 | 47% |
70% | 415 | 50% |
80% | 475 | 53% |
90% | 534 | 56% |
100% | 593 | 59% |
Direct cost of influenza | Indirect cost of influenza | Cost of inpatient vaccination | Total | Savings | |
---|---|---|---|---|---|
No increased assessment | $56.48 | $39.51 | $ 0 | $95.99 | Base |
Intervention to increase assessment | $45.24 | $31.64 | $13.66 | $90.54 | $5.45 |
This cost analysis demonstrated cost savings of $5.45 per child assessed and $9.19 per child vaccinated (Table 3). The cost savings per child assessed was lower than per child vaccinated because of including the costs of assessment without delivery of vaccination. This estimated savings is lower than the previously published $34.79 per vaccination of school‐age children in a group setting but more comparable to the cost savings of $3.99 in an individual‐initiated setting.15
The cost savings with this intervention depends on the vaccine's efficacy, and efficacy can vary as a function of whether it is an epidemic season and the degree of matching between the prevalent influenza strains and the vaccine strains. At higher predicted levels of vaccination efficacy, UTD patients have improved, less negative outcomes from influenza, and the intervention results in higher cost savings (cost savings with base‐case vaccine efficacy of $5.45/child assessed increases to $11.93/child assessed with best‐case vaccine efficacy). No current research accurately predicts vaccine efficacy for each outcome in pediatric patients with asthma. Therefore, this study design varied vaccine efficacy during sensitivity analyses among 3 potential vaccine efficacy scenarios (best‐, base‐, and worst‐case scenarios).15 Varying the vaccine efficacy to the best‐case scenario resulted in a cost savings of $11.93 per child assessed and $20.13 per child vaccinated. Dropping the efficacy to the worst‐case scenario resulted in cost savings of $1.01 per child assessed and $1.71 per child vaccinated. If the vaccine efficacy was 40%, the intervention was cost neutral. It should be remembered that the goal of the health care system is to generate good health. A project that is cost neutral (cost of vaccination intervention = cost savings from illness prevention), such as this intervention with a vaccine efficacy of 40%, may still be considered cost effective because of the improvement in clinical outcomes. At a vaccine efficacy of 40%, this model predicts that clinic visits will decrease by 19/1000 children, unwarranted antibiotics will decrease by 12/1000 children, hospitalizations will decrease by 4/10,000 children, and secondary infections of adult caregivers will decrease by 17/1000 children, suggesting an overall benefit to the health care system and to patients.
The clinical improvement predicted by this model with base‐case vaccine efficacy and 100% assessment include: a decrease in clinic visits of 27/1000 children, decrease in antibiotic use of 75/1000 children, decrease in hospitalizations of 6/10,000 children, decrease in missed school days of 132/1000 children, and a decrease in secondary infections of adult caregivers of 23/1000 children assessed.
The total cost of vaccination in this intervention was estimated as $10,593 per 1000 children. A hospital that discharges fewer than 1000 children with asthma per year would accrue less direct cost yearly with this intervention ($1059 per 100 children). A portion of this cost could be recuperated through reimbursement for influenza vaccination via insurance payers and/or government programs to reimburse for childhood vaccinations.
The results from the investigation into the effect of year‐to‐year variation in influenza prevalence and morbidity (by using the lowest and highest estimates found in the literature) demonstrated the model to be sensitive to these estimates, but the intervention maintained a cost savings. In a year with low influenza prevalence and morbidity, the cost savings decreases to $1.89 per child assessed and $3.20 per child vaccinated. In a year with high influenza prevalence and morbidity, the cost savings would increase to $8.75 per child assessed and $14.77 per child vaccinated. This finding of cost savings even with low influenza prevalence and morbidity is consistent with previous studies of influenza vaccination of pediatric patients in group‐based settings.15
For all other estimates, traditional sensitivity analysis was performed, and the results continued to show cost savings during this procedure, suggesting that the conclusions based on this model are generalizable and robust.
DISCUSSION
Universal screening for influenza vaccine status and then delivery of the vaccine to those not UTD among hospitalized children with asthma has the potential to increase the percentage of these children receiving the influenza vaccine and to reduce costs. This model suggests that with 100% assessment of this difficult‐to‐reach population for being UTD with the influenza vaccine, it would be possible to achieve a vaccination rate of 59%, doubling the current yearly receipt of influenza vaccination of children admitted secondary to asthma. However, universal screening is not imperative to achieve significant clinical improvement and cost savings. The cost‐effectiveness analysis demonstrated that the cost savings would be $5.45 per child assessed and $9.19 per child vaccinated.
The cost savings in this analysis ($9.19/child vaccinated) is favorable but lower than a previous analysis of influenza vaccination of children in an unspecified group‐based setting ($34.79/child vaccinated).15 Multiple factors contributed to our lower cost savings. First, our model accounts for the cost of children becoming partially vaccinated, incurring the full cost for 1 vaccination without the resulting clinical benefit. Second, the model accommodates for nursing assessment without vaccination delivery. Most importantly, this model estimates direct cost of vaccination delivery more conservatively than other group‐based estimates ($18.84 vs. $4.31).15 Previous cost‐effective analyses of influenza vaccination referenced 2 articles from the mid‐1990s that used a direct cost for 1 vaccination of $4 for group‐based vaccination in an HMO34 and $10 for individual‐initiated vaccination.33 Our estimate of vaccine cost is more conservative than these studies but more likely to represent the cost of vaccination assessment and delivery in an acute‐care hospital setting.
As the model was created, it became apparent that vaccination in the inpatient setting would accrue less indirect costs as compared with an individual‐initiated outpatient setting. This is a result of there not being any incrementally increased loss of work by the family/caregiver in order to be vaccinated above that lost because of hospitalization of the child for asthma. This finding supports that there is cost savings by vaccinating pediatric patients while hospitalized and is consistent with previous literature on this subject.15
The use of modeling techniques to evaluate inpatient interventions has many benefits. Modeling techniques permit the generation of synthetic trials by utilizing the combined published data from multiple studies. This permits the investigator to apply findings to other populations with different risks or prognoses and to other settings, to extend the impact over time, and to display multiple outcomes together. This technique has the potential to be used in investigations of the theoretical benefit of a specific quality improvement intervention in an inpatient setting without the extensive cost of a clinical trial. In fact, preliminary analysis with modeling could improve the cost efficiency of quality improvement research. Modeling studies could demonstrate which interventions are most likely to generate cost savings and direct clinical investigation and funding.
Limitations of this project included the possibility of vaccine efficacy changing from year to year depending on the similarity of the viruses in the vaccine with the predominant infectious strains circulating that year, as observed in previous studies.11 In a year with a poor match, the cost savings would be reduced compared with that in years with a good match. In addition, influenza prevalence and morbidity affects this model and the cost savings of the intervention, but the analysis using the lowest estimates of prevalence and morbidity continued to demonstrate that the intervention was saving costs. This continued cost savings for a group‐based vaccination intervention during years when there is low prevalence and morbidity is consistent with previous reports in the literature for pediatric patients.15 Modeling techniques decrease the effect of year‐to‐year variation in vaccination match, prevalence, and morbidity and estimate results based on an average over multiple influenza seasons.
For children with asthma, the data are not well established about influenza vaccination's ability to improve specific clinical outcomes attributed to influenza illness (missed school days, clinic visits, antibiotic prescriptions, hospitalizations, and secondary infections). Acknowledging the limitations of these data, our model estimates the intervention's improvement in clinical outcomes by using published data on vaccine efficacy and varying the vaccine efficacy from worst case to base case to best case. In addition, with a vaccine efficacy of 40%, this intervention would be cost neutral. Regardless of concerns about vaccine efficacy, the CDC/ACIP recommends finding missed opportunities for vaccination to improve vaccination delivery and the ultimate UTD status of high‐risk children. Recently, the CDC/ACIP stated that influenza vaccination coverage among children with asthma is inadequate and that opportunities for vaccination during health‐care provider visits likely are being missed.12 This intervention to assess and deliver influenza vaccination to pediatric patients while hospitalized for an asthma exacerbation would allow all hospitals that treat children to participate in reaching the goal of the CDC/ACIP to reduce missed opportunities for influenza vaccination.
The direct cost of inpatient vaccination in this intervention would fall primarily on the hospital implementing the intervention ($10,593 per 1000 children). However, the Vaccines for Children program exists to offset the cost of immunizations to both patients and providers for the uninsured and children receiving Medicaid.11 If properly implemented by participating hospitals, this program would improve reimbursement for vaccination and therefore shift the cost away from the individual hospital. For example, if the intervention cost borne by a hospital were decreased by the direct cost of vaccination alone ($6.75), this model predicts the cost of a program per 1000 children would decrease from $10,593 to $7190. This cost reduction would depend on the percentage of patients uninsured or on Medicaid at a given hospital. Another approach to shifting cost from individual hospitals would be for policy makers to consider influenza vaccination of these high‐risk pediatric patients as a performance/quality measure and associate the measure with improved reimbursement to hospitals in order to compensate for the cost of the vaccination intervention.
CONCLUSIONS
Influenza immunization is an accepted method of prevention and is underutilized in children with asthma.12 This model suggests that an inpatient program to deliver influenza vaccination to hospitalized pediatric patients with asthma would be beneficial by producing better health outcomes and reducing health care costs. Further research should be performed to verify the assumptions used in this analysis for children with asthma.
Acknowledgements
The authors acknowledge all the individuals who participated in the Academic Generalist Fellowship in Health Services Research at the Medical University of South Carolina for their direct and indirect participation in the study.
- Longitudinal studies of types a and b influenza among Seattle schoolchildren and families.J Infect Dis.1976;134:362–369. , , .
- Influenza virus infections in infants.Pediatr Infect Dis J.1997;16:1065–1068. , , , , .
- Economic impact of influenza vaccination in preschool children.Pediatrics.2000;106:973–976. , .
- Cost of influenza hospitalization at a tertiary care children's hospital and its impact on the cost‐benefit analysis of the recommendation for universal influenza immunization in children age 6 to 23 months.J Pediatr.2005;147:807–811. , .
- Direct medical cost of influenza‐related hospitalizations in children.Pediatrics.2006;118:e1321–e1327. , , , , .
- School absenteeism, parental work loss, and acceptance of childhood influenza vaccination.Am J Med Sci.2001;321:178–180. , , , .
- Illness among schoolchildren during influenza season: effect on school absenteeism, parental absenteeism from work, and secondary illness in families.Arch Pediatr Adolesc Med.2002;156:986–991. , , .
- The effect of influenza on hospitalizations, outpatient visits, and courses of antibiotics in children.N Engl J Med.2000;342:225–231. , , , , .
- The burden of influenza illness in children with asthma and other chronic medical conditions.J Pediatr.2000;137:856–864. , , , .
- The underrecognized burden of influenza in young children.N Engl J Med.2006;355(1):31–40. , , , et al.
- Prevention and control of influenza: recommendations of the Advisory Committee on Immunization Practices (ACIP).MMWR Recomm Rep.2006;55(RR‐10):1–42. , , , , , .
- Influenza vaccination coverage among children with asthma—United States, 2004–05 influenza season.MMWR Weekly.2007;56(9):193–196. , , , .
- Effect of prednisone on response to influenza virus vaccine in asthmatic children.Arch Pediatr Adolesc Med.1998;152:1191–1195. , , , , .
- Influenza vaccination of children during acute asthma exacerbation and concurrent prednisone therapy.Pediatrics.1996;98(2 Pt 1):196–200. , , , , .
- Potential cost savings attributable to influenza vaccination of school‐aged children.Pediatrics.1999;103:e73. , , .
- Providing immunizations in a pediatric emergency department: underimmunization rates and parental acceptance.Pediatr Emerg Care.1999;15:255–259. .
- Improving influenza vaccination rates in children with asthma: a test of a computerized reminder system and an analysis of factors predicting vaccination compliance.Pediatrics.1992;90:871–875. , , , .
- HealthCare Cost and Utilization Project: Kids' Inpatient Database (KID)2000. Agency for Healthcare Research and Quality.
- Influenza vaccination in patients with asthma: effect on the frequency of upper respiratory tract infections and exacerbations.J Asthma.2004;41:279–283. , , , , .
- Clinical effectiveness of conventional influenza vaccination in asthmatic children.Epidemiol Infect.2002;128:205–211. , , , , , .
- Influenza vaccination in asthmatic children: effects on quality of life and symptoms.Eur Respir J.2004;24:925–931. , , , et al.
- Influenza vaccination in children with asthma: randomized double‐blind placebo‐controlled trial.Am J Respir Crit Care Med.2004;169:488–493. , , , et al.
- Does influenza vaccination prevent asthma exacerbations in children?J Pediatr.2001;138:306–310. , , , et al.
- Red Book: Pharmacy's Fundamental Reference.2006 ed: Thomson Healthcare2006.
- Flu Vaccine Pre‐Book 2007‐08. Dubin Medical Incorporation. Available at: http://www.dubinmedical.com/news/index.htm. Accessed June 21,2007.
- Bureau of Labor Statistics Data. U.S. Department of Labor: Bureau of Labor Statistics; 2006. Available at: http://www.bls.gov. Accessed May 18,2007.
- Association Medical Association.Socioeconomics of medical practice, 1997.Am Med Assoc.1997;69(89):107.
- The Cost of antibiotics in treating respiratory tract infections in a Medicaid population.Arch Fam Med.1998;7:45–49. , .
- Comparison of US inactivated split‐virus and Russian attenuated, cold‐adapted trivalent influenza vaccines in Russian schoolchildren.JInfect Dis.1996;173:453–456. , , .
- Current estimates from the National Health Interview Survey, 1994. National Health Statistics.Vital Health Stat.1995;10:193. , .
- Influenza B virus infections in the community and the family. The epidemics of 1976‐1977 and 1979‐1980 in Houston, Texas.Am J Epidemiol.1983;118:313–325. , , , , , .
- Emergence and apparent transmission of rimantidine‐resistant influenza a virus in families.N Engl J Med.1989;321:1696–1702. , , , , , .
- The effectiveness ofvaccination against influenza in healthy, working adults.N Engl J Med.1995;333:889–893. , , , et al.
- The efficacy and cost effectiveness of vaccination against influenza among elderly persons living in the community.N Engl J Med.1994;331:778–784. , , , .
- Longitudinal studies of types a and b influenza among Seattle schoolchildren and families.J Infect Dis.1976;134:362–369. , , .
- Influenza virus infections in infants.Pediatr Infect Dis J.1997;16:1065–1068. , , , , .
- Economic impact of influenza vaccination in preschool children.Pediatrics.2000;106:973–976. , .
- Cost of influenza hospitalization at a tertiary care children's hospital and its impact on the cost‐benefit analysis of the recommendation for universal influenza immunization in children age 6 to 23 months.J Pediatr.2005;147:807–811. , .
- Direct medical cost of influenza‐related hospitalizations in children.Pediatrics.2006;118:e1321–e1327. , , , , .
- School absenteeism, parental work loss, and acceptance of childhood influenza vaccination.Am J Med Sci.2001;321:178–180. , , , .
- Illness among schoolchildren during influenza season: effect on school absenteeism, parental absenteeism from work, and secondary illness in families.Arch Pediatr Adolesc Med.2002;156:986–991. , , .
- The effect of influenza on hospitalizations, outpatient visits, and courses of antibiotics in children.N Engl J Med.2000;342:225–231. , , , , .
- The burden of influenza illness in children with asthma and other chronic medical conditions.J Pediatr.2000;137:856–864. , , , .
- The underrecognized burden of influenza in young children.N Engl J Med.2006;355(1):31–40. , , , et al.
- Prevention and control of influenza: recommendations of the Advisory Committee on Immunization Practices (ACIP).MMWR Recomm Rep.2006;55(RR‐10):1–42. , , , , , .
- Influenza vaccination coverage among children with asthma—United States, 2004–05 influenza season.MMWR Weekly.2007;56(9):193–196. , , , .
- Effect of prednisone on response to influenza virus vaccine in asthmatic children.Arch Pediatr Adolesc Med.1998;152:1191–1195. , , , , .
- Influenza vaccination of children during acute asthma exacerbation and concurrent prednisone therapy.Pediatrics.1996;98(2 Pt 1):196–200. , , , , .
- Potential cost savings attributable to influenza vaccination of school‐aged children.Pediatrics.1999;103:e73. , , .
- Providing immunizations in a pediatric emergency department: underimmunization rates and parental acceptance.Pediatr Emerg Care.1999;15:255–259. .
- Improving influenza vaccination rates in children with asthma: a test of a computerized reminder system and an analysis of factors predicting vaccination compliance.Pediatrics.1992;90:871–875. , , , .
- HealthCare Cost and Utilization Project: Kids' Inpatient Database (KID)2000. Agency for Healthcare Research and Quality.
- Influenza vaccination in patients with asthma: effect on the frequency of upper respiratory tract infections and exacerbations.J Asthma.2004;41:279–283. , , , , .
- Clinical effectiveness of conventional influenza vaccination in asthmatic children.Epidemiol Infect.2002;128:205–211. , , , , , .
- Influenza vaccination in asthmatic children: effects on quality of life and symptoms.Eur Respir J.2004;24:925–931. , , , et al.
- Influenza vaccination in children with asthma: randomized double‐blind placebo‐controlled trial.Am J Respir Crit Care Med.2004;169:488–493. , , , et al.
- Does influenza vaccination prevent asthma exacerbations in children?J Pediatr.2001;138:306–310. , , , et al.
- Red Book: Pharmacy's Fundamental Reference.2006 ed: Thomson Healthcare2006.
- Flu Vaccine Pre‐Book 2007‐08. Dubin Medical Incorporation. Available at: http://www.dubinmedical.com/news/index.htm. Accessed June 21,2007.
- Bureau of Labor Statistics Data. U.S. Department of Labor: Bureau of Labor Statistics; 2006. Available at: http://www.bls.gov. Accessed May 18,2007.
- Association Medical Association.Socioeconomics of medical practice, 1997.Am Med Assoc.1997;69(89):107.
- The Cost of antibiotics in treating respiratory tract infections in a Medicaid population.Arch Fam Med.1998;7:45–49. , .
- Comparison of US inactivated split‐virus and Russian attenuated, cold‐adapted trivalent influenza vaccines in Russian schoolchildren.JInfect Dis.1996;173:453–456. , , .
- Current estimates from the National Health Interview Survey, 1994. National Health Statistics.Vital Health Stat.1995;10:193. , .
- Influenza B virus infections in the community and the family. The epidemics of 1976‐1977 and 1979‐1980 in Houston, Texas.Am J Epidemiol.1983;118:313–325. , , , , , .
- Emergence and apparent transmission of rimantidine‐resistant influenza a virus in families.N Engl J Med.1989;321:1696–1702. , , , , , .
- The effectiveness ofvaccination against influenza in healthy, working adults.N Engl J Med.1995;333:889–893. , , , et al.
- The efficacy and cost effectiveness of vaccination against influenza among elderly persons living in the community.N Engl J Med.1994;331:778–784. , , , .
Copyright © 2008 Society of Hospital Medicine
Diagnosis of Exclusion
A 26‐year‐old woman was brought to the emergency department following several episodes of seizures. The patient's friend witnessed several 15‐minute episodes of sudden jerks and tremors of her right arm during which the patient bit her tongue, had word‐finding difficulty, had horizontal eye deviation, and was incontinent of urine. She became unresponsive during the episodes, with incomplete recovery of consciousness between attacks. She was afebrile. Her neurologic exam 4 hours after several seizures revealed word‐finding difficulty and right arm weakness. A complete blood count, chemistry panel including renal and liver function tests, urine toxicology screen, and computed tomography (CT) of the head were normal. After a loading dose of fosphenytoin, the patient did not experience further seizures and was discharged on a maintenance dose of phenytoin.
Over the next week, the patient continued to note a sensation of heaviness in her right arm and felt fatigued. The patient's mother brought her back to the emergency department after witnessing a similar seizure episode that persisted for an hour. On arrival, the patient was no longer seizing.
Although it can sometimes be difficult to differentiate between seizure, stroke, syncope, and other causes of transient loss of consciousness, this constellation of symptoms strongly points to a seizure. I would classify the patient's focal arm movements associated with impaired consciousness as partial complex seizures. One of the first considerations is determining whether the seizure is caused by a systemic process or by an intrinsic central nervous system disorder. Common systemic illnesses include infections, metabolic disturbances, toxins, and malignancies, none of which are evident on the preliminary evaluation. The absence of fever is important as is the time frame (now extending over 1 week) in excluding acute bacterial meningitis. A negative urine toxicology is very helpful but does not exclude the possibility that the seizure is from unmeasured drug intoxication, for example, tricyclic antidepressants, or from drug withdrawal, for example, benzodiazepines, barbiturates, ethanol, and antiepileptic drugs. The persistent right arm heaviness and right arm jerking during the seizures suggest a left cortical focus that the CT scan did not detect. Without a clear diagnosis and with recurrent seizures despite antiepileptic drugs, hospitalization is warranted.
The patient experienced migraine headaches each month during menses. There was no family history of seizures. Her only medication was phenytoin. The mother was unaware of any use of tobacco, alcohol, or recreational drugs. The patient was raised in New Jersey and moved to the San Francisco Bay area 9 months ago. She had no pets and had traveled to Florida and Montreal in the past 6 months. She was a graduate student in performing arts. During the preceding 2 weeks she had been under significant stress and had not slept much in preparation for an upcoming production. The patient's mother was not aware of any head trauma, recent illness, fevers, chills, weight loss, photosensitivity, arthralgias, nausea, vomiting, or diarrhea.
Recent sleep deprivation could provoke seizures in a patient with a latent anatomic focus or metabolic predisposition. Nonadherence to antiepileptic drug therapy is the most common reason for patients to present to the ED with seizures; therefore, I would check a phenytoin level to assess whether she is at a therapeutic level and would consider administering another loading dose. In the absence of immunocompromise or unusual activities or exposures, North American travel does not bring to mind additional etiologies at this time.
On exam, temperature was 37.3C, blood pressure was 148/84 mm Hg, heart rate was 120 per minute, and respiratory rate was 16 per minute. The patient was stuporous and withdrew from painful stimuli. She was unable to speak. Pupils were 4 mm in diameter and reacted to light. No gaze preference or nystagmus was present. There was no meningismus. Deep tendon reflexes were 1+ and symmetrical in both upper and lower extremities. Plantar reflexes were extensor bilaterally. The tone in the right upper extremity was mildly increased compared to the left. The patient demonstrated semipurposeful movement of the limbs, such as reaching for the bed rails with her arms. Examination of the heart, lungs, abdomen, skin, and oropharynx was normal.
The white blood cell count was 22,300/mm3 with 50% neutrophils, 40% lymphocytes, 7% monocytes, and 3% eosinophils. Results of the chemistry panel including electrolytes, glucose, creatinine, and liver enzymes, urinalysis, and thyroid‐stimulating hormone were normal. Serum phenytoin level was 8.1 g/mL. Urine toxicology screen, obtained after the patient had received lorazepam, was positive only for benzodiazepines. A chest radiograph was normal.
The cerebrospinal fluid (CSF) was colorless, containing 35 white blood cells/mm3 (48% lymphocytes, 30% neutrophils, 22% monocytes), 3 red blood cells/mm3, 62 mg/dL protein, and 50 mg/dL glucose. There was no xanthochromia. The CSF was negative for cryptococcal antigen, antibodies to West Nile virus, PCR for herpes simplex viruses‐1 and ‐2, and PCR for Borrelia burgdorferi. CSF bacterial culture, cryptococcal antigen, and AFB stain were negative. The serum antinuclear antibody, rheumatoid factor, and rapid plasma reagin were negative. Serum antibodies to human immunodeficiency virus, hepatitis B and C viruses, Borrelia burgdorferi, and herpes simplex viruses were negative. The erythrocyte sedimentation rate was 25 mm/hr. There was no growth in her blood cultures.
These CSF findings have to be interpreted in light of her clinical picture, as they are congruent with both an aseptic meningitis and encephalitis. In practice, these can be hard to distinguish, but the early and dominant cortical findings (focal neurologic deficits, prominent altered mental status, bilateral extensor plantar reflexes) and absence of meningeal signs favor encephalitis. This CSF profile can be seen in a variety of disease processes causing a meningoencephalitis, including partially treated bacterial meningitis; meningitis due to viruses, fungi, mycobacteria, or atypical bacteria (eg, Listeria); neurosarcoidosis; carcinomatous meningitis; and infection or inflammation from a parameningeal focus in the sinuses, epidural space, or brain parenchyma. Seizure itself can lead to a postictal pleocytosis in the CSF, although this degree of inflammation would be unusual. Many tests can be sent, and the clinicians appropriately focused on some of the most treatable and serious etiologies first. The negative HIV test limits the list of opportunistic pathogens. The negative ANA substantially lowers the likelihood of systemic lupus, an important consideration in a young woman with an inflammatory disorder involving the central nervous system.
Magnetic resonance imaging (MRI) of the brain showed cortical T2 prolongation with significant enhancement with gadolinium in the cortex and leptomeninges of the left parietal and posterotemporal lobes and right cingulate gyrus region (Fig. 1). The patient was admitted to the intensive care unit, and phenytoin and levetiracetam were administered. Over the next several days, she remained afebrile, and her leukocytosis resolved. She continued to have seizures every day despite receiving phenytoin, levetiracetam, and lamotrigine. She was alert and complained about persistent right arm weakness and word‐finding difficulties. Posterior cervical lymphadenopathy at the base of her left occiput was detected on subsequent exam.
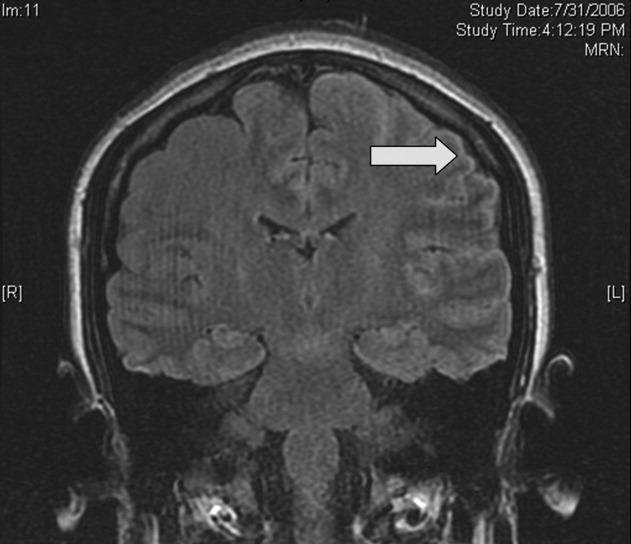
An excisional lymph node biopsy demonstrated extensive necrosis without evidence of granulomata, malignancy, or lymphoproliferative disease. Stains and cultures for bacteria, fungi, and mycobacteria were negative. The patient's electroencephalogram captured epileptiform activity over the left hemisphere 2 hours after a cluster of seizures. MR angiography and cerebral angiography demonstrated no abnormalities.
Despite this additional information, there is no distinguishing clue that points to a single diagnosis. This is a 26‐year‐old healthy, seemingly immunocompetent woman who has had a 2‐week progressive and refractory seizure disorder secondary to a multifocal neuroinvasive process with a CSF pleocytosis. She does not have evidence of a systemic underlying disorder, save for nonspecific localized lymphadenopathy and a transient episode of leukocytosis on admission, and has no distinguishing epidemiological factors or exposures.
Despite my initial concerns for infectious meningoencephalitis, the negative stains, serologies, and cultures of the blood, CSF, and lymph nodes in the setting of a normal immune system and no suspect exposure substantially lower this probability. Arthropod‐borne viruses are still possible, especially West Nile virus, because the serological tests are less sensitive early in the illness, acknowledging that the absence of fever, weakness, and known mosquito bites detracts from this diagnosis. Pathogens that cause regional lymphadenopathy and encephalitis such as Bartonella remain possibilities, as the history of exposure to a kitten can be easily overlooked.
Rheumatologic disorders merit close attention in a young woman, but the negative ANA makes lupus cerebritis unlikely, and the 2 angiograms did not detect evidence of vasculitis. Finally, there is the question of malignancy and other miscellaneous infiltrative disorders (such as sarcoid), which are of importance here because of the multifocal cortical involvement on imaging.
At this point, I would resample the CSF for viral etiologies (eg, West Nile virus) and cytology and would send serum Bartonella serologies. If these studies were negative, a brain biopsy, primarily to exclude malignancy but also to uncover an unsuspected process, would be indicated. I cannot make a definitive diagnosis or find a perfect fit here, but in the absence of strong evidence of an infection, I am concerned about a malignancy, perhaps a low‐grade primary brain tumor.
Brain biopsy of the leptomeninges and cortex of the left parietal lobe showed multiple blood vessels infiltrated by lymphocytes, neutrophils, and eosinophils (Fig. 2). The pattern of inflammation was consistent with primary angiitis of the central nervous system (PACNS). The patient received 1 g of intravenous methylprednisolone on 3 consecutive days, followed by oral prednisone and cyclophosphamide. The seizures ceased, and she made steady progress with rehabilitation therapy. Four months after discharge a cerebral angiogram (done to ensure there was no interval evidence of vasculitis prior to tapering therapy) demonstrated patency of all major intracranial arteries and venous sinuses.
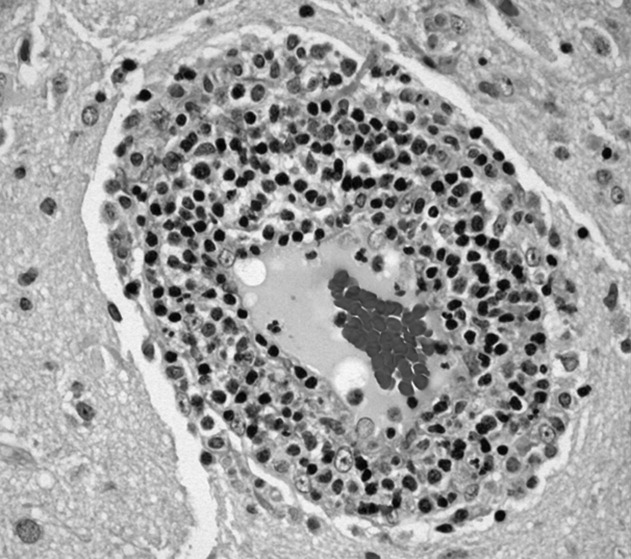
COMMENTARY
When a patient presents with symptoms or signs referable to the central nervous system (CNS), hospitalists must simultaneously consider primary neurologic disorders and systemic diseases that involve the CNS. Initial evaluation includes a thorough history and physical examination, basic lab studies, routine CSF analysis, and neuroimaging (often a CT scan of the head). Complicated neurologic cases may warrant more elaborate testing including EEG, brain MRI, cerebral angiography, and specialized blood and CSF studies. Clinicians may still find themselves faced with a patient who has clear CNS dysfunction but no obvious diagnosis despite an exhaustive and expensive evaluation. Several disorders match this profile including intravascular lymphoma, prion diseases, paraneoplastic syndromes, and cerebritis. Primary angiitis of the central nervous system (PACNS), a rare disorder characterized by inflammation of the medium‐sized and small arteries of the CNS, is among these disorders. Although the aforementioned diseases may sometimes have suggestive or even pathognomonic features (eg, the string of beads angiographic appearance in vasculitides), they are challenging to diagnose when such findings are absent.
Like any vasculitis of the CNS, PACNS may present with a wide spectrum of clinical features.12 Although headache and altered mental status are the most common complaints, paresis, seizures, ataxia, visual changes, and aphasia have all been described. The onset of symptoms ranges from acute to chronic, and neurologic deficits can be focal or diffuse. Systemic manifestations such as fever and weight loss are rare. The average age of onset is 42 years, with no significant sex preponderance. The histopathology of PACNS is granulomatous inflammation of arteries in the parenchyma and leptomeninges of the brain and less commonly in the spinal cord. The narrowing of the affected vessels causes cerebral ischemia and the associated neurologic deficits. The trigger for this focal inflammation is unknown.
After common disorders have been excluded in cases of CNS dysfunction, compatible CSF findings and imaging results may prompt consideration of PACNS. CSF analysis in patients with PACNS typically demonstrates a lymphocytic pleocytosis. MRI abnormalities in PACNS include multiple infarcts in the cortex, deep white matter, or leptomeninges.34 Less specific findings are contrast enhancement in the leptomeninges and white matter disease, both of which may direct the site for meningeal and brain biopsy.
Both brain MRA and cerebral angiography have a limited role in the diagnosis of vasculitis within the CNS. In 18 patients with CNS vasculitis due to autoimmune disease, all had parenchymal abnormalities on MRA but only 65% had evidence of vasculitis on angiography. In 2 retrospective studies of patients with suspected PACNS, abnormal angiograms had a specificity less than 30% for PACNS, whereas brain biopsies had a negative predictive value of 70%.57 Although in practice patients with compatible clinical features are sometimes diagnosed with CNS vasculitis on the basis of angiographic findings, brain biopsy is necessary to differentiate vasculitis from other vasculopathies and to establish a definitive diagnosis.
Before a diagnosis of PACNS is made, care must be taken to exclude infections, neoplasms, and autoimmune processes that cause angiitis of the CNS (Table 1). The presence of any extracranial abnormalities (which were not present in this case) should prompt consideration of an underlying systemic disorder causing a secondary CNS vasculitis and should cast doubt on the diagnosis of PACNS. Meningovascular syphilis and tuberculosis are among the long list of infections that may cause inflammation of the CNS vasculature. Autoimmune disorders that may cause vasculitis inside the brain include polyarteritis nodosa and Wegener's granulomatosis. Reversible cerebral vasoconstrictive disease, which is most commonly seen in women ages 20 to 50, and sympathomimetic toxins such as cocaine and amphetamine may exhibit clinical and angiographic abnormalities indistinguishable from PACNS.89
Infection: Viruses (HIV, varicella‐zoster virus, hepatitis C virus), syphilis, Borrelia burgdorferi, Bartonella, Mycobacterium tuberculosis, fungi (Aspergillus, Coccidioides), bacteria. |
Autoimmune: Polyarteritis nodosa, Wegener's granulomatosis, temporal arteritis, cryoglobulinemic vasculitis, lupus vasculitis, rheumatoid vasculitis. |
Toxins: Amphetamine, cocaine, ephedrine, heroin. |
Malignancy: Primary CNS lymphoma, angioimmunoproliferative disorders, infiltrating glioma. |
There are no prospective trials investigating PACNS treatment. Aggressive immunosuppression with cyclophosphamide and glucocorticoids is the mainstay of treatment. The duration of treatment varies with the severity of the disease and response to therapy. One study suggests that treatment should be continued for 6 to 12 months.10 Neurologic deficits may remain irreversible because of scarring of the affected vessels. Serial brain MRI examinations are often used to follow radiographic resolution during and after the therapy, although radiographic changes do not predict clinical response.11 New abnormalities on MRI, however, delay any tapering of treatment. The availability of neuroimaging studies and immunosuppressive therapy has improved the prognosis of PACNS. One study reported a favorable outcome with a 29% relapse rate and a 10% mortality rate in 54 patients over a mean follow‐up period of 35 months.12
PACNS remains a challenging diagnosis because of its rarity, the wide range of neurologic manifestations, and the difficulty in establishing a diagnosis noninvasively. It is an extremely uncommon disease but should be considered in patients with unexplained neurologic deficits referable to the CNS alone after an exhaustive workup. Ultimately, the diagnosis is made by a thorough history and physical examination, exclusion of underlying conditions (particularly systemic vasculitides and infections), and histological confirmation.
Key Points for Hospitalists
-
Serious disorders that may present with CNS abnormalities and nondiagnostic abnormal findings on lumbar puncture, brain MRI, and cerebral angiography include intravascular lymphoma, prion diseases, cerebritis, paraneoplastic syndromes, and CNS vasculitis.
-
PACNS is a challenging diagnosis with varied clinical features and often normal angiographic findings. In particular, the specificity of brain MRA and cerebral angiography is low. Although PACNS is rare, it should be on the differential diagnosis, as the condition is fatal without prompt treatment.
-
A diagnosis of PACNS is made only after excluding secondary causes of CNS vasculitis such as infections, malignancies, autoimmune conditions, reversible cerebral vasoconstrictive disease, and medications. The diagnosis is confirmed with a biopsy of the brain and meninges.
- Medical progress: small‐vessel vasculitis.N Engl J Med.1997;337:1512–1523. , .
- Arthritis and Allied Conditions: A Textbook of Rheumatology.15th ed.Philadelphia:Lippincott Williams 2005. , .
- Primary angiitis of the central nervous system: unusual MR appearance.Am J Neuroradiol.1994;15:331–334. , , , .
- Radiographic features of central nervous system vasculitis.Neurol Clin.1997;15:779–804. , , , .
- Primary angiitis of the central nervous system at conventional angiography.Radiology.2004;233:878–882. , , , , , .
- CNS vasculitis in autoimmune disease: MR imaging findings and correlation with angiography.Am J Neuroradiol.1999;20:75–85. , , , , .
- Limitations of invasive modalities in the diagnosis of primary angiitis of the central nervous system.J Rheumatol.1995;22:662–667. , .
- Amphetamine abuse and intracranial haemorrhage.J R Soc Med.2000;93:472–477. , .
- Drug‐induced vasculitis.Curr Opin Rheumatol.1996;8:34–40. , .
- Vasculitis in the central nervous system.Arthritis Rheum.1997;40:1189–1201. , , .
- Therapy of systemic vasculitis.Neurol Clin.1997;15:973–991. .
- Long‐term outcomes of patients with primary angiitis of the central nervous system.Arthritis Rheum.2000;43:S162. , , , et al.
A 26‐year‐old woman was brought to the emergency department following several episodes of seizures. The patient's friend witnessed several 15‐minute episodes of sudden jerks and tremors of her right arm during which the patient bit her tongue, had word‐finding difficulty, had horizontal eye deviation, and was incontinent of urine. She became unresponsive during the episodes, with incomplete recovery of consciousness between attacks. She was afebrile. Her neurologic exam 4 hours after several seizures revealed word‐finding difficulty and right arm weakness. A complete blood count, chemistry panel including renal and liver function tests, urine toxicology screen, and computed tomography (CT) of the head were normal. After a loading dose of fosphenytoin, the patient did not experience further seizures and was discharged on a maintenance dose of phenytoin.
Over the next week, the patient continued to note a sensation of heaviness in her right arm and felt fatigued. The patient's mother brought her back to the emergency department after witnessing a similar seizure episode that persisted for an hour. On arrival, the patient was no longer seizing.
Although it can sometimes be difficult to differentiate between seizure, stroke, syncope, and other causes of transient loss of consciousness, this constellation of symptoms strongly points to a seizure. I would classify the patient's focal arm movements associated with impaired consciousness as partial complex seizures. One of the first considerations is determining whether the seizure is caused by a systemic process or by an intrinsic central nervous system disorder. Common systemic illnesses include infections, metabolic disturbances, toxins, and malignancies, none of which are evident on the preliminary evaluation. The absence of fever is important as is the time frame (now extending over 1 week) in excluding acute bacterial meningitis. A negative urine toxicology is very helpful but does not exclude the possibility that the seizure is from unmeasured drug intoxication, for example, tricyclic antidepressants, or from drug withdrawal, for example, benzodiazepines, barbiturates, ethanol, and antiepileptic drugs. The persistent right arm heaviness and right arm jerking during the seizures suggest a left cortical focus that the CT scan did not detect. Without a clear diagnosis and with recurrent seizures despite antiepileptic drugs, hospitalization is warranted.
The patient experienced migraine headaches each month during menses. There was no family history of seizures. Her only medication was phenytoin. The mother was unaware of any use of tobacco, alcohol, or recreational drugs. The patient was raised in New Jersey and moved to the San Francisco Bay area 9 months ago. She had no pets and had traveled to Florida and Montreal in the past 6 months. She was a graduate student in performing arts. During the preceding 2 weeks she had been under significant stress and had not slept much in preparation for an upcoming production. The patient's mother was not aware of any head trauma, recent illness, fevers, chills, weight loss, photosensitivity, arthralgias, nausea, vomiting, or diarrhea.
Recent sleep deprivation could provoke seizures in a patient with a latent anatomic focus or metabolic predisposition. Nonadherence to antiepileptic drug therapy is the most common reason for patients to present to the ED with seizures; therefore, I would check a phenytoin level to assess whether she is at a therapeutic level and would consider administering another loading dose. In the absence of immunocompromise or unusual activities or exposures, North American travel does not bring to mind additional etiologies at this time.
On exam, temperature was 37.3C, blood pressure was 148/84 mm Hg, heart rate was 120 per minute, and respiratory rate was 16 per minute. The patient was stuporous and withdrew from painful stimuli. She was unable to speak. Pupils were 4 mm in diameter and reacted to light. No gaze preference or nystagmus was present. There was no meningismus. Deep tendon reflexes were 1+ and symmetrical in both upper and lower extremities. Plantar reflexes were extensor bilaterally. The tone in the right upper extremity was mildly increased compared to the left. The patient demonstrated semipurposeful movement of the limbs, such as reaching for the bed rails with her arms. Examination of the heart, lungs, abdomen, skin, and oropharynx was normal.
The white blood cell count was 22,300/mm3 with 50% neutrophils, 40% lymphocytes, 7% monocytes, and 3% eosinophils. Results of the chemistry panel including electrolytes, glucose, creatinine, and liver enzymes, urinalysis, and thyroid‐stimulating hormone were normal. Serum phenytoin level was 8.1 g/mL. Urine toxicology screen, obtained after the patient had received lorazepam, was positive only for benzodiazepines. A chest radiograph was normal.
The cerebrospinal fluid (CSF) was colorless, containing 35 white blood cells/mm3 (48% lymphocytes, 30% neutrophils, 22% monocytes), 3 red blood cells/mm3, 62 mg/dL protein, and 50 mg/dL glucose. There was no xanthochromia. The CSF was negative for cryptococcal antigen, antibodies to West Nile virus, PCR for herpes simplex viruses‐1 and ‐2, and PCR for Borrelia burgdorferi. CSF bacterial culture, cryptococcal antigen, and AFB stain were negative. The serum antinuclear antibody, rheumatoid factor, and rapid plasma reagin were negative. Serum antibodies to human immunodeficiency virus, hepatitis B and C viruses, Borrelia burgdorferi, and herpes simplex viruses were negative. The erythrocyte sedimentation rate was 25 mm/hr. There was no growth in her blood cultures.
These CSF findings have to be interpreted in light of her clinical picture, as they are congruent with both an aseptic meningitis and encephalitis. In practice, these can be hard to distinguish, but the early and dominant cortical findings (focal neurologic deficits, prominent altered mental status, bilateral extensor plantar reflexes) and absence of meningeal signs favor encephalitis. This CSF profile can be seen in a variety of disease processes causing a meningoencephalitis, including partially treated bacterial meningitis; meningitis due to viruses, fungi, mycobacteria, or atypical bacteria (eg, Listeria); neurosarcoidosis; carcinomatous meningitis; and infection or inflammation from a parameningeal focus in the sinuses, epidural space, or brain parenchyma. Seizure itself can lead to a postictal pleocytosis in the CSF, although this degree of inflammation would be unusual. Many tests can be sent, and the clinicians appropriately focused on some of the most treatable and serious etiologies first. The negative HIV test limits the list of opportunistic pathogens. The negative ANA substantially lowers the likelihood of systemic lupus, an important consideration in a young woman with an inflammatory disorder involving the central nervous system.
Magnetic resonance imaging (MRI) of the brain showed cortical T2 prolongation with significant enhancement with gadolinium in the cortex and leptomeninges of the left parietal and posterotemporal lobes and right cingulate gyrus region (Fig. 1). The patient was admitted to the intensive care unit, and phenytoin and levetiracetam were administered. Over the next several days, she remained afebrile, and her leukocytosis resolved. She continued to have seizures every day despite receiving phenytoin, levetiracetam, and lamotrigine. She was alert and complained about persistent right arm weakness and word‐finding difficulties. Posterior cervical lymphadenopathy at the base of her left occiput was detected on subsequent exam.
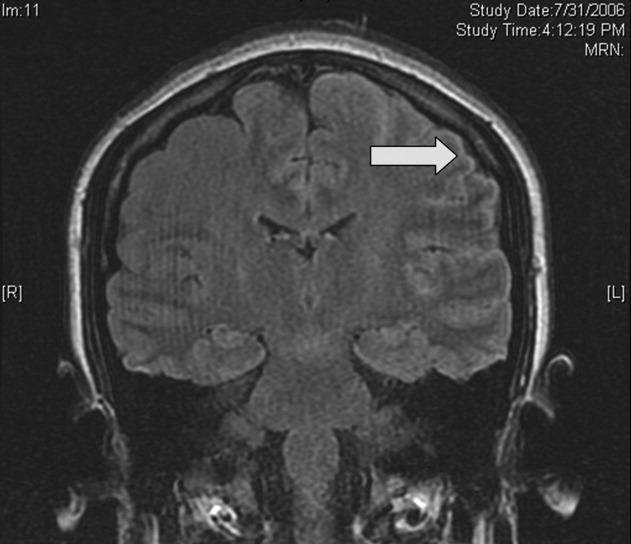
An excisional lymph node biopsy demonstrated extensive necrosis without evidence of granulomata, malignancy, or lymphoproliferative disease. Stains and cultures for bacteria, fungi, and mycobacteria were negative. The patient's electroencephalogram captured epileptiform activity over the left hemisphere 2 hours after a cluster of seizures. MR angiography and cerebral angiography demonstrated no abnormalities.
Despite this additional information, there is no distinguishing clue that points to a single diagnosis. This is a 26‐year‐old healthy, seemingly immunocompetent woman who has had a 2‐week progressive and refractory seizure disorder secondary to a multifocal neuroinvasive process with a CSF pleocytosis. She does not have evidence of a systemic underlying disorder, save for nonspecific localized lymphadenopathy and a transient episode of leukocytosis on admission, and has no distinguishing epidemiological factors or exposures.
Despite my initial concerns for infectious meningoencephalitis, the negative stains, serologies, and cultures of the blood, CSF, and lymph nodes in the setting of a normal immune system and no suspect exposure substantially lower this probability. Arthropod‐borne viruses are still possible, especially West Nile virus, because the serological tests are less sensitive early in the illness, acknowledging that the absence of fever, weakness, and known mosquito bites detracts from this diagnosis. Pathogens that cause regional lymphadenopathy and encephalitis such as Bartonella remain possibilities, as the history of exposure to a kitten can be easily overlooked.
Rheumatologic disorders merit close attention in a young woman, but the negative ANA makes lupus cerebritis unlikely, and the 2 angiograms did not detect evidence of vasculitis. Finally, there is the question of malignancy and other miscellaneous infiltrative disorders (such as sarcoid), which are of importance here because of the multifocal cortical involvement on imaging.
At this point, I would resample the CSF for viral etiologies (eg, West Nile virus) and cytology and would send serum Bartonella serologies. If these studies were negative, a brain biopsy, primarily to exclude malignancy but also to uncover an unsuspected process, would be indicated. I cannot make a definitive diagnosis or find a perfect fit here, but in the absence of strong evidence of an infection, I am concerned about a malignancy, perhaps a low‐grade primary brain tumor.
Brain biopsy of the leptomeninges and cortex of the left parietal lobe showed multiple blood vessels infiltrated by lymphocytes, neutrophils, and eosinophils (Fig. 2). The pattern of inflammation was consistent with primary angiitis of the central nervous system (PACNS). The patient received 1 g of intravenous methylprednisolone on 3 consecutive days, followed by oral prednisone and cyclophosphamide. The seizures ceased, and she made steady progress with rehabilitation therapy. Four months after discharge a cerebral angiogram (done to ensure there was no interval evidence of vasculitis prior to tapering therapy) demonstrated patency of all major intracranial arteries and venous sinuses.
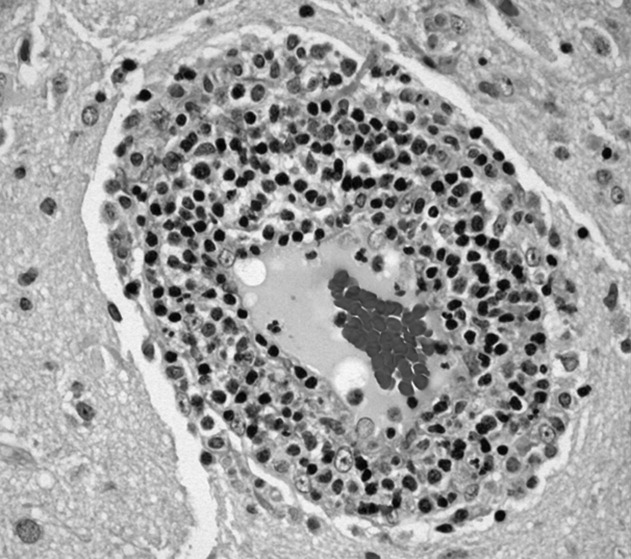
COMMENTARY
When a patient presents with symptoms or signs referable to the central nervous system (CNS), hospitalists must simultaneously consider primary neurologic disorders and systemic diseases that involve the CNS. Initial evaluation includes a thorough history and physical examination, basic lab studies, routine CSF analysis, and neuroimaging (often a CT scan of the head). Complicated neurologic cases may warrant more elaborate testing including EEG, brain MRI, cerebral angiography, and specialized blood and CSF studies. Clinicians may still find themselves faced with a patient who has clear CNS dysfunction but no obvious diagnosis despite an exhaustive and expensive evaluation. Several disorders match this profile including intravascular lymphoma, prion diseases, paraneoplastic syndromes, and cerebritis. Primary angiitis of the central nervous system (PACNS), a rare disorder characterized by inflammation of the medium‐sized and small arteries of the CNS, is among these disorders. Although the aforementioned diseases may sometimes have suggestive or even pathognomonic features (eg, the string of beads angiographic appearance in vasculitides), they are challenging to diagnose when such findings are absent.
Like any vasculitis of the CNS, PACNS may present with a wide spectrum of clinical features.12 Although headache and altered mental status are the most common complaints, paresis, seizures, ataxia, visual changes, and aphasia have all been described. The onset of symptoms ranges from acute to chronic, and neurologic deficits can be focal or diffuse. Systemic manifestations such as fever and weight loss are rare. The average age of onset is 42 years, with no significant sex preponderance. The histopathology of PACNS is granulomatous inflammation of arteries in the parenchyma and leptomeninges of the brain and less commonly in the spinal cord. The narrowing of the affected vessels causes cerebral ischemia and the associated neurologic deficits. The trigger for this focal inflammation is unknown.
After common disorders have been excluded in cases of CNS dysfunction, compatible CSF findings and imaging results may prompt consideration of PACNS. CSF analysis in patients with PACNS typically demonstrates a lymphocytic pleocytosis. MRI abnormalities in PACNS include multiple infarcts in the cortex, deep white matter, or leptomeninges.34 Less specific findings are contrast enhancement in the leptomeninges and white matter disease, both of which may direct the site for meningeal and brain biopsy.
Both brain MRA and cerebral angiography have a limited role in the diagnosis of vasculitis within the CNS. In 18 patients with CNS vasculitis due to autoimmune disease, all had parenchymal abnormalities on MRA but only 65% had evidence of vasculitis on angiography. In 2 retrospective studies of patients with suspected PACNS, abnormal angiograms had a specificity less than 30% for PACNS, whereas brain biopsies had a negative predictive value of 70%.57 Although in practice patients with compatible clinical features are sometimes diagnosed with CNS vasculitis on the basis of angiographic findings, brain biopsy is necessary to differentiate vasculitis from other vasculopathies and to establish a definitive diagnosis.
Before a diagnosis of PACNS is made, care must be taken to exclude infections, neoplasms, and autoimmune processes that cause angiitis of the CNS (Table 1). The presence of any extracranial abnormalities (which were not present in this case) should prompt consideration of an underlying systemic disorder causing a secondary CNS vasculitis and should cast doubt on the diagnosis of PACNS. Meningovascular syphilis and tuberculosis are among the long list of infections that may cause inflammation of the CNS vasculature. Autoimmune disorders that may cause vasculitis inside the brain include polyarteritis nodosa and Wegener's granulomatosis. Reversible cerebral vasoconstrictive disease, which is most commonly seen in women ages 20 to 50, and sympathomimetic toxins such as cocaine and amphetamine may exhibit clinical and angiographic abnormalities indistinguishable from PACNS.89
Infection: Viruses (HIV, varicella‐zoster virus, hepatitis C virus), syphilis, Borrelia burgdorferi, Bartonella, Mycobacterium tuberculosis, fungi (Aspergillus, Coccidioides), bacteria. |
Autoimmune: Polyarteritis nodosa, Wegener's granulomatosis, temporal arteritis, cryoglobulinemic vasculitis, lupus vasculitis, rheumatoid vasculitis. |
Toxins: Amphetamine, cocaine, ephedrine, heroin. |
Malignancy: Primary CNS lymphoma, angioimmunoproliferative disorders, infiltrating glioma. |
There are no prospective trials investigating PACNS treatment. Aggressive immunosuppression with cyclophosphamide and glucocorticoids is the mainstay of treatment. The duration of treatment varies with the severity of the disease and response to therapy. One study suggests that treatment should be continued for 6 to 12 months.10 Neurologic deficits may remain irreversible because of scarring of the affected vessels. Serial brain MRI examinations are often used to follow radiographic resolution during and after the therapy, although radiographic changes do not predict clinical response.11 New abnormalities on MRI, however, delay any tapering of treatment. The availability of neuroimaging studies and immunosuppressive therapy has improved the prognosis of PACNS. One study reported a favorable outcome with a 29% relapse rate and a 10% mortality rate in 54 patients over a mean follow‐up period of 35 months.12
PACNS remains a challenging diagnosis because of its rarity, the wide range of neurologic manifestations, and the difficulty in establishing a diagnosis noninvasively. It is an extremely uncommon disease but should be considered in patients with unexplained neurologic deficits referable to the CNS alone after an exhaustive workup. Ultimately, the diagnosis is made by a thorough history and physical examination, exclusion of underlying conditions (particularly systemic vasculitides and infections), and histological confirmation.
Key Points for Hospitalists
-
Serious disorders that may present with CNS abnormalities and nondiagnostic abnormal findings on lumbar puncture, brain MRI, and cerebral angiography include intravascular lymphoma, prion diseases, cerebritis, paraneoplastic syndromes, and CNS vasculitis.
-
PACNS is a challenging diagnosis with varied clinical features and often normal angiographic findings. In particular, the specificity of brain MRA and cerebral angiography is low. Although PACNS is rare, it should be on the differential diagnosis, as the condition is fatal without prompt treatment.
-
A diagnosis of PACNS is made only after excluding secondary causes of CNS vasculitis such as infections, malignancies, autoimmune conditions, reversible cerebral vasoconstrictive disease, and medications. The diagnosis is confirmed with a biopsy of the brain and meninges.
A 26‐year‐old woman was brought to the emergency department following several episodes of seizures. The patient's friend witnessed several 15‐minute episodes of sudden jerks and tremors of her right arm during which the patient bit her tongue, had word‐finding difficulty, had horizontal eye deviation, and was incontinent of urine. She became unresponsive during the episodes, with incomplete recovery of consciousness between attacks. She was afebrile. Her neurologic exam 4 hours after several seizures revealed word‐finding difficulty and right arm weakness. A complete blood count, chemistry panel including renal and liver function tests, urine toxicology screen, and computed tomography (CT) of the head were normal. After a loading dose of fosphenytoin, the patient did not experience further seizures and was discharged on a maintenance dose of phenytoin.
Over the next week, the patient continued to note a sensation of heaviness in her right arm and felt fatigued. The patient's mother brought her back to the emergency department after witnessing a similar seizure episode that persisted for an hour. On arrival, the patient was no longer seizing.
Although it can sometimes be difficult to differentiate between seizure, stroke, syncope, and other causes of transient loss of consciousness, this constellation of symptoms strongly points to a seizure. I would classify the patient's focal arm movements associated with impaired consciousness as partial complex seizures. One of the first considerations is determining whether the seizure is caused by a systemic process or by an intrinsic central nervous system disorder. Common systemic illnesses include infections, metabolic disturbances, toxins, and malignancies, none of which are evident on the preliminary evaluation. The absence of fever is important as is the time frame (now extending over 1 week) in excluding acute bacterial meningitis. A negative urine toxicology is very helpful but does not exclude the possibility that the seizure is from unmeasured drug intoxication, for example, tricyclic antidepressants, or from drug withdrawal, for example, benzodiazepines, barbiturates, ethanol, and antiepileptic drugs. The persistent right arm heaviness and right arm jerking during the seizures suggest a left cortical focus that the CT scan did not detect. Without a clear diagnosis and with recurrent seizures despite antiepileptic drugs, hospitalization is warranted.
The patient experienced migraine headaches each month during menses. There was no family history of seizures. Her only medication was phenytoin. The mother was unaware of any use of tobacco, alcohol, or recreational drugs. The patient was raised in New Jersey and moved to the San Francisco Bay area 9 months ago. She had no pets and had traveled to Florida and Montreal in the past 6 months. She was a graduate student in performing arts. During the preceding 2 weeks she had been under significant stress and had not slept much in preparation for an upcoming production. The patient's mother was not aware of any head trauma, recent illness, fevers, chills, weight loss, photosensitivity, arthralgias, nausea, vomiting, or diarrhea.
Recent sleep deprivation could provoke seizures in a patient with a latent anatomic focus or metabolic predisposition. Nonadherence to antiepileptic drug therapy is the most common reason for patients to present to the ED with seizures; therefore, I would check a phenytoin level to assess whether she is at a therapeutic level and would consider administering another loading dose. In the absence of immunocompromise or unusual activities or exposures, North American travel does not bring to mind additional etiologies at this time.
On exam, temperature was 37.3C, blood pressure was 148/84 mm Hg, heart rate was 120 per minute, and respiratory rate was 16 per minute. The patient was stuporous and withdrew from painful stimuli. She was unable to speak. Pupils were 4 mm in diameter and reacted to light. No gaze preference or nystagmus was present. There was no meningismus. Deep tendon reflexes were 1+ and symmetrical in both upper and lower extremities. Plantar reflexes were extensor bilaterally. The tone in the right upper extremity was mildly increased compared to the left. The patient demonstrated semipurposeful movement of the limbs, such as reaching for the bed rails with her arms. Examination of the heart, lungs, abdomen, skin, and oropharynx was normal.
The white blood cell count was 22,300/mm3 with 50% neutrophils, 40% lymphocytes, 7% monocytes, and 3% eosinophils. Results of the chemistry panel including electrolytes, glucose, creatinine, and liver enzymes, urinalysis, and thyroid‐stimulating hormone were normal. Serum phenytoin level was 8.1 g/mL. Urine toxicology screen, obtained after the patient had received lorazepam, was positive only for benzodiazepines. A chest radiograph was normal.
The cerebrospinal fluid (CSF) was colorless, containing 35 white blood cells/mm3 (48% lymphocytes, 30% neutrophils, 22% monocytes), 3 red blood cells/mm3, 62 mg/dL protein, and 50 mg/dL glucose. There was no xanthochromia. The CSF was negative for cryptococcal antigen, antibodies to West Nile virus, PCR for herpes simplex viruses‐1 and ‐2, and PCR for Borrelia burgdorferi. CSF bacterial culture, cryptococcal antigen, and AFB stain were negative. The serum antinuclear antibody, rheumatoid factor, and rapid plasma reagin were negative. Serum antibodies to human immunodeficiency virus, hepatitis B and C viruses, Borrelia burgdorferi, and herpes simplex viruses were negative. The erythrocyte sedimentation rate was 25 mm/hr. There was no growth in her blood cultures.
These CSF findings have to be interpreted in light of her clinical picture, as they are congruent with both an aseptic meningitis and encephalitis. In practice, these can be hard to distinguish, but the early and dominant cortical findings (focal neurologic deficits, prominent altered mental status, bilateral extensor plantar reflexes) and absence of meningeal signs favor encephalitis. This CSF profile can be seen in a variety of disease processes causing a meningoencephalitis, including partially treated bacterial meningitis; meningitis due to viruses, fungi, mycobacteria, or atypical bacteria (eg, Listeria); neurosarcoidosis; carcinomatous meningitis; and infection or inflammation from a parameningeal focus in the sinuses, epidural space, or brain parenchyma. Seizure itself can lead to a postictal pleocytosis in the CSF, although this degree of inflammation would be unusual. Many tests can be sent, and the clinicians appropriately focused on some of the most treatable and serious etiologies first. The negative HIV test limits the list of opportunistic pathogens. The negative ANA substantially lowers the likelihood of systemic lupus, an important consideration in a young woman with an inflammatory disorder involving the central nervous system.
Magnetic resonance imaging (MRI) of the brain showed cortical T2 prolongation with significant enhancement with gadolinium in the cortex and leptomeninges of the left parietal and posterotemporal lobes and right cingulate gyrus region (Fig. 1). The patient was admitted to the intensive care unit, and phenytoin and levetiracetam were administered. Over the next several days, she remained afebrile, and her leukocytosis resolved. She continued to have seizures every day despite receiving phenytoin, levetiracetam, and lamotrigine. She was alert and complained about persistent right arm weakness and word‐finding difficulties. Posterior cervical lymphadenopathy at the base of her left occiput was detected on subsequent exam.
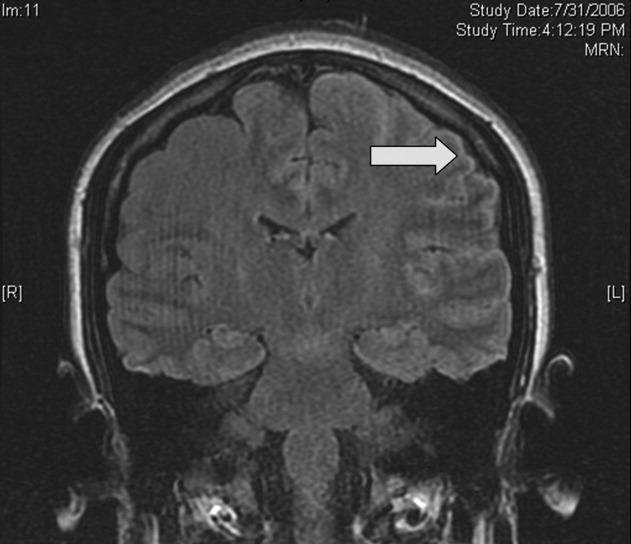
An excisional lymph node biopsy demonstrated extensive necrosis without evidence of granulomata, malignancy, or lymphoproliferative disease. Stains and cultures for bacteria, fungi, and mycobacteria were negative. The patient's electroencephalogram captured epileptiform activity over the left hemisphere 2 hours after a cluster of seizures. MR angiography and cerebral angiography demonstrated no abnormalities.
Despite this additional information, there is no distinguishing clue that points to a single diagnosis. This is a 26‐year‐old healthy, seemingly immunocompetent woman who has had a 2‐week progressive and refractory seizure disorder secondary to a multifocal neuroinvasive process with a CSF pleocytosis. She does not have evidence of a systemic underlying disorder, save for nonspecific localized lymphadenopathy and a transient episode of leukocytosis on admission, and has no distinguishing epidemiological factors or exposures.
Despite my initial concerns for infectious meningoencephalitis, the negative stains, serologies, and cultures of the blood, CSF, and lymph nodes in the setting of a normal immune system and no suspect exposure substantially lower this probability. Arthropod‐borne viruses are still possible, especially West Nile virus, because the serological tests are less sensitive early in the illness, acknowledging that the absence of fever, weakness, and known mosquito bites detracts from this diagnosis. Pathogens that cause regional lymphadenopathy and encephalitis such as Bartonella remain possibilities, as the history of exposure to a kitten can be easily overlooked.
Rheumatologic disorders merit close attention in a young woman, but the negative ANA makes lupus cerebritis unlikely, and the 2 angiograms did not detect evidence of vasculitis. Finally, there is the question of malignancy and other miscellaneous infiltrative disorders (such as sarcoid), which are of importance here because of the multifocal cortical involvement on imaging.
At this point, I would resample the CSF for viral etiologies (eg, West Nile virus) and cytology and would send serum Bartonella serologies. If these studies were negative, a brain biopsy, primarily to exclude malignancy but also to uncover an unsuspected process, would be indicated. I cannot make a definitive diagnosis or find a perfect fit here, but in the absence of strong evidence of an infection, I am concerned about a malignancy, perhaps a low‐grade primary brain tumor.
Brain biopsy of the leptomeninges and cortex of the left parietal lobe showed multiple blood vessels infiltrated by lymphocytes, neutrophils, and eosinophils (Fig. 2). The pattern of inflammation was consistent with primary angiitis of the central nervous system (PACNS). The patient received 1 g of intravenous methylprednisolone on 3 consecutive days, followed by oral prednisone and cyclophosphamide. The seizures ceased, and she made steady progress with rehabilitation therapy. Four months after discharge a cerebral angiogram (done to ensure there was no interval evidence of vasculitis prior to tapering therapy) demonstrated patency of all major intracranial arteries and venous sinuses.
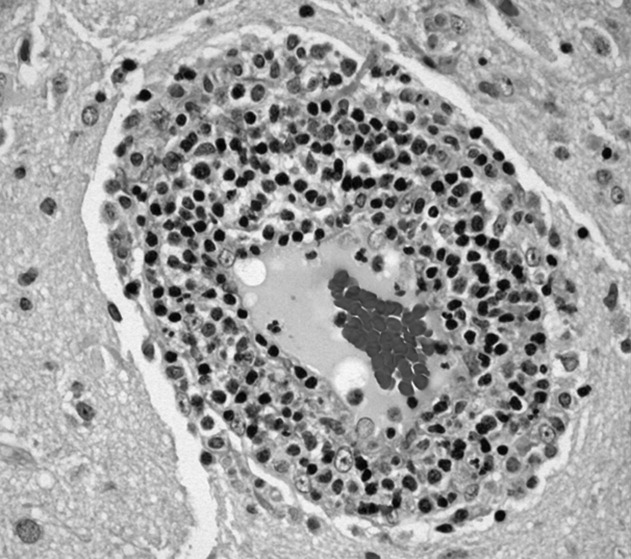
COMMENTARY
When a patient presents with symptoms or signs referable to the central nervous system (CNS), hospitalists must simultaneously consider primary neurologic disorders and systemic diseases that involve the CNS. Initial evaluation includes a thorough history and physical examination, basic lab studies, routine CSF analysis, and neuroimaging (often a CT scan of the head). Complicated neurologic cases may warrant more elaborate testing including EEG, brain MRI, cerebral angiography, and specialized blood and CSF studies. Clinicians may still find themselves faced with a patient who has clear CNS dysfunction but no obvious diagnosis despite an exhaustive and expensive evaluation. Several disorders match this profile including intravascular lymphoma, prion diseases, paraneoplastic syndromes, and cerebritis. Primary angiitis of the central nervous system (PACNS), a rare disorder characterized by inflammation of the medium‐sized and small arteries of the CNS, is among these disorders. Although the aforementioned diseases may sometimes have suggestive or even pathognomonic features (eg, the string of beads angiographic appearance in vasculitides), they are challenging to diagnose when such findings are absent.
Like any vasculitis of the CNS, PACNS may present with a wide spectrum of clinical features.12 Although headache and altered mental status are the most common complaints, paresis, seizures, ataxia, visual changes, and aphasia have all been described. The onset of symptoms ranges from acute to chronic, and neurologic deficits can be focal or diffuse. Systemic manifestations such as fever and weight loss are rare. The average age of onset is 42 years, with no significant sex preponderance. The histopathology of PACNS is granulomatous inflammation of arteries in the parenchyma and leptomeninges of the brain and less commonly in the spinal cord. The narrowing of the affected vessels causes cerebral ischemia and the associated neurologic deficits. The trigger for this focal inflammation is unknown.
After common disorders have been excluded in cases of CNS dysfunction, compatible CSF findings and imaging results may prompt consideration of PACNS. CSF analysis in patients with PACNS typically demonstrates a lymphocytic pleocytosis. MRI abnormalities in PACNS include multiple infarcts in the cortex, deep white matter, or leptomeninges.34 Less specific findings are contrast enhancement in the leptomeninges and white matter disease, both of which may direct the site for meningeal and brain biopsy.
Both brain MRA and cerebral angiography have a limited role in the diagnosis of vasculitis within the CNS. In 18 patients with CNS vasculitis due to autoimmune disease, all had parenchymal abnormalities on MRA but only 65% had evidence of vasculitis on angiography. In 2 retrospective studies of patients with suspected PACNS, abnormal angiograms had a specificity less than 30% for PACNS, whereas brain biopsies had a negative predictive value of 70%.57 Although in practice patients with compatible clinical features are sometimes diagnosed with CNS vasculitis on the basis of angiographic findings, brain biopsy is necessary to differentiate vasculitis from other vasculopathies and to establish a definitive diagnosis.
Before a diagnosis of PACNS is made, care must be taken to exclude infections, neoplasms, and autoimmune processes that cause angiitis of the CNS (Table 1). The presence of any extracranial abnormalities (which were not present in this case) should prompt consideration of an underlying systemic disorder causing a secondary CNS vasculitis and should cast doubt on the diagnosis of PACNS. Meningovascular syphilis and tuberculosis are among the long list of infections that may cause inflammation of the CNS vasculature. Autoimmune disorders that may cause vasculitis inside the brain include polyarteritis nodosa and Wegener's granulomatosis. Reversible cerebral vasoconstrictive disease, which is most commonly seen in women ages 20 to 50, and sympathomimetic toxins such as cocaine and amphetamine may exhibit clinical and angiographic abnormalities indistinguishable from PACNS.89
Infection: Viruses (HIV, varicella‐zoster virus, hepatitis C virus), syphilis, Borrelia burgdorferi, Bartonella, Mycobacterium tuberculosis, fungi (Aspergillus, Coccidioides), bacteria. |
Autoimmune: Polyarteritis nodosa, Wegener's granulomatosis, temporal arteritis, cryoglobulinemic vasculitis, lupus vasculitis, rheumatoid vasculitis. |
Toxins: Amphetamine, cocaine, ephedrine, heroin. |
Malignancy: Primary CNS lymphoma, angioimmunoproliferative disorders, infiltrating glioma. |
There are no prospective trials investigating PACNS treatment. Aggressive immunosuppression with cyclophosphamide and glucocorticoids is the mainstay of treatment. The duration of treatment varies with the severity of the disease and response to therapy. One study suggests that treatment should be continued for 6 to 12 months.10 Neurologic deficits may remain irreversible because of scarring of the affected vessels. Serial brain MRI examinations are often used to follow radiographic resolution during and after the therapy, although radiographic changes do not predict clinical response.11 New abnormalities on MRI, however, delay any tapering of treatment. The availability of neuroimaging studies and immunosuppressive therapy has improved the prognosis of PACNS. One study reported a favorable outcome with a 29% relapse rate and a 10% mortality rate in 54 patients over a mean follow‐up period of 35 months.12
PACNS remains a challenging diagnosis because of its rarity, the wide range of neurologic manifestations, and the difficulty in establishing a diagnosis noninvasively. It is an extremely uncommon disease but should be considered in patients with unexplained neurologic deficits referable to the CNS alone after an exhaustive workup. Ultimately, the diagnosis is made by a thorough history and physical examination, exclusion of underlying conditions (particularly systemic vasculitides and infections), and histological confirmation.
Key Points for Hospitalists
-
Serious disorders that may present with CNS abnormalities and nondiagnostic abnormal findings on lumbar puncture, brain MRI, and cerebral angiography include intravascular lymphoma, prion diseases, cerebritis, paraneoplastic syndromes, and CNS vasculitis.
-
PACNS is a challenging diagnosis with varied clinical features and often normal angiographic findings. In particular, the specificity of brain MRA and cerebral angiography is low. Although PACNS is rare, it should be on the differential diagnosis, as the condition is fatal without prompt treatment.
-
A diagnosis of PACNS is made only after excluding secondary causes of CNS vasculitis such as infections, malignancies, autoimmune conditions, reversible cerebral vasoconstrictive disease, and medications. The diagnosis is confirmed with a biopsy of the brain and meninges.
- Medical progress: small‐vessel vasculitis.N Engl J Med.1997;337:1512–1523. , .
- Arthritis and Allied Conditions: A Textbook of Rheumatology.15th ed.Philadelphia:Lippincott Williams 2005. , .
- Primary angiitis of the central nervous system: unusual MR appearance.Am J Neuroradiol.1994;15:331–334. , , , .
- Radiographic features of central nervous system vasculitis.Neurol Clin.1997;15:779–804. , , , .
- Primary angiitis of the central nervous system at conventional angiography.Radiology.2004;233:878–882. , , , , , .
- CNS vasculitis in autoimmune disease: MR imaging findings and correlation with angiography.Am J Neuroradiol.1999;20:75–85. , , , , .
- Limitations of invasive modalities in the diagnosis of primary angiitis of the central nervous system.J Rheumatol.1995;22:662–667. , .
- Amphetamine abuse and intracranial haemorrhage.J R Soc Med.2000;93:472–477. , .
- Drug‐induced vasculitis.Curr Opin Rheumatol.1996;8:34–40. , .
- Vasculitis in the central nervous system.Arthritis Rheum.1997;40:1189–1201. , , .
- Therapy of systemic vasculitis.Neurol Clin.1997;15:973–991. .
- Long‐term outcomes of patients with primary angiitis of the central nervous system.Arthritis Rheum.2000;43:S162. , , , et al.
- Medical progress: small‐vessel vasculitis.N Engl J Med.1997;337:1512–1523. , .
- Arthritis and Allied Conditions: A Textbook of Rheumatology.15th ed.Philadelphia:Lippincott Williams 2005. , .
- Primary angiitis of the central nervous system: unusual MR appearance.Am J Neuroradiol.1994;15:331–334. , , , .
- Radiographic features of central nervous system vasculitis.Neurol Clin.1997;15:779–804. , , , .
- Primary angiitis of the central nervous system at conventional angiography.Radiology.2004;233:878–882. , , , , , .
- CNS vasculitis in autoimmune disease: MR imaging findings and correlation with angiography.Am J Neuroradiol.1999;20:75–85. , , , , .
- Limitations of invasive modalities in the diagnosis of primary angiitis of the central nervous system.J Rheumatol.1995;22:662–667. , .
- Amphetamine abuse and intracranial haemorrhage.J R Soc Med.2000;93:472–477. , .
- Drug‐induced vasculitis.Curr Opin Rheumatol.1996;8:34–40. , .
- Vasculitis in the central nervous system.Arthritis Rheum.1997;40:1189–1201. , , .
- Therapy of systemic vasculitis.Neurol Clin.1997;15:973–991. .
- Long‐term outcomes of patients with primary angiitis of the central nervous system.Arthritis Rheum.2000;43:S162. , , , et al.
Hospitalist–Care Coordinator Team
Many hospitalists work with clinical coordinators and case managers.13 The descriptions of these roles often overlap4 and commonly include activities such as obtaining medical records, expediting tests and procedures, coordinating the plan of care with other health care providers, assessing postdischarge needs, completing discharge paperwork, and arranging follow‐up visits.2, 5, 6 Despite the potential to improve patient care and hospital efficiency, few studies have formally evaluated the impact of these roles. Moher et al. found that adding a clinical coordinator to a general medical team decreased length of stay (LOS) and improved patient satisfaction.5 However, this study was conducted at a time when the LOS was routinely longer than it is today. Forster et al. found that adding a clinical coordinator to a general medical team resulted in improved patient satisfaction but did not reduce length of stay or risk of adverse events occurring following hospital discharge.6 Both these studies evaluated the impact of adding a clinical coordinator to resident‐covered medical teams. Yet many hospitalists deliver care without residents, limiting the generalizability of the findings from these studies.
To date, no studies have evaluated the impact of clinical coordinators, case managers, or other nonphysician providers on the hospitalist work experience. This is surprising, as hospital medicine group leaders list daily workload and work hours among their top concerns.7 Clinical coordinators have the potential to improve patient care and hospital efficiency while simultaneously improving the experience of the hospitalists with whom they work. We conducted this study to evaluate the impact of a hospitalistcare coordinator team on hospitalist work experience, patient satisfaction, and hospital efficiency.
METHODS
Setting
The study was conducted on the hospitalist service at Northwestern Memorial Hospital (NMH), a 753‐bed hospital in Chicago, Illinois. The hospitalist service is staffed by 5 hospitalists on duty at a time. Hospitalists work without residents and are on service for 7 consecutive days, usually followed by 7 consecutive days off. Daytime admissions are distributed among all hospitalists on duty in a consecutive fashion. A night float hospitalist performs admissions and all cross‐cover activities from 7:00 PM until 7:00 AM. Nighttime admissions are distributed to day hospitalists based on each hospitalist's daily census.
Study Design
Funding was provided by the hospital for a 12‐week study, and hospital administrative leaders collaborated as part of the research team. During each of the 12 weeks from September 2006 through November 2006, half the hospitalists on duty each week were randomly assigned to work with a hospitalist care coordinator (HCC) in a team approach. Hospitalists not assigned to work with a hospitalist care coordinator continued to work in their usual fashion, which included working with unit‐based care coordinators (UCCs). UCCs follow all patients on a 30‐bed medicine unit but generally do not round with physicians. HCCs performed all the activities that UCCs perform but also performed additional key activities (see Table 1) and worked in a team approach with their hospitalist. Unit‐based social workers and discharge planners were available for all hospitalists during the study. During each day patients were admitted consecutively to one of the hospitalists on service, regardless of their assignment to work with or without an HCC. Similarly, night admissions were distributed to hospitalists without regard to their assignment to work with or without an HCC.
Activity type | Activities performed by both UCCs and HCCs | Additional activities performed by HCCs only |
---|---|---|
Care coordination | Document the interdisciplinary plan of care | Ensure collaboration in formulating the plan of care |
Obtain outside medical records | ||
Inform staff nurse of stat orders during rounds | ||
Obtain certain test results (eg, preliminary echo reports) | ||
Patient and family needs | Address patient and family concerns | Proactively identify and address patient and family concerns |
Schedule family meetings | ||
Efficiency of care delivery | Remediate barriers that impede plan of care and/or discharge | Identify barriers that impede plan of care |
Identify and document avoidable days | Ensure tests are scheduled | |
Discharge process | Coordinate discharge plans with social work and discharge planner | Schedule and confirm follow‐up appointments |
Initiate discharge instructions | ||
Write discharge prescriptionsverified and signed by physician | ||
Review discharge instructions with patient and/or family |
HospitalistCare Coordinator Team
Four HCCs were used in this study. All 4 were registered nurses with specialized training in case management. Prior to the start of the study, the investigators held meetings with hospitalists and the HCCs to describe this new role, the work flow for the hospitalistcare coordinator team, and work activities appropriate for the HCC. Activities related to the discharge process were emphasized as a key feature of the HCC role. Hospitalists and HCCs were instructed to round together as a team each morning. They were advised to collaborate on the daily plan of care and assign specific activities for each to accomplish. During the study weekly meetings were held with the HCCs, the hospitalists with whom they were finishing the week, and the hospitalists with whom they were scheduled to work during the upcoming week. The purpose of these meetings was to ensure that the work flow and work activities were optimal.
Outcome Measures
At the completion of each week, all hospitalists on service were given an anonymous Web‐based survey designed to assess their satisfaction and perceived work efficiency. Hospitalists were asked to rate the efficiency of various work activities during the preceding week on a 5 point Likert scale (1 = very inefficient, 2 = somewhat inefficient, 3 = neutral, 4 = somewhat efficient, 5 = very efficient). Hospitalists who had worked with an HCC were also asked whether they thought working with an HCC improved their efficiency and increased their job satisfaction. We postulated that patient satisfaction with the discharge process might improve with use of the hospitalistHCC team. Therefore, patient satisfaction was assessed by telephone interviews conducted 714 days after discharge. Because of resource limitations, we were only able to interview patient during the second half of the study. Patients were asked to rate their satisfaction with the clarity of verbal and written discharge instructions as well as their overall satisfaction with hospital discharge using a 10‐point Likert scale (from 1 = least satisfied to 10 = most satisfied). Hospital databases provided information on patient demographics, LOS, and cost.
Data Analysis
All analyses were conducted using Stata version 9.0 (StataCorp LP, College Station, TX). Patient characteristics were compared using chi‐square and t tests. Responses to the hospitalist survey for the weeks when they worked independently and the weeks when they worked as a hospitalistHCC team were compared using the Wilcoxon rank sum test. To adjust for the clustering of responses by physicians (ie, individual physicians completed more than 1 survey), we used linear regression and the cluster option. The results were very similar, and only the P values from the Wilcoxon rank sum test are presented. Unadjusted LOS and cost were compared using the Wilcoxon rank sum test. We also conducted multivariate linear regressions using log‐transformed LOS and log‐transformed cost as dependent variables. The independent variable was the team type (whether patients were cared for by a hospitalistHCC team or a hospitalist working independently); age, sex, ethnicity, payer type, and diagnosis‐related group (DRG) weight were included as covariates, and P values were adjusted for physician clustering. We hypothesized a priori that the HCC would have no effect on the LOS of or cost for patients whose hospitalizations were very short. We therefore conducted secondary analyses in which we eliminated patients with an LOS of 1 day or less.
RESULTS
There were 356 patients cared for by hospitalistHCC teams and 337 patients cared for by control hospitalists. Of the 60 weeks of hospitalist service of the study, hospitalistHCC teams accounted for 31 weeks (52%) and control hospitalists for 29 weeks (48%). Patients cared for by the hospitalistHCC teams were similar in age, sex, ethnicity, payer type, and DRG weight to those cared for by control hospitalists (see Table 2).
HospitalistHCC team | Control | P value | |
---|---|---|---|
n (%) or mean SD | |||
Patients, n (%) | 356 (51.4%) | 337 (48.6%) | |
Age (years) | 59.3 18.6 | 59.0 20.1 | .86 |
Women (%) | 190 (53.4%) | 192 (57.0%) | .34 |
Ethnicity | .74 | ||
White | 182 (51.1%) | 174 (51.6%) | |
Black | 111 (31.2%) | 114 (33.8%) | |
Hispanic | 23 (6.5%) | 21 (6.2%) | |
Asian | 5 (1.4%) | 4 (1.2%) | |
Other | 35 (9.8%) | 24 (7.1%) | |
Payer | .47 | ||
Medicare | 177 (49.7%) | 168 (49.9%) | |
Private | 76 (21.4%) | 69 (20.5%) | |
Medicaid | 43 (12.1%) | 44 (13.1%) | |
Capitated | 43 (12.1%) | 31 (9.2%) | |
Other | 17 (4.8%) | 25 (7.4%) | |
Diagnosis‐related group weight | 1.1 0.8 | 1.2 0.8 | .31 |
Sixty surveys were completed by hospitalists at the end of their week on service (response rate 100%). Of the 31 responses from hospitalists completing a hospitalistHCC team week, 28 (90%) reported that working with an HCC improved their efficiency and 28 (90%) that working with an HCC improved their job satisfaction. The hospitalists indicated that working with an HCC significantly improved the efficiency of most of their activities (see Table 3). Specifically, activities related to communication with nurses and patients and activities involving discharge planning and execution were improved with the use of an HCC. As would be expected, certain other activities did not improve. For example, there were no differences between the groups in the perceived efficiency of performing histories and physicals or placing admission orders. For activities that were significantly different, the Wilcoxon rank sum test and linear regression analysis adjusting for physician clustering showed identical results.
HospitalistHCC Team | Control | P value | |
---|---|---|---|
Mean score SD* | |||
| |||
Performing histories and physicals | 3.94 0.77 | 3.93 0.84 | .98 |
Performing medication reconciliation | 3.35 1.08 | 2.03 1.18 | < .001 |
Placing admission orders | 3.94 0.73 | 4.00 0.87 | .57 |
Communicating with nurses | 4.45 0.68 | 3.14 1.09 | < .001 |
Communicating with consultants | 3.65 0.75 | 3.34 1.04 | .25 |
Communicating with patients | 4.42 0.62 | 3.62 1.01 | < .001 |
Communicating with families | 4.32 0.70 | 2.89 1.14 | < .001 |
Coordinating discharge plans | 4.74 0.51 | 2.76 1.18 | < .001 |
Making/updating sign‐out | 3.55 0.72 | 3.49 0.83 | .55 |
Making discharge instructions | 4.29 0.74 | 3.10 1.01 | < .001 |
Going over discharge instructions | 4.48 0.57 | 2.76 1.15 | < .001 |
Writing discharge prescriptions | 3.87 0.67 | 2.52 1.21 | < .001 |
Arranging follow‐up appointments | 4.19 0.75 | 2.03 1.09 | < .001 |
Seventy‐one of 196 eligible patients (36%) completed the discharge satisfaction interview. Of the 71 patients interviewed, 44 (62%) were cared for by hospitalistHCC teams and 27 (38%) were cared for by control hospitalists. Patient satisfaction with the clarity of the verbal and written discharge instructions and overall satisfaction with hospital discharge was similar between the 2 groups (see Table 4).
HospitalistHCC Team (n = 44) | Control (n = 27) | P value | |
---|---|---|---|
Mean score (SD)* | |||
| |||
Clarity of verbal discharge instructions | 8.86 ( 2.31) | 8.44 ( 2.63) | 0.52 |
Clarity of written discharge instructions | 8.95 ( 2.30) | 8.93 ( 2.54) | 0.78 |
Overall satisfaction with hospital discharge | 8.57 ( 2.42) | 8.37 ( 2.90) | 0.94 |
The unadjusted mean LOS for patients cared for by hospitalistHCC teams was 4.70 4.15 days compared with 5.07 3.99 days for patients cared for by control hospitalists (P = .005; see Table 5). The unadjusted mean cost for patients cared for by hospitalistHCC teams was $10,052.96 $11,708.73 compared with $11,703.19 $20,455.78 for patients cared for by control hospitalists (P = .008). In multivariate analysis using age, sex, ethnicity, payer type, and DRG weight as independent variables and adjusting for physician clustering, LOS remained lower for patients cared for by hospitalistHCC teams; however, this result was not statistically significant (0.28 days, P = .17). Similar multivariate regression analysis showed a trend toward lower cost for patients cared for by the hospitalistHCC teams (585.62, P = .15).
Unadjusted mean (SD) | P value for unadjusted difference* | Adjusted difference with hospitalistHCC team | P value for adjusted difference | |
---|---|---|---|---|
| ||||
Length of stay | ||||
HospitalistHCC teams | 4.70 (4.15) | .005 | 0.28 | .17 |
Control hospitalists | 5.07 (3.99) | |||
Cost | ||||
HospitalistHCC teams | 10,052.96 (11,708.73) | .008 | 585.62 | .15 |
Control hospitalists | 11,703.19 (20,455.78) |
DISCUSSION
Our study found that hospitalists working in a team approach with an HCC rated the efficiency of their daily work and their job satisfaction significantly higher than did control hospitalists. Specific areas of improved efficiency included communication activities and activities related to hospital discharge. A prior study conducted by our group found that hospitalists spend a lot of time on indirect patient care activities such as communication and activities related to the discharge process, while spending relatively little time on direct patient care.8 Improving the efficiency of indirect patient care activities of hospitalists is likely to improve their job satisfaction. The importance of improving hospitalist workload and job satisfaction is underscored by the relatively high number of hospitalists at risk for burnout9 and the growing concern about daily workload among hospital medicine group leaders.7
Patient satisfaction was not significantly affected by the use of the hospitalistHCC team in our study. A priori, we postulated that patient satisfaction with the discharge process might improve with the use of the hospitalistHCC team. We therefore limited survey questions to assessing only satisfaction with hospital discharge rather than other aspects of patient hospital care. A recent study reported that patients rated the quality of discharge instructions significantly lower than they rated the overall quality of their hospital stay.10 However, the patients in our study gave high ratings to both discharge instructions and overall satisfaction with hospital discharge. This may explain why we were unable to detect a difference. Our study was limited by the relatively small number of patients we were able to contact to assess satisfaction. Previous studies evaluating the impact of care coordinators either did not assess patient satisfaction with discharge5 or found no difference in satisfaction with hospital discharge.6
Although our study did not find a difference in patient satisfaction with the discharge process, we believe the hospitalistHCC model has the potential to complement efforts to reduce the risk of adverse events as patients transition out of the hospital. It has been reported that 12% of patients have a preventable or ameliorable adverse event in the period immediately following hospital discharge.11, 12 Although Forster et al. did not find a reduction in the risk of adverse events with the addition of a clinical coordinator to a general medical team, they noted incongruence between the coordinator's role and the outcomes measured.6 Similarly, we would need to modify the role of the HCC from a position designed mainly to improve efficiency to one that complements efforts to improve the quality of the discharge process. Possible ways to enhance the HCC role in this regard include increasing the emphasis on and training in patient education skills. Several recently published articles have emphasized the need to redesign the discharge process in an effort to reduce the risk of adverse events following hospital discharge.1315 A modified HCC role might be an essential feature of a redesigned multidisciplinary discharge process.
We were unable to demonstrate improved efficiency for the hospital. Although LOS and cost were lower for patients cared for by the hospitalistHCC teams, the difference was not statistically significant. One possible explanation for why we did not observe a larger reduction in LOS is that our hospitalist service had a lower‐than‐average patient volume during the study period. The lower volume mirrored an unanticipated dip in hospital volume during the same period. Specifically, our service normally discharges an average of 338 patients per month, but during the study period we discharged an average of 235 patients per month. A potential LOS and cost benefit may have been attenuated by the relatively low volume, as hospitalists had ample time to dedicate to communication and coordination of discharge plans.
Our study had several limitations. It was conducted on a nonteaching hospitalist service at a single site. Hospitalist practices vary widely in their staffing and scheduling models. As previously mentioned, we were only able to perform patient satisfaction surveys during the second half of the study period. In addition, hospitalistHCC team patients made up a larger percentage of the patient survey responses (62%) than did control hospitalist patients (38%). This may have affected our ability to detect differences in satisfaction with the hospital discharge process. As also previously noted, our patient volume was lower than normal during the study period. We believe that a higher volume would have magnified differences in hospitalists' perceived efficiency and perhaps resulted in significant improvements in LOS and cost. Finally, the hospital provided funding for only a 12‐week study. This limited our sample size and the power of the study to detect important differences. It is possible that a larger sample size and/or longer study period may have been able to demonstrate a statistically significant improvement in LOS and cost.
Our findings are of particular importance in light of the persistent concerns about hospitalist workload and job satisfaction. Although many hospitalists work with clinical coordinators and case managers, we believe that having the formal structure of a hospitalistcare coordinator team was the key element to improving hospitalist efficiency and satisfaction. We hope that our study is a precursor to research evaluating models of delivering hospital care and their impact on hospitalist work experience, hospital efficiency, and patient outcomes.
- Innovations in the management of hospitalized patients.Nurse Pract.2006;Suppl:2–3. .
- Hospitalists and case managers: the perfect partnership.Lippincotts Case Manag.2004;9:280–286. , .
- Productive interdisciplinary team relationships: the hospitalist and the case manager.Lippincotts Case Manag.2006;11:160–164. , .
- Care coordinator—blending roles to improve patient outcomes.J Nurs Manage.1997;28:49–52. , , .
- Effects of a medical team coordinator on length of hospital stay.Can Med Assoc J.1992;146:511–515. , , , .
- Effect of a nurse team coordinator on outcomes for hospitalized medicine patients.Am J Med.2005;118:1148–1153. , , , et al.
- Society of Hospital Medicine. Available at: http://www.hospitalmedicine.org/Content/NavigationMenu/ResourceCenter/Surveys/Surveys1.htm. Accessed April 2,2007.
- How hospitalists spend their time: insights on efficiency and safety.J Hosp Med.2006;1:88–93. , , .
- Characteristics and work experiences of hospitalists in the United States.Arch Intern Med.2001;161:851–858. , , , , .
- Patient perception of quality in discharge instruction.Patient Educ Couns.2005;59:56–68. , , , , , .
- Adverse events among medical patient after hospital discharge.Can Med Assoc J.2004;170:345–349. , , , et al.
- The incidence and severity of adverse events affecting patients after discharge from the hospital.Ann Intern Med.2003;138:161–167. , , , , .
- Lost in transition: challenges and opportunities for improving the quality of transitional care.Ann Intern Med.2004;141:533–536. , .
- Transition of care for hospitalized elderly patients—development of a discharge checklist for hospitalists.J Hosp Med.2006;1:354–360. , , , et al.
- Re‐engineering the hospital discharge—an example of a multifaceted process evaluation. Advances in Patient Safety: From Research to Implementation. Vol.2,Concepts and Methodology. AHRQ publication 05‐0021‐2.Rockville, MD:AHRQ;2005. p379–394. , , , et al.
Many hospitalists work with clinical coordinators and case managers.13 The descriptions of these roles often overlap4 and commonly include activities such as obtaining medical records, expediting tests and procedures, coordinating the plan of care with other health care providers, assessing postdischarge needs, completing discharge paperwork, and arranging follow‐up visits.2, 5, 6 Despite the potential to improve patient care and hospital efficiency, few studies have formally evaluated the impact of these roles. Moher et al. found that adding a clinical coordinator to a general medical team decreased length of stay (LOS) and improved patient satisfaction.5 However, this study was conducted at a time when the LOS was routinely longer than it is today. Forster et al. found that adding a clinical coordinator to a general medical team resulted in improved patient satisfaction but did not reduce length of stay or risk of adverse events occurring following hospital discharge.6 Both these studies evaluated the impact of adding a clinical coordinator to resident‐covered medical teams. Yet many hospitalists deliver care without residents, limiting the generalizability of the findings from these studies.
To date, no studies have evaluated the impact of clinical coordinators, case managers, or other nonphysician providers on the hospitalist work experience. This is surprising, as hospital medicine group leaders list daily workload and work hours among their top concerns.7 Clinical coordinators have the potential to improve patient care and hospital efficiency while simultaneously improving the experience of the hospitalists with whom they work. We conducted this study to evaluate the impact of a hospitalistcare coordinator team on hospitalist work experience, patient satisfaction, and hospital efficiency.
METHODS
Setting
The study was conducted on the hospitalist service at Northwestern Memorial Hospital (NMH), a 753‐bed hospital in Chicago, Illinois. The hospitalist service is staffed by 5 hospitalists on duty at a time. Hospitalists work without residents and are on service for 7 consecutive days, usually followed by 7 consecutive days off. Daytime admissions are distributed among all hospitalists on duty in a consecutive fashion. A night float hospitalist performs admissions and all cross‐cover activities from 7:00 PM until 7:00 AM. Nighttime admissions are distributed to day hospitalists based on each hospitalist's daily census.
Study Design
Funding was provided by the hospital for a 12‐week study, and hospital administrative leaders collaborated as part of the research team. During each of the 12 weeks from September 2006 through November 2006, half the hospitalists on duty each week were randomly assigned to work with a hospitalist care coordinator (HCC) in a team approach. Hospitalists not assigned to work with a hospitalist care coordinator continued to work in their usual fashion, which included working with unit‐based care coordinators (UCCs). UCCs follow all patients on a 30‐bed medicine unit but generally do not round with physicians. HCCs performed all the activities that UCCs perform but also performed additional key activities (see Table 1) and worked in a team approach with their hospitalist. Unit‐based social workers and discharge planners were available for all hospitalists during the study. During each day patients were admitted consecutively to one of the hospitalists on service, regardless of their assignment to work with or without an HCC. Similarly, night admissions were distributed to hospitalists without regard to their assignment to work with or without an HCC.
Activity type | Activities performed by both UCCs and HCCs | Additional activities performed by HCCs only |
---|---|---|
Care coordination | Document the interdisciplinary plan of care | Ensure collaboration in formulating the plan of care |
Obtain outside medical records | ||
Inform staff nurse of stat orders during rounds | ||
Obtain certain test results (eg, preliminary echo reports) | ||
Patient and family needs | Address patient and family concerns | Proactively identify and address patient and family concerns |
Schedule family meetings | ||
Efficiency of care delivery | Remediate barriers that impede plan of care and/or discharge | Identify barriers that impede plan of care |
Identify and document avoidable days | Ensure tests are scheduled | |
Discharge process | Coordinate discharge plans with social work and discharge planner | Schedule and confirm follow‐up appointments |
Initiate discharge instructions | ||
Write discharge prescriptionsverified and signed by physician | ||
Review discharge instructions with patient and/or family |
HospitalistCare Coordinator Team
Four HCCs were used in this study. All 4 were registered nurses with specialized training in case management. Prior to the start of the study, the investigators held meetings with hospitalists and the HCCs to describe this new role, the work flow for the hospitalistcare coordinator team, and work activities appropriate for the HCC. Activities related to the discharge process were emphasized as a key feature of the HCC role. Hospitalists and HCCs were instructed to round together as a team each morning. They were advised to collaborate on the daily plan of care and assign specific activities for each to accomplish. During the study weekly meetings were held with the HCCs, the hospitalists with whom they were finishing the week, and the hospitalists with whom they were scheduled to work during the upcoming week. The purpose of these meetings was to ensure that the work flow and work activities were optimal.
Outcome Measures
At the completion of each week, all hospitalists on service were given an anonymous Web‐based survey designed to assess their satisfaction and perceived work efficiency. Hospitalists were asked to rate the efficiency of various work activities during the preceding week on a 5 point Likert scale (1 = very inefficient, 2 = somewhat inefficient, 3 = neutral, 4 = somewhat efficient, 5 = very efficient). Hospitalists who had worked with an HCC were also asked whether they thought working with an HCC improved their efficiency and increased their job satisfaction. We postulated that patient satisfaction with the discharge process might improve with use of the hospitalistHCC team. Therefore, patient satisfaction was assessed by telephone interviews conducted 714 days after discharge. Because of resource limitations, we were only able to interview patient during the second half of the study. Patients were asked to rate their satisfaction with the clarity of verbal and written discharge instructions as well as their overall satisfaction with hospital discharge using a 10‐point Likert scale (from 1 = least satisfied to 10 = most satisfied). Hospital databases provided information on patient demographics, LOS, and cost.
Data Analysis
All analyses were conducted using Stata version 9.0 (StataCorp LP, College Station, TX). Patient characteristics were compared using chi‐square and t tests. Responses to the hospitalist survey for the weeks when they worked independently and the weeks when they worked as a hospitalistHCC team were compared using the Wilcoxon rank sum test. To adjust for the clustering of responses by physicians (ie, individual physicians completed more than 1 survey), we used linear regression and the cluster option. The results were very similar, and only the P values from the Wilcoxon rank sum test are presented. Unadjusted LOS and cost were compared using the Wilcoxon rank sum test. We also conducted multivariate linear regressions using log‐transformed LOS and log‐transformed cost as dependent variables. The independent variable was the team type (whether patients were cared for by a hospitalistHCC team or a hospitalist working independently); age, sex, ethnicity, payer type, and diagnosis‐related group (DRG) weight were included as covariates, and P values were adjusted for physician clustering. We hypothesized a priori that the HCC would have no effect on the LOS of or cost for patients whose hospitalizations were very short. We therefore conducted secondary analyses in which we eliminated patients with an LOS of 1 day or less.
RESULTS
There were 356 patients cared for by hospitalistHCC teams and 337 patients cared for by control hospitalists. Of the 60 weeks of hospitalist service of the study, hospitalistHCC teams accounted for 31 weeks (52%) and control hospitalists for 29 weeks (48%). Patients cared for by the hospitalistHCC teams were similar in age, sex, ethnicity, payer type, and DRG weight to those cared for by control hospitalists (see Table 2).
HospitalistHCC team | Control | P value | |
---|---|---|---|
n (%) or mean SD | |||
Patients, n (%) | 356 (51.4%) | 337 (48.6%) | |
Age (years) | 59.3 18.6 | 59.0 20.1 | .86 |
Women (%) | 190 (53.4%) | 192 (57.0%) | .34 |
Ethnicity | .74 | ||
White | 182 (51.1%) | 174 (51.6%) | |
Black | 111 (31.2%) | 114 (33.8%) | |
Hispanic | 23 (6.5%) | 21 (6.2%) | |
Asian | 5 (1.4%) | 4 (1.2%) | |
Other | 35 (9.8%) | 24 (7.1%) | |
Payer | .47 | ||
Medicare | 177 (49.7%) | 168 (49.9%) | |
Private | 76 (21.4%) | 69 (20.5%) | |
Medicaid | 43 (12.1%) | 44 (13.1%) | |
Capitated | 43 (12.1%) | 31 (9.2%) | |
Other | 17 (4.8%) | 25 (7.4%) | |
Diagnosis‐related group weight | 1.1 0.8 | 1.2 0.8 | .31 |
Sixty surveys were completed by hospitalists at the end of their week on service (response rate 100%). Of the 31 responses from hospitalists completing a hospitalistHCC team week, 28 (90%) reported that working with an HCC improved their efficiency and 28 (90%) that working with an HCC improved their job satisfaction. The hospitalists indicated that working with an HCC significantly improved the efficiency of most of their activities (see Table 3). Specifically, activities related to communication with nurses and patients and activities involving discharge planning and execution were improved with the use of an HCC. As would be expected, certain other activities did not improve. For example, there were no differences between the groups in the perceived efficiency of performing histories and physicals or placing admission orders. For activities that were significantly different, the Wilcoxon rank sum test and linear regression analysis adjusting for physician clustering showed identical results.
HospitalistHCC Team | Control | P value | |
---|---|---|---|
Mean score SD* | |||
| |||
Performing histories and physicals | 3.94 0.77 | 3.93 0.84 | .98 |
Performing medication reconciliation | 3.35 1.08 | 2.03 1.18 | < .001 |
Placing admission orders | 3.94 0.73 | 4.00 0.87 | .57 |
Communicating with nurses | 4.45 0.68 | 3.14 1.09 | < .001 |
Communicating with consultants | 3.65 0.75 | 3.34 1.04 | .25 |
Communicating with patients | 4.42 0.62 | 3.62 1.01 | < .001 |
Communicating with families | 4.32 0.70 | 2.89 1.14 | < .001 |
Coordinating discharge plans | 4.74 0.51 | 2.76 1.18 | < .001 |
Making/updating sign‐out | 3.55 0.72 | 3.49 0.83 | .55 |
Making discharge instructions | 4.29 0.74 | 3.10 1.01 | < .001 |
Going over discharge instructions | 4.48 0.57 | 2.76 1.15 | < .001 |
Writing discharge prescriptions | 3.87 0.67 | 2.52 1.21 | < .001 |
Arranging follow‐up appointments | 4.19 0.75 | 2.03 1.09 | < .001 |
Seventy‐one of 196 eligible patients (36%) completed the discharge satisfaction interview. Of the 71 patients interviewed, 44 (62%) were cared for by hospitalistHCC teams and 27 (38%) were cared for by control hospitalists. Patient satisfaction with the clarity of the verbal and written discharge instructions and overall satisfaction with hospital discharge was similar between the 2 groups (see Table 4).
HospitalistHCC Team (n = 44) | Control (n = 27) | P value | |
---|---|---|---|
Mean score (SD)* | |||
| |||
Clarity of verbal discharge instructions | 8.86 ( 2.31) | 8.44 ( 2.63) | 0.52 |
Clarity of written discharge instructions | 8.95 ( 2.30) | 8.93 ( 2.54) | 0.78 |
Overall satisfaction with hospital discharge | 8.57 ( 2.42) | 8.37 ( 2.90) | 0.94 |
The unadjusted mean LOS for patients cared for by hospitalistHCC teams was 4.70 4.15 days compared with 5.07 3.99 days for patients cared for by control hospitalists (P = .005; see Table 5). The unadjusted mean cost for patients cared for by hospitalistHCC teams was $10,052.96 $11,708.73 compared with $11,703.19 $20,455.78 for patients cared for by control hospitalists (P = .008). In multivariate analysis using age, sex, ethnicity, payer type, and DRG weight as independent variables and adjusting for physician clustering, LOS remained lower for patients cared for by hospitalistHCC teams; however, this result was not statistically significant (0.28 days, P = .17). Similar multivariate regression analysis showed a trend toward lower cost for patients cared for by the hospitalistHCC teams (585.62, P = .15).
Unadjusted mean (SD) | P value for unadjusted difference* | Adjusted difference with hospitalistHCC team | P value for adjusted difference | |
---|---|---|---|---|
| ||||
Length of stay | ||||
HospitalistHCC teams | 4.70 (4.15) | .005 | 0.28 | .17 |
Control hospitalists | 5.07 (3.99) | |||
Cost | ||||
HospitalistHCC teams | 10,052.96 (11,708.73) | .008 | 585.62 | .15 |
Control hospitalists | 11,703.19 (20,455.78) |
DISCUSSION
Our study found that hospitalists working in a team approach with an HCC rated the efficiency of their daily work and their job satisfaction significantly higher than did control hospitalists. Specific areas of improved efficiency included communication activities and activities related to hospital discharge. A prior study conducted by our group found that hospitalists spend a lot of time on indirect patient care activities such as communication and activities related to the discharge process, while spending relatively little time on direct patient care.8 Improving the efficiency of indirect patient care activities of hospitalists is likely to improve their job satisfaction. The importance of improving hospitalist workload and job satisfaction is underscored by the relatively high number of hospitalists at risk for burnout9 and the growing concern about daily workload among hospital medicine group leaders.7
Patient satisfaction was not significantly affected by the use of the hospitalistHCC team in our study. A priori, we postulated that patient satisfaction with the discharge process might improve with the use of the hospitalistHCC team. We therefore limited survey questions to assessing only satisfaction with hospital discharge rather than other aspects of patient hospital care. A recent study reported that patients rated the quality of discharge instructions significantly lower than they rated the overall quality of their hospital stay.10 However, the patients in our study gave high ratings to both discharge instructions and overall satisfaction with hospital discharge. This may explain why we were unable to detect a difference. Our study was limited by the relatively small number of patients we were able to contact to assess satisfaction. Previous studies evaluating the impact of care coordinators either did not assess patient satisfaction with discharge5 or found no difference in satisfaction with hospital discharge.6
Although our study did not find a difference in patient satisfaction with the discharge process, we believe the hospitalistHCC model has the potential to complement efforts to reduce the risk of adverse events as patients transition out of the hospital. It has been reported that 12% of patients have a preventable or ameliorable adverse event in the period immediately following hospital discharge.11, 12 Although Forster et al. did not find a reduction in the risk of adverse events with the addition of a clinical coordinator to a general medical team, they noted incongruence between the coordinator's role and the outcomes measured.6 Similarly, we would need to modify the role of the HCC from a position designed mainly to improve efficiency to one that complements efforts to improve the quality of the discharge process. Possible ways to enhance the HCC role in this regard include increasing the emphasis on and training in patient education skills. Several recently published articles have emphasized the need to redesign the discharge process in an effort to reduce the risk of adverse events following hospital discharge.1315 A modified HCC role might be an essential feature of a redesigned multidisciplinary discharge process.
We were unable to demonstrate improved efficiency for the hospital. Although LOS and cost were lower for patients cared for by the hospitalistHCC teams, the difference was not statistically significant. One possible explanation for why we did not observe a larger reduction in LOS is that our hospitalist service had a lower‐than‐average patient volume during the study period. The lower volume mirrored an unanticipated dip in hospital volume during the same period. Specifically, our service normally discharges an average of 338 patients per month, but during the study period we discharged an average of 235 patients per month. A potential LOS and cost benefit may have been attenuated by the relatively low volume, as hospitalists had ample time to dedicate to communication and coordination of discharge plans.
Our study had several limitations. It was conducted on a nonteaching hospitalist service at a single site. Hospitalist practices vary widely in their staffing and scheduling models. As previously mentioned, we were only able to perform patient satisfaction surveys during the second half of the study period. In addition, hospitalistHCC team patients made up a larger percentage of the patient survey responses (62%) than did control hospitalist patients (38%). This may have affected our ability to detect differences in satisfaction with the hospital discharge process. As also previously noted, our patient volume was lower than normal during the study period. We believe that a higher volume would have magnified differences in hospitalists' perceived efficiency and perhaps resulted in significant improvements in LOS and cost. Finally, the hospital provided funding for only a 12‐week study. This limited our sample size and the power of the study to detect important differences. It is possible that a larger sample size and/or longer study period may have been able to demonstrate a statistically significant improvement in LOS and cost.
Our findings are of particular importance in light of the persistent concerns about hospitalist workload and job satisfaction. Although many hospitalists work with clinical coordinators and case managers, we believe that having the formal structure of a hospitalistcare coordinator team was the key element to improving hospitalist efficiency and satisfaction. We hope that our study is a precursor to research evaluating models of delivering hospital care and their impact on hospitalist work experience, hospital efficiency, and patient outcomes.
Many hospitalists work with clinical coordinators and case managers.13 The descriptions of these roles often overlap4 and commonly include activities such as obtaining medical records, expediting tests and procedures, coordinating the plan of care with other health care providers, assessing postdischarge needs, completing discharge paperwork, and arranging follow‐up visits.2, 5, 6 Despite the potential to improve patient care and hospital efficiency, few studies have formally evaluated the impact of these roles. Moher et al. found that adding a clinical coordinator to a general medical team decreased length of stay (LOS) and improved patient satisfaction.5 However, this study was conducted at a time when the LOS was routinely longer than it is today. Forster et al. found that adding a clinical coordinator to a general medical team resulted in improved patient satisfaction but did not reduce length of stay or risk of adverse events occurring following hospital discharge.6 Both these studies evaluated the impact of adding a clinical coordinator to resident‐covered medical teams. Yet many hospitalists deliver care without residents, limiting the generalizability of the findings from these studies.
To date, no studies have evaluated the impact of clinical coordinators, case managers, or other nonphysician providers on the hospitalist work experience. This is surprising, as hospital medicine group leaders list daily workload and work hours among their top concerns.7 Clinical coordinators have the potential to improve patient care and hospital efficiency while simultaneously improving the experience of the hospitalists with whom they work. We conducted this study to evaluate the impact of a hospitalistcare coordinator team on hospitalist work experience, patient satisfaction, and hospital efficiency.
METHODS
Setting
The study was conducted on the hospitalist service at Northwestern Memorial Hospital (NMH), a 753‐bed hospital in Chicago, Illinois. The hospitalist service is staffed by 5 hospitalists on duty at a time. Hospitalists work without residents and are on service for 7 consecutive days, usually followed by 7 consecutive days off. Daytime admissions are distributed among all hospitalists on duty in a consecutive fashion. A night float hospitalist performs admissions and all cross‐cover activities from 7:00 PM until 7:00 AM. Nighttime admissions are distributed to day hospitalists based on each hospitalist's daily census.
Study Design
Funding was provided by the hospital for a 12‐week study, and hospital administrative leaders collaborated as part of the research team. During each of the 12 weeks from September 2006 through November 2006, half the hospitalists on duty each week were randomly assigned to work with a hospitalist care coordinator (HCC) in a team approach. Hospitalists not assigned to work with a hospitalist care coordinator continued to work in their usual fashion, which included working with unit‐based care coordinators (UCCs). UCCs follow all patients on a 30‐bed medicine unit but generally do not round with physicians. HCCs performed all the activities that UCCs perform but also performed additional key activities (see Table 1) and worked in a team approach with their hospitalist. Unit‐based social workers and discharge planners were available for all hospitalists during the study. During each day patients were admitted consecutively to one of the hospitalists on service, regardless of their assignment to work with or without an HCC. Similarly, night admissions were distributed to hospitalists without regard to their assignment to work with or without an HCC.
Activity type | Activities performed by both UCCs and HCCs | Additional activities performed by HCCs only |
---|---|---|
Care coordination | Document the interdisciplinary plan of care | Ensure collaboration in formulating the plan of care |
Obtain outside medical records | ||
Inform staff nurse of stat orders during rounds | ||
Obtain certain test results (eg, preliminary echo reports) | ||
Patient and family needs | Address patient and family concerns | Proactively identify and address patient and family concerns |
Schedule family meetings | ||
Efficiency of care delivery | Remediate barriers that impede plan of care and/or discharge | Identify barriers that impede plan of care |
Identify and document avoidable days | Ensure tests are scheduled | |
Discharge process | Coordinate discharge plans with social work and discharge planner | Schedule and confirm follow‐up appointments |
Initiate discharge instructions | ||
Write discharge prescriptionsverified and signed by physician | ||
Review discharge instructions with patient and/or family |
HospitalistCare Coordinator Team
Four HCCs were used in this study. All 4 were registered nurses with specialized training in case management. Prior to the start of the study, the investigators held meetings with hospitalists and the HCCs to describe this new role, the work flow for the hospitalistcare coordinator team, and work activities appropriate for the HCC. Activities related to the discharge process were emphasized as a key feature of the HCC role. Hospitalists and HCCs were instructed to round together as a team each morning. They were advised to collaborate on the daily plan of care and assign specific activities for each to accomplish. During the study weekly meetings were held with the HCCs, the hospitalists with whom they were finishing the week, and the hospitalists with whom they were scheduled to work during the upcoming week. The purpose of these meetings was to ensure that the work flow and work activities were optimal.
Outcome Measures
At the completion of each week, all hospitalists on service were given an anonymous Web‐based survey designed to assess their satisfaction and perceived work efficiency. Hospitalists were asked to rate the efficiency of various work activities during the preceding week on a 5 point Likert scale (1 = very inefficient, 2 = somewhat inefficient, 3 = neutral, 4 = somewhat efficient, 5 = very efficient). Hospitalists who had worked with an HCC were also asked whether they thought working with an HCC improved their efficiency and increased their job satisfaction. We postulated that patient satisfaction with the discharge process might improve with use of the hospitalistHCC team. Therefore, patient satisfaction was assessed by telephone interviews conducted 714 days after discharge. Because of resource limitations, we were only able to interview patient during the second half of the study. Patients were asked to rate their satisfaction with the clarity of verbal and written discharge instructions as well as their overall satisfaction with hospital discharge using a 10‐point Likert scale (from 1 = least satisfied to 10 = most satisfied). Hospital databases provided information on patient demographics, LOS, and cost.
Data Analysis
All analyses were conducted using Stata version 9.0 (StataCorp LP, College Station, TX). Patient characteristics were compared using chi‐square and t tests. Responses to the hospitalist survey for the weeks when they worked independently and the weeks when they worked as a hospitalistHCC team were compared using the Wilcoxon rank sum test. To adjust for the clustering of responses by physicians (ie, individual physicians completed more than 1 survey), we used linear regression and the cluster option. The results were very similar, and only the P values from the Wilcoxon rank sum test are presented. Unadjusted LOS and cost were compared using the Wilcoxon rank sum test. We also conducted multivariate linear regressions using log‐transformed LOS and log‐transformed cost as dependent variables. The independent variable was the team type (whether patients were cared for by a hospitalistHCC team or a hospitalist working independently); age, sex, ethnicity, payer type, and diagnosis‐related group (DRG) weight were included as covariates, and P values were adjusted for physician clustering. We hypothesized a priori that the HCC would have no effect on the LOS of or cost for patients whose hospitalizations were very short. We therefore conducted secondary analyses in which we eliminated patients with an LOS of 1 day or less.
RESULTS
There were 356 patients cared for by hospitalistHCC teams and 337 patients cared for by control hospitalists. Of the 60 weeks of hospitalist service of the study, hospitalistHCC teams accounted for 31 weeks (52%) and control hospitalists for 29 weeks (48%). Patients cared for by the hospitalistHCC teams were similar in age, sex, ethnicity, payer type, and DRG weight to those cared for by control hospitalists (see Table 2).
HospitalistHCC team | Control | P value | |
---|---|---|---|
n (%) or mean SD | |||
Patients, n (%) | 356 (51.4%) | 337 (48.6%) | |
Age (years) | 59.3 18.6 | 59.0 20.1 | .86 |
Women (%) | 190 (53.4%) | 192 (57.0%) | .34 |
Ethnicity | .74 | ||
White | 182 (51.1%) | 174 (51.6%) | |
Black | 111 (31.2%) | 114 (33.8%) | |
Hispanic | 23 (6.5%) | 21 (6.2%) | |
Asian | 5 (1.4%) | 4 (1.2%) | |
Other | 35 (9.8%) | 24 (7.1%) | |
Payer | .47 | ||
Medicare | 177 (49.7%) | 168 (49.9%) | |
Private | 76 (21.4%) | 69 (20.5%) | |
Medicaid | 43 (12.1%) | 44 (13.1%) | |
Capitated | 43 (12.1%) | 31 (9.2%) | |
Other | 17 (4.8%) | 25 (7.4%) | |
Diagnosis‐related group weight | 1.1 0.8 | 1.2 0.8 | .31 |
Sixty surveys were completed by hospitalists at the end of their week on service (response rate 100%). Of the 31 responses from hospitalists completing a hospitalistHCC team week, 28 (90%) reported that working with an HCC improved their efficiency and 28 (90%) that working with an HCC improved their job satisfaction. The hospitalists indicated that working with an HCC significantly improved the efficiency of most of their activities (see Table 3). Specifically, activities related to communication with nurses and patients and activities involving discharge planning and execution were improved with the use of an HCC. As would be expected, certain other activities did not improve. For example, there were no differences between the groups in the perceived efficiency of performing histories and physicals or placing admission orders. For activities that were significantly different, the Wilcoxon rank sum test and linear regression analysis adjusting for physician clustering showed identical results.
HospitalistHCC Team | Control | P value | |
---|---|---|---|
Mean score SD* | |||
| |||
Performing histories and physicals | 3.94 0.77 | 3.93 0.84 | .98 |
Performing medication reconciliation | 3.35 1.08 | 2.03 1.18 | < .001 |
Placing admission orders | 3.94 0.73 | 4.00 0.87 | .57 |
Communicating with nurses | 4.45 0.68 | 3.14 1.09 | < .001 |
Communicating with consultants | 3.65 0.75 | 3.34 1.04 | .25 |
Communicating with patients | 4.42 0.62 | 3.62 1.01 | < .001 |
Communicating with families | 4.32 0.70 | 2.89 1.14 | < .001 |
Coordinating discharge plans | 4.74 0.51 | 2.76 1.18 | < .001 |
Making/updating sign‐out | 3.55 0.72 | 3.49 0.83 | .55 |
Making discharge instructions | 4.29 0.74 | 3.10 1.01 | < .001 |
Going over discharge instructions | 4.48 0.57 | 2.76 1.15 | < .001 |
Writing discharge prescriptions | 3.87 0.67 | 2.52 1.21 | < .001 |
Arranging follow‐up appointments | 4.19 0.75 | 2.03 1.09 | < .001 |
Seventy‐one of 196 eligible patients (36%) completed the discharge satisfaction interview. Of the 71 patients interviewed, 44 (62%) were cared for by hospitalistHCC teams and 27 (38%) were cared for by control hospitalists. Patient satisfaction with the clarity of the verbal and written discharge instructions and overall satisfaction with hospital discharge was similar between the 2 groups (see Table 4).
HospitalistHCC Team (n = 44) | Control (n = 27) | P value | |
---|---|---|---|
Mean score (SD)* | |||
| |||
Clarity of verbal discharge instructions | 8.86 ( 2.31) | 8.44 ( 2.63) | 0.52 |
Clarity of written discharge instructions | 8.95 ( 2.30) | 8.93 ( 2.54) | 0.78 |
Overall satisfaction with hospital discharge | 8.57 ( 2.42) | 8.37 ( 2.90) | 0.94 |
The unadjusted mean LOS for patients cared for by hospitalistHCC teams was 4.70 4.15 days compared with 5.07 3.99 days for patients cared for by control hospitalists (P = .005; see Table 5). The unadjusted mean cost for patients cared for by hospitalistHCC teams was $10,052.96 $11,708.73 compared with $11,703.19 $20,455.78 for patients cared for by control hospitalists (P = .008). In multivariate analysis using age, sex, ethnicity, payer type, and DRG weight as independent variables and adjusting for physician clustering, LOS remained lower for patients cared for by hospitalistHCC teams; however, this result was not statistically significant (0.28 days, P = .17). Similar multivariate regression analysis showed a trend toward lower cost for patients cared for by the hospitalistHCC teams (585.62, P = .15).
Unadjusted mean (SD) | P value for unadjusted difference* | Adjusted difference with hospitalistHCC team | P value for adjusted difference | |
---|---|---|---|---|
| ||||
Length of stay | ||||
HospitalistHCC teams | 4.70 (4.15) | .005 | 0.28 | .17 |
Control hospitalists | 5.07 (3.99) | |||
Cost | ||||
HospitalistHCC teams | 10,052.96 (11,708.73) | .008 | 585.62 | .15 |
Control hospitalists | 11,703.19 (20,455.78) |
DISCUSSION
Our study found that hospitalists working in a team approach with an HCC rated the efficiency of their daily work and their job satisfaction significantly higher than did control hospitalists. Specific areas of improved efficiency included communication activities and activities related to hospital discharge. A prior study conducted by our group found that hospitalists spend a lot of time on indirect patient care activities such as communication and activities related to the discharge process, while spending relatively little time on direct patient care.8 Improving the efficiency of indirect patient care activities of hospitalists is likely to improve their job satisfaction. The importance of improving hospitalist workload and job satisfaction is underscored by the relatively high number of hospitalists at risk for burnout9 and the growing concern about daily workload among hospital medicine group leaders.7
Patient satisfaction was not significantly affected by the use of the hospitalistHCC team in our study. A priori, we postulated that patient satisfaction with the discharge process might improve with the use of the hospitalistHCC team. We therefore limited survey questions to assessing only satisfaction with hospital discharge rather than other aspects of patient hospital care. A recent study reported that patients rated the quality of discharge instructions significantly lower than they rated the overall quality of their hospital stay.10 However, the patients in our study gave high ratings to both discharge instructions and overall satisfaction with hospital discharge. This may explain why we were unable to detect a difference. Our study was limited by the relatively small number of patients we were able to contact to assess satisfaction. Previous studies evaluating the impact of care coordinators either did not assess patient satisfaction with discharge5 or found no difference in satisfaction with hospital discharge.6
Although our study did not find a difference in patient satisfaction with the discharge process, we believe the hospitalistHCC model has the potential to complement efforts to reduce the risk of adverse events as patients transition out of the hospital. It has been reported that 12% of patients have a preventable or ameliorable adverse event in the period immediately following hospital discharge.11, 12 Although Forster et al. did not find a reduction in the risk of adverse events with the addition of a clinical coordinator to a general medical team, they noted incongruence between the coordinator's role and the outcomes measured.6 Similarly, we would need to modify the role of the HCC from a position designed mainly to improve efficiency to one that complements efforts to improve the quality of the discharge process. Possible ways to enhance the HCC role in this regard include increasing the emphasis on and training in patient education skills. Several recently published articles have emphasized the need to redesign the discharge process in an effort to reduce the risk of adverse events following hospital discharge.1315 A modified HCC role might be an essential feature of a redesigned multidisciplinary discharge process.
We were unable to demonstrate improved efficiency for the hospital. Although LOS and cost were lower for patients cared for by the hospitalistHCC teams, the difference was not statistically significant. One possible explanation for why we did not observe a larger reduction in LOS is that our hospitalist service had a lower‐than‐average patient volume during the study period. The lower volume mirrored an unanticipated dip in hospital volume during the same period. Specifically, our service normally discharges an average of 338 patients per month, but during the study period we discharged an average of 235 patients per month. A potential LOS and cost benefit may have been attenuated by the relatively low volume, as hospitalists had ample time to dedicate to communication and coordination of discharge plans.
Our study had several limitations. It was conducted on a nonteaching hospitalist service at a single site. Hospitalist practices vary widely in their staffing and scheduling models. As previously mentioned, we were only able to perform patient satisfaction surveys during the second half of the study period. In addition, hospitalistHCC team patients made up a larger percentage of the patient survey responses (62%) than did control hospitalist patients (38%). This may have affected our ability to detect differences in satisfaction with the hospital discharge process. As also previously noted, our patient volume was lower than normal during the study period. We believe that a higher volume would have magnified differences in hospitalists' perceived efficiency and perhaps resulted in significant improvements in LOS and cost. Finally, the hospital provided funding for only a 12‐week study. This limited our sample size and the power of the study to detect important differences. It is possible that a larger sample size and/or longer study period may have been able to demonstrate a statistically significant improvement in LOS and cost.
Our findings are of particular importance in light of the persistent concerns about hospitalist workload and job satisfaction. Although many hospitalists work with clinical coordinators and case managers, we believe that having the formal structure of a hospitalistcare coordinator team was the key element to improving hospitalist efficiency and satisfaction. We hope that our study is a precursor to research evaluating models of delivering hospital care and their impact on hospitalist work experience, hospital efficiency, and patient outcomes.
- Innovations in the management of hospitalized patients.Nurse Pract.2006;Suppl:2–3. .
- Hospitalists and case managers: the perfect partnership.Lippincotts Case Manag.2004;9:280–286. , .
- Productive interdisciplinary team relationships: the hospitalist and the case manager.Lippincotts Case Manag.2006;11:160–164. , .
- Care coordinator—blending roles to improve patient outcomes.J Nurs Manage.1997;28:49–52. , , .
- Effects of a medical team coordinator on length of hospital stay.Can Med Assoc J.1992;146:511–515. , , , .
- Effect of a nurse team coordinator on outcomes for hospitalized medicine patients.Am J Med.2005;118:1148–1153. , , , et al.
- Society of Hospital Medicine. Available at: http://www.hospitalmedicine.org/Content/NavigationMenu/ResourceCenter/Surveys/Surveys1.htm. Accessed April 2,2007.
- How hospitalists spend their time: insights on efficiency and safety.J Hosp Med.2006;1:88–93. , , .
- Characteristics and work experiences of hospitalists in the United States.Arch Intern Med.2001;161:851–858. , , , , .
- Patient perception of quality in discharge instruction.Patient Educ Couns.2005;59:56–68. , , , , , .
- Adverse events among medical patient after hospital discharge.Can Med Assoc J.2004;170:345–349. , , , et al.
- The incidence and severity of adverse events affecting patients after discharge from the hospital.Ann Intern Med.2003;138:161–167. , , , , .
- Lost in transition: challenges and opportunities for improving the quality of transitional care.Ann Intern Med.2004;141:533–536. , .
- Transition of care for hospitalized elderly patients—development of a discharge checklist for hospitalists.J Hosp Med.2006;1:354–360. , , , et al.
- Re‐engineering the hospital discharge—an example of a multifaceted process evaluation. Advances in Patient Safety: From Research to Implementation. Vol.2,Concepts and Methodology. AHRQ publication 05‐0021‐2.Rockville, MD:AHRQ;2005. p379–394. , , , et al.
- Innovations in the management of hospitalized patients.Nurse Pract.2006;Suppl:2–3. .
- Hospitalists and case managers: the perfect partnership.Lippincotts Case Manag.2004;9:280–286. , .
- Productive interdisciplinary team relationships: the hospitalist and the case manager.Lippincotts Case Manag.2006;11:160–164. , .
- Care coordinator—blending roles to improve patient outcomes.J Nurs Manage.1997;28:49–52. , , .
- Effects of a medical team coordinator on length of hospital stay.Can Med Assoc J.1992;146:511–515. , , , .
- Effect of a nurse team coordinator on outcomes for hospitalized medicine patients.Am J Med.2005;118:1148–1153. , , , et al.
- Society of Hospital Medicine. Available at: http://www.hospitalmedicine.org/Content/NavigationMenu/ResourceCenter/Surveys/Surveys1.htm. Accessed April 2,2007.
- How hospitalists spend their time: insights on efficiency and safety.J Hosp Med.2006;1:88–93. , , .
- Characteristics and work experiences of hospitalists in the United States.Arch Intern Med.2001;161:851–858. , , , , .
- Patient perception of quality in discharge instruction.Patient Educ Couns.2005;59:56–68. , , , , , .
- Adverse events among medical patient after hospital discharge.Can Med Assoc J.2004;170:345–349. , , , et al.
- The incidence and severity of adverse events affecting patients after discharge from the hospital.Ann Intern Med.2003;138:161–167. , , , , .
- Lost in transition: challenges and opportunities for improving the quality of transitional care.Ann Intern Med.2004;141:533–536. , .
- Transition of care for hospitalized elderly patients—development of a discharge checklist for hospitalists.J Hosp Med.2006;1:354–360. , , , et al.
- Re‐engineering the hospital discharge—an example of a multifaceted process evaluation. Advances in Patient Safety: From Research to Implementation. Vol.2,Concepts and Methodology. AHRQ publication 05‐0021‐2.Rockville, MD:AHRQ;2005. p379–394. , , , et al.
Copyright © 2008 Society of Hospital Medicine
Inappropriate Medication Use in Older Adults
Medications are central to managing the health of older patients. In 2006, more than 93% of adults 65 years or older reported taking at least 1 medication in the last week, 58% reported taking 5 or more medications, and 18% reported taking 10 or more.1 Medication use by older adults will likely increase further as the U.S. population ages, new drugs are developed, and new therapeutic and preventive uses for medications are discovered.2
Older patients, especially those who are chronically frail or acutely ill, may require special consideration when making prescribing decisions because of age‐related changes in the metabolism and clearance of medications and enhanced pharmacodynamic sensitivities.3 Thus, panels of experts in pharmacology and geriatrics have compiled lists of medications to avoid prescribing for patients 65 years of age or older. The most commonly used list is the Beers criteria, which were introduced in 1991 to serve researchers evaluating prescribing quality in nursing homes. The Beers criteria were updated in 1997 and again in 2003 to include 48 potentially inappropriate medications (PIMs) for which, according to the consensus panel, there are more effective or safer alternatives for older patients.4
Numerous studies in the last 15 years have found that PIMs continue to be used in 12% to 40% of older patients in community and nursing home settings.5 To address the continued use of PIMs, the Centers for Medicare and Medicaid Services incorporated the Beers criteria into federal safety regulations for long‐term care facilities in 1999.6 In 2006, the prescription rate of PIMs was introduced as a Health Plan and Employer Data and Information Set (HEDIS) quality measure for managed care plans.7 Despite adoption of the Beers criteria to monitor prescribing quality and safety in nursing homes and outpatient settings, there has been considerably less study of potentially inappropriate medication use in hospitalized patients.
In this issue of the Journal of Hospital Medicine, Rothberg and colleagues analyzed administrative data from nearly 400 hospitals across the United States and found that nearly half of all older patients hospitalized for 7 common conditions were prescribed at least 1 PIM.8 Thus, the incidence of PIM use in hospitalized older patients far exceeded that reported in most studies of community‐dwelling or nursing home patients. Most notable, however, was the variability found in prescribing rates based on a number of physician and hospital characteristics. For example, although hospitalists and geriatricians were found to be less likely to prescribe PIMs than cardiologists and general internists, among high‐volume cardiologists and internists, PIM prescribing rates ranged widely, from 0% to more than 90%. Similarly, hospitalwide prescribing rates varied by geographic region, and there were 7 hospitals in which not a single PIM was reportedly prescribed.
These findings raise three questions and bring to mind parallels with efforts to control inappropriate antimicrobial use. First question: Can inpatient use of PIMs truly be higher than outpatient use? Yes. The finding that more hospitalized patients are prescribed PIMs than ambulatory patients has face validity for several reasons. First, patients admitted for an acute hospitalization may have more comorbid diseases and take more medications than community‐dwelling older adults. Second, new medications are typically added to treat acutely ill patients on hospitalization. Third, previous studies estimating outpatient PIM use have typically used more narrowly defined lists of PIMs and have not captured over‐the‐counter use of PIMs, particularly antihistamines.9 Diphenhydramine alone accounted for 9% of PIM use in Rothberg's study. Finally, as Rothberg and colleagues point out, this study was limited to certain diagnoses such as acute myocardial infarction that may have protocol‐driven prescribing, which includes PIMs, that may be used only a single time such as promethazine.
Second question: Can PIM prescribing truly be so variable across regions, specialties, and individual hospitals and physicians? Yes. Using multivariable modeling, Rothberg and colleagues controlled for many patient, hospital, and physician characteristics and still found significant variation. John Wennberg and others have documented similar variations for a host of medical treatments; but although variation is interesting, it is unwarranted variation that matters for improving health care quality.10 It is not clear how much of the variation in prescribing rates of PIMs is unwarranted.
Some degree of variation in PIM prescribing rates is certainly acceptable. As the creators of the Beers criteria acknowledge, these medications are deemed only potentially inappropriate, and individual treatment decisions should be tailored to individual patients. However, others have taken the term potentially inappropriate one step further by recategorizing Beers medications as always avoid medications, rarely acceptable medications, and medications that indeed have some indications for use in older adults.8
Variation in prescribing practice may also be acceptable when there is not a clear consensus on the superiority of one practice over another. Indeed, the evidence that PIM prescribing causes large numbers of clinically significant adverse drug events and patient harm is weak and largely based on observational studies with inconsistent results. Although some studies demonstrated an epidemiological association between Beers criteria medications and general adverse outcomes (eg, hospitalizations),11 other studies did not.12 A recent systematic review concluded that Beers criteria medications were associated with some adverse health effects, but the studies analyzed were too heterogeneous to support formal meta‐analysis.13 Thus, variability in prescribing rates of Beers medications may simply reflect individual clinical judgment in the absence of conclusive outcomes data.
Third question: Can hospitalists use the findings of Rothberg and colleagues to improve the quality of medication prescribing for older adults in their institutions? Maybe. But hospitalists wishing to reduce PIM use in their institutions should draw lessons from other efforts to modify physician‐prescribing practice such as efforts to reduce inappropriate antimicrobial use. Although national data draw attention to the high frequency of potentially inappropriate medication use in hospitalized patients, the large variation in use across hospitals confirms the need for monitoring in individual facilities. For example, the National Healthcare Safety Network provides national benchmarks of antimicrobial use and resistance, but individual hospitals monitor antibiotic use and resistance in their own institutions to tailor local efforts to improve antimicrobial prescribing.14
Also, initiating a quality improvement effort targeting all 48 Beers criteria medications may be an inefficient use of resources. Using such a composite measure obscures the contribution of the component medications, each of which possesses unique and sometimes controversial profiles of efficacy and harm for older patients. Instead, a targeted intervention addressing the most commonly prescribed Beers medications that have widely accepted alternatives could be more practical. For instance, many antibiotic management programs focus on replacing a popular, extended‐spectrum antimicrobial with a narrow‐spectrum agent as soon as microbiological susceptibly results are available.
Propoxephene is a PIM that may be an attractive target for intervention. Propoxephene was the third most commonly prescribed PIM identified by Rothberg and colleagues, but meta‐analyses of controlled trials have concluded that propoxephene provides inferior analgesia for acute pain compared with that provided by other opioids with similar side effects, and has more adverse effects than nonopioid analgesics.15 Indeed, Rothberg found that just 3 of 48 PIMs (promethazine, diphenhydramine, and propoxyphene), each of which has viable alternative agents, accounted for approximately a quarter of all potentially inappropriate prescribing.
However, not all of the 48 Beers medications have alternatives with strong evidence of superiority. The Beers list includes medications (eg, amiodarone) that may not have equivalent alternative agents. On the other hand, some Beers medications have largely been supplanted (eg, ticlopidine or tripelennamine), and identifying these medications may be an inefficient use of scarce patient safety resources. As with antimicrobial stewardship programs, local surveillance of PIM use should be combined with local consensus on appropriate alternatives to target PIM interventions.
Of course, once specific PIM use is targeted for improvement, a specific intervention must be implemented. Only a handful of studies have examined the effectiveness of interventions (eg, computerized pharmacy alerts) to reduce PIM use, and most of these have focused on the outpatient setting rather than hospitalized patients.3 One study that included hospitalized patients utilized a team approach (geriatricians, nurses, social workers, and pharmacists) and demonstrated a reduction in potentially inappropriate medication use but no reduction in adverse drug reactions during hospitalization.16 In light of the scarcity of controlled intervention trials to reduce PIM use, initiatives to reduce inappropriate antimicrobial prescribing may provide useful insights into the strengths and limitations of approaches such as clinician education, formulary restrictions, pharmacist review, and computer‐based monitoring.17
Finally, any intervention to reduce PIM use should have reasonable expectations. The Beers criteria were developed to improve the effectiveness of medication therapy for older adults as well as to prevent harm, but it is unlikely that reducing PIM use in hospitalized patients will result in improvements that could be measured easily during an initial hospitalization. If preventing drug‐induced harm during the hospitalization of older patients is the primary concern, a shift in focus is required. Safety efforts should be concentrated on identifying and mitigating the most common and severe adverse drug events, rather than focusing efforts on reducing the use of PIMs. National data demonstrate that a handful of drugsinsulin, warfarin, and digoxinmost commonly cause severe adverse events in older outpatients.18 Optimizing the management of these medications may be another approach for improving drug safety in hospitalized patients. Regardless of the focus of a drug safety intervention, the experience of infection control and hospital epidemiology programs suggests that success will require dedicated professionals and the commitment of resources to examine patterns of local use, implement interventions, and monitor outcomes.
Acknowledgements
The author thanks Carolyn Gould, MD, and Nadine Shehab, PharmD, of the Centers for Disease Control and Prevention for their insights and thoughtful comments.
- Slone Epidemiology Center. Patterns of medication use in the United States, 2006: a report from the Slone survey. Available at: http://www.bu.edu/slone/SloneSurvey/AnnualRpt/SloneSurveyWebReport2006.pdf. Accessed September 17,2007.
- Outpatient drug safety: new steps in an old direction.Pharmacoepidemiol Drug Saf.2007;16:160–165. , .
- Appropriate prescribing in elderly people: how well can it be measured and optimised?Lancet.2007;370:173–184. , , , et al.
- Updating the Beers criteria for potentially inappropriate medication use in older adults: results of a US consensus panel of experts.Arch Intern Med.2003;163:2716–2724. , , , , , .
- Randomized trial to improve prescribing safety in ambulatory elderly patients.JAm Geriatr Soc.2007;55:977–985. , , , et al.
- Centers for Medicare and Medicaid Services.Survey Protocol for Long‐Term Care Facilities, Vol.2004.1999.
- HEDIS® 2007 Final NDC Lists: Drugs to Be Avoided in the Elderly. Washington, DC: National Committee on Quality Assurance; 2007. Available at: http://web.ncqa.org/tabid/210/Default.aspx. Accessed September 17,2007.
- Potentially inappropriate medication use in hospitalized elders.J Hosp Med.2008;3:91–102. , , , et al.
- Potentially inappropriate medication use in the community‐dwelling elderly: findings from the 1996 medical expenditure panel survey.JAMA.2001;286:2823–2829. , , , et al.
- Unwarranted variations in healthcare delivery: implications for academic medical centers.BMJ.2002;325:961–964. .
- Hospitalization and death associated with potentially inappropriate medication prescriptions among elderly nursing home residents.Arch Intern Med.2005;165:68–74. , , , , .
- Can an algorithm for appropriate prescribing predict adverse drug events?Am J Manag Care.2005;11:145–151. , , , , , .
- Healthcare outcomes associated with Beers' criteria: a systematic review.Ann Pharmacother.2007;41:438–448. , .
- Surveillance of antimicrobial use and antimicrobial resistance in United States hospitals: project ICARE phase 2.Clin Infect Dis.1999;29:245–252. , , , et al.
- Systematic overview of co‐proxamol to assess analgesic effects of addition of dextropropoxyphene to parcetamol.BMJ.1997;315:1565–1571. , .
- Effects of geriatric evaluation and management on adverse drug reactions and suboptimal prescribing in the frail elderly.Am J Med.2004;116:394–401. , , , et al.
- Antimicrobial stewardship programs in health care systems.Clin Microbiol Rev.18;4:638–656. , .
- National surveillance of emergency department visits for outpatient adverse drug events.JAMA.2006;296:1858–1866. , , , , , .
Medications are central to managing the health of older patients. In 2006, more than 93% of adults 65 years or older reported taking at least 1 medication in the last week, 58% reported taking 5 or more medications, and 18% reported taking 10 or more.1 Medication use by older adults will likely increase further as the U.S. population ages, new drugs are developed, and new therapeutic and preventive uses for medications are discovered.2
Older patients, especially those who are chronically frail or acutely ill, may require special consideration when making prescribing decisions because of age‐related changes in the metabolism and clearance of medications and enhanced pharmacodynamic sensitivities.3 Thus, panels of experts in pharmacology and geriatrics have compiled lists of medications to avoid prescribing for patients 65 years of age or older. The most commonly used list is the Beers criteria, which were introduced in 1991 to serve researchers evaluating prescribing quality in nursing homes. The Beers criteria were updated in 1997 and again in 2003 to include 48 potentially inappropriate medications (PIMs) for which, according to the consensus panel, there are more effective or safer alternatives for older patients.4
Numerous studies in the last 15 years have found that PIMs continue to be used in 12% to 40% of older patients in community and nursing home settings.5 To address the continued use of PIMs, the Centers for Medicare and Medicaid Services incorporated the Beers criteria into federal safety regulations for long‐term care facilities in 1999.6 In 2006, the prescription rate of PIMs was introduced as a Health Plan and Employer Data and Information Set (HEDIS) quality measure for managed care plans.7 Despite adoption of the Beers criteria to monitor prescribing quality and safety in nursing homes and outpatient settings, there has been considerably less study of potentially inappropriate medication use in hospitalized patients.
In this issue of the Journal of Hospital Medicine, Rothberg and colleagues analyzed administrative data from nearly 400 hospitals across the United States and found that nearly half of all older patients hospitalized for 7 common conditions were prescribed at least 1 PIM.8 Thus, the incidence of PIM use in hospitalized older patients far exceeded that reported in most studies of community‐dwelling or nursing home patients. Most notable, however, was the variability found in prescribing rates based on a number of physician and hospital characteristics. For example, although hospitalists and geriatricians were found to be less likely to prescribe PIMs than cardiologists and general internists, among high‐volume cardiologists and internists, PIM prescribing rates ranged widely, from 0% to more than 90%. Similarly, hospitalwide prescribing rates varied by geographic region, and there were 7 hospitals in which not a single PIM was reportedly prescribed.
These findings raise three questions and bring to mind parallels with efforts to control inappropriate antimicrobial use. First question: Can inpatient use of PIMs truly be higher than outpatient use? Yes. The finding that more hospitalized patients are prescribed PIMs than ambulatory patients has face validity for several reasons. First, patients admitted for an acute hospitalization may have more comorbid diseases and take more medications than community‐dwelling older adults. Second, new medications are typically added to treat acutely ill patients on hospitalization. Third, previous studies estimating outpatient PIM use have typically used more narrowly defined lists of PIMs and have not captured over‐the‐counter use of PIMs, particularly antihistamines.9 Diphenhydramine alone accounted for 9% of PIM use in Rothberg's study. Finally, as Rothberg and colleagues point out, this study was limited to certain diagnoses such as acute myocardial infarction that may have protocol‐driven prescribing, which includes PIMs, that may be used only a single time such as promethazine.
Second question: Can PIM prescribing truly be so variable across regions, specialties, and individual hospitals and physicians? Yes. Using multivariable modeling, Rothberg and colleagues controlled for many patient, hospital, and physician characteristics and still found significant variation. John Wennberg and others have documented similar variations for a host of medical treatments; but although variation is interesting, it is unwarranted variation that matters for improving health care quality.10 It is not clear how much of the variation in prescribing rates of PIMs is unwarranted.
Some degree of variation in PIM prescribing rates is certainly acceptable. As the creators of the Beers criteria acknowledge, these medications are deemed only potentially inappropriate, and individual treatment decisions should be tailored to individual patients. However, others have taken the term potentially inappropriate one step further by recategorizing Beers medications as always avoid medications, rarely acceptable medications, and medications that indeed have some indications for use in older adults.8
Variation in prescribing practice may also be acceptable when there is not a clear consensus on the superiority of one practice over another. Indeed, the evidence that PIM prescribing causes large numbers of clinically significant adverse drug events and patient harm is weak and largely based on observational studies with inconsistent results. Although some studies demonstrated an epidemiological association between Beers criteria medications and general adverse outcomes (eg, hospitalizations),11 other studies did not.12 A recent systematic review concluded that Beers criteria medications were associated with some adverse health effects, but the studies analyzed were too heterogeneous to support formal meta‐analysis.13 Thus, variability in prescribing rates of Beers medications may simply reflect individual clinical judgment in the absence of conclusive outcomes data.
Third question: Can hospitalists use the findings of Rothberg and colleagues to improve the quality of medication prescribing for older adults in their institutions? Maybe. But hospitalists wishing to reduce PIM use in their institutions should draw lessons from other efforts to modify physician‐prescribing practice such as efforts to reduce inappropriate antimicrobial use. Although national data draw attention to the high frequency of potentially inappropriate medication use in hospitalized patients, the large variation in use across hospitals confirms the need for monitoring in individual facilities. For example, the National Healthcare Safety Network provides national benchmarks of antimicrobial use and resistance, but individual hospitals monitor antibiotic use and resistance in their own institutions to tailor local efforts to improve antimicrobial prescribing.14
Also, initiating a quality improvement effort targeting all 48 Beers criteria medications may be an inefficient use of resources. Using such a composite measure obscures the contribution of the component medications, each of which possesses unique and sometimes controversial profiles of efficacy and harm for older patients. Instead, a targeted intervention addressing the most commonly prescribed Beers medications that have widely accepted alternatives could be more practical. For instance, many antibiotic management programs focus on replacing a popular, extended‐spectrum antimicrobial with a narrow‐spectrum agent as soon as microbiological susceptibly results are available.
Propoxephene is a PIM that may be an attractive target for intervention. Propoxephene was the third most commonly prescribed PIM identified by Rothberg and colleagues, but meta‐analyses of controlled trials have concluded that propoxephene provides inferior analgesia for acute pain compared with that provided by other opioids with similar side effects, and has more adverse effects than nonopioid analgesics.15 Indeed, Rothberg found that just 3 of 48 PIMs (promethazine, diphenhydramine, and propoxyphene), each of which has viable alternative agents, accounted for approximately a quarter of all potentially inappropriate prescribing.
However, not all of the 48 Beers medications have alternatives with strong evidence of superiority. The Beers list includes medications (eg, amiodarone) that may not have equivalent alternative agents. On the other hand, some Beers medications have largely been supplanted (eg, ticlopidine or tripelennamine), and identifying these medications may be an inefficient use of scarce patient safety resources. As with antimicrobial stewardship programs, local surveillance of PIM use should be combined with local consensus on appropriate alternatives to target PIM interventions.
Of course, once specific PIM use is targeted for improvement, a specific intervention must be implemented. Only a handful of studies have examined the effectiveness of interventions (eg, computerized pharmacy alerts) to reduce PIM use, and most of these have focused on the outpatient setting rather than hospitalized patients.3 One study that included hospitalized patients utilized a team approach (geriatricians, nurses, social workers, and pharmacists) and demonstrated a reduction in potentially inappropriate medication use but no reduction in adverse drug reactions during hospitalization.16 In light of the scarcity of controlled intervention trials to reduce PIM use, initiatives to reduce inappropriate antimicrobial prescribing may provide useful insights into the strengths and limitations of approaches such as clinician education, formulary restrictions, pharmacist review, and computer‐based monitoring.17
Finally, any intervention to reduce PIM use should have reasonable expectations. The Beers criteria were developed to improve the effectiveness of medication therapy for older adults as well as to prevent harm, but it is unlikely that reducing PIM use in hospitalized patients will result in improvements that could be measured easily during an initial hospitalization. If preventing drug‐induced harm during the hospitalization of older patients is the primary concern, a shift in focus is required. Safety efforts should be concentrated on identifying and mitigating the most common and severe adverse drug events, rather than focusing efforts on reducing the use of PIMs. National data demonstrate that a handful of drugsinsulin, warfarin, and digoxinmost commonly cause severe adverse events in older outpatients.18 Optimizing the management of these medications may be another approach for improving drug safety in hospitalized patients. Regardless of the focus of a drug safety intervention, the experience of infection control and hospital epidemiology programs suggests that success will require dedicated professionals and the commitment of resources to examine patterns of local use, implement interventions, and monitor outcomes.
Acknowledgements
The author thanks Carolyn Gould, MD, and Nadine Shehab, PharmD, of the Centers for Disease Control and Prevention for their insights and thoughtful comments.
Medications are central to managing the health of older patients. In 2006, more than 93% of adults 65 years or older reported taking at least 1 medication in the last week, 58% reported taking 5 or more medications, and 18% reported taking 10 or more.1 Medication use by older adults will likely increase further as the U.S. population ages, new drugs are developed, and new therapeutic and preventive uses for medications are discovered.2
Older patients, especially those who are chronically frail or acutely ill, may require special consideration when making prescribing decisions because of age‐related changes in the metabolism and clearance of medications and enhanced pharmacodynamic sensitivities.3 Thus, panels of experts in pharmacology and geriatrics have compiled lists of medications to avoid prescribing for patients 65 years of age or older. The most commonly used list is the Beers criteria, which were introduced in 1991 to serve researchers evaluating prescribing quality in nursing homes. The Beers criteria were updated in 1997 and again in 2003 to include 48 potentially inappropriate medications (PIMs) for which, according to the consensus panel, there are more effective or safer alternatives for older patients.4
Numerous studies in the last 15 years have found that PIMs continue to be used in 12% to 40% of older patients in community and nursing home settings.5 To address the continued use of PIMs, the Centers for Medicare and Medicaid Services incorporated the Beers criteria into federal safety regulations for long‐term care facilities in 1999.6 In 2006, the prescription rate of PIMs was introduced as a Health Plan and Employer Data and Information Set (HEDIS) quality measure for managed care plans.7 Despite adoption of the Beers criteria to monitor prescribing quality and safety in nursing homes and outpatient settings, there has been considerably less study of potentially inappropriate medication use in hospitalized patients.
In this issue of the Journal of Hospital Medicine, Rothberg and colleagues analyzed administrative data from nearly 400 hospitals across the United States and found that nearly half of all older patients hospitalized for 7 common conditions were prescribed at least 1 PIM.8 Thus, the incidence of PIM use in hospitalized older patients far exceeded that reported in most studies of community‐dwelling or nursing home patients. Most notable, however, was the variability found in prescribing rates based on a number of physician and hospital characteristics. For example, although hospitalists and geriatricians were found to be less likely to prescribe PIMs than cardiologists and general internists, among high‐volume cardiologists and internists, PIM prescribing rates ranged widely, from 0% to more than 90%. Similarly, hospitalwide prescribing rates varied by geographic region, and there were 7 hospitals in which not a single PIM was reportedly prescribed.
These findings raise three questions and bring to mind parallels with efforts to control inappropriate antimicrobial use. First question: Can inpatient use of PIMs truly be higher than outpatient use? Yes. The finding that more hospitalized patients are prescribed PIMs than ambulatory patients has face validity for several reasons. First, patients admitted for an acute hospitalization may have more comorbid diseases and take more medications than community‐dwelling older adults. Second, new medications are typically added to treat acutely ill patients on hospitalization. Third, previous studies estimating outpatient PIM use have typically used more narrowly defined lists of PIMs and have not captured over‐the‐counter use of PIMs, particularly antihistamines.9 Diphenhydramine alone accounted for 9% of PIM use in Rothberg's study. Finally, as Rothberg and colleagues point out, this study was limited to certain diagnoses such as acute myocardial infarction that may have protocol‐driven prescribing, which includes PIMs, that may be used only a single time such as promethazine.
Second question: Can PIM prescribing truly be so variable across regions, specialties, and individual hospitals and physicians? Yes. Using multivariable modeling, Rothberg and colleagues controlled for many patient, hospital, and physician characteristics and still found significant variation. John Wennberg and others have documented similar variations for a host of medical treatments; but although variation is interesting, it is unwarranted variation that matters for improving health care quality.10 It is not clear how much of the variation in prescribing rates of PIMs is unwarranted.
Some degree of variation in PIM prescribing rates is certainly acceptable. As the creators of the Beers criteria acknowledge, these medications are deemed only potentially inappropriate, and individual treatment decisions should be tailored to individual patients. However, others have taken the term potentially inappropriate one step further by recategorizing Beers medications as always avoid medications, rarely acceptable medications, and medications that indeed have some indications for use in older adults.8
Variation in prescribing practice may also be acceptable when there is not a clear consensus on the superiority of one practice over another. Indeed, the evidence that PIM prescribing causes large numbers of clinically significant adverse drug events and patient harm is weak and largely based on observational studies with inconsistent results. Although some studies demonstrated an epidemiological association between Beers criteria medications and general adverse outcomes (eg, hospitalizations),11 other studies did not.12 A recent systematic review concluded that Beers criteria medications were associated with some adverse health effects, but the studies analyzed were too heterogeneous to support formal meta‐analysis.13 Thus, variability in prescribing rates of Beers medications may simply reflect individual clinical judgment in the absence of conclusive outcomes data.
Third question: Can hospitalists use the findings of Rothberg and colleagues to improve the quality of medication prescribing for older adults in their institutions? Maybe. But hospitalists wishing to reduce PIM use in their institutions should draw lessons from other efforts to modify physician‐prescribing practice such as efforts to reduce inappropriate antimicrobial use. Although national data draw attention to the high frequency of potentially inappropriate medication use in hospitalized patients, the large variation in use across hospitals confirms the need for monitoring in individual facilities. For example, the National Healthcare Safety Network provides national benchmarks of antimicrobial use and resistance, but individual hospitals monitor antibiotic use and resistance in their own institutions to tailor local efforts to improve antimicrobial prescribing.14
Also, initiating a quality improvement effort targeting all 48 Beers criteria medications may be an inefficient use of resources. Using such a composite measure obscures the contribution of the component medications, each of which possesses unique and sometimes controversial profiles of efficacy and harm for older patients. Instead, a targeted intervention addressing the most commonly prescribed Beers medications that have widely accepted alternatives could be more practical. For instance, many antibiotic management programs focus on replacing a popular, extended‐spectrum antimicrobial with a narrow‐spectrum agent as soon as microbiological susceptibly results are available.
Propoxephene is a PIM that may be an attractive target for intervention. Propoxephene was the third most commonly prescribed PIM identified by Rothberg and colleagues, but meta‐analyses of controlled trials have concluded that propoxephene provides inferior analgesia for acute pain compared with that provided by other opioids with similar side effects, and has more adverse effects than nonopioid analgesics.15 Indeed, Rothberg found that just 3 of 48 PIMs (promethazine, diphenhydramine, and propoxyphene), each of which has viable alternative agents, accounted for approximately a quarter of all potentially inappropriate prescribing.
However, not all of the 48 Beers medications have alternatives with strong evidence of superiority. The Beers list includes medications (eg, amiodarone) that may not have equivalent alternative agents. On the other hand, some Beers medications have largely been supplanted (eg, ticlopidine or tripelennamine), and identifying these medications may be an inefficient use of scarce patient safety resources. As with antimicrobial stewardship programs, local surveillance of PIM use should be combined with local consensus on appropriate alternatives to target PIM interventions.
Of course, once specific PIM use is targeted for improvement, a specific intervention must be implemented. Only a handful of studies have examined the effectiveness of interventions (eg, computerized pharmacy alerts) to reduce PIM use, and most of these have focused on the outpatient setting rather than hospitalized patients.3 One study that included hospitalized patients utilized a team approach (geriatricians, nurses, social workers, and pharmacists) and demonstrated a reduction in potentially inappropriate medication use but no reduction in adverse drug reactions during hospitalization.16 In light of the scarcity of controlled intervention trials to reduce PIM use, initiatives to reduce inappropriate antimicrobial prescribing may provide useful insights into the strengths and limitations of approaches such as clinician education, formulary restrictions, pharmacist review, and computer‐based monitoring.17
Finally, any intervention to reduce PIM use should have reasonable expectations. The Beers criteria were developed to improve the effectiveness of medication therapy for older adults as well as to prevent harm, but it is unlikely that reducing PIM use in hospitalized patients will result in improvements that could be measured easily during an initial hospitalization. If preventing drug‐induced harm during the hospitalization of older patients is the primary concern, a shift in focus is required. Safety efforts should be concentrated on identifying and mitigating the most common and severe adverse drug events, rather than focusing efforts on reducing the use of PIMs. National data demonstrate that a handful of drugsinsulin, warfarin, and digoxinmost commonly cause severe adverse events in older outpatients.18 Optimizing the management of these medications may be another approach for improving drug safety in hospitalized patients. Regardless of the focus of a drug safety intervention, the experience of infection control and hospital epidemiology programs suggests that success will require dedicated professionals and the commitment of resources to examine patterns of local use, implement interventions, and monitor outcomes.
Acknowledgements
The author thanks Carolyn Gould, MD, and Nadine Shehab, PharmD, of the Centers for Disease Control and Prevention for their insights and thoughtful comments.
- Slone Epidemiology Center. Patterns of medication use in the United States, 2006: a report from the Slone survey. Available at: http://www.bu.edu/slone/SloneSurvey/AnnualRpt/SloneSurveyWebReport2006.pdf. Accessed September 17,2007.
- Outpatient drug safety: new steps in an old direction.Pharmacoepidemiol Drug Saf.2007;16:160–165. , .
- Appropriate prescribing in elderly people: how well can it be measured and optimised?Lancet.2007;370:173–184. , , , et al.
- Updating the Beers criteria for potentially inappropriate medication use in older adults: results of a US consensus panel of experts.Arch Intern Med.2003;163:2716–2724. , , , , , .
- Randomized trial to improve prescribing safety in ambulatory elderly patients.JAm Geriatr Soc.2007;55:977–985. , , , et al.
- Centers for Medicare and Medicaid Services.Survey Protocol for Long‐Term Care Facilities, Vol.2004.1999.
- HEDIS® 2007 Final NDC Lists: Drugs to Be Avoided in the Elderly. Washington, DC: National Committee on Quality Assurance; 2007. Available at: http://web.ncqa.org/tabid/210/Default.aspx. Accessed September 17,2007.
- Potentially inappropriate medication use in hospitalized elders.J Hosp Med.2008;3:91–102. , , , et al.
- Potentially inappropriate medication use in the community‐dwelling elderly: findings from the 1996 medical expenditure panel survey.JAMA.2001;286:2823–2829. , , , et al.
- Unwarranted variations in healthcare delivery: implications for academic medical centers.BMJ.2002;325:961–964. .
- Hospitalization and death associated with potentially inappropriate medication prescriptions among elderly nursing home residents.Arch Intern Med.2005;165:68–74. , , , , .
- Can an algorithm for appropriate prescribing predict adverse drug events?Am J Manag Care.2005;11:145–151. , , , , , .
- Healthcare outcomes associated with Beers' criteria: a systematic review.Ann Pharmacother.2007;41:438–448. , .
- Surveillance of antimicrobial use and antimicrobial resistance in United States hospitals: project ICARE phase 2.Clin Infect Dis.1999;29:245–252. , , , et al.
- Systematic overview of co‐proxamol to assess analgesic effects of addition of dextropropoxyphene to parcetamol.BMJ.1997;315:1565–1571. , .
- Effects of geriatric evaluation and management on adverse drug reactions and suboptimal prescribing in the frail elderly.Am J Med.2004;116:394–401. , , , et al.
- Antimicrobial stewardship programs in health care systems.Clin Microbiol Rev.18;4:638–656. , .
- National surveillance of emergency department visits for outpatient adverse drug events.JAMA.2006;296:1858–1866. , , , , , .
- Slone Epidemiology Center. Patterns of medication use in the United States, 2006: a report from the Slone survey. Available at: http://www.bu.edu/slone/SloneSurvey/AnnualRpt/SloneSurveyWebReport2006.pdf. Accessed September 17,2007.
- Outpatient drug safety: new steps in an old direction.Pharmacoepidemiol Drug Saf.2007;16:160–165. , .
- Appropriate prescribing in elderly people: how well can it be measured and optimised?Lancet.2007;370:173–184. , , , et al.
- Updating the Beers criteria for potentially inappropriate medication use in older adults: results of a US consensus panel of experts.Arch Intern Med.2003;163:2716–2724. , , , , , .
- Randomized trial to improve prescribing safety in ambulatory elderly patients.JAm Geriatr Soc.2007;55:977–985. , , , et al.
- Centers for Medicare and Medicaid Services.Survey Protocol for Long‐Term Care Facilities, Vol.2004.1999.
- HEDIS® 2007 Final NDC Lists: Drugs to Be Avoided in the Elderly. Washington, DC: National Committee on Quality Assurance; 2007. Available at: http://web.ncqa.org/tabid/210/Default.aspx. Accessed September 17,2007.
- Potentially inappropriate medication use in hospitalized elders.J Hosp Med.2008;3:91–102. , , , et al.
- Potentially inappropriate medication use in the community‐dwelling elderly: findings from the 1996 medical expenditure panel survey.JAMA.2001;286:2823–2829. , , , et al.
- Unwarranted variations in healthcare delivery: implications for academic medical centers.BMJ.2002;325:961–964. .
- Hospitalization and death associated with potentially inappropriate medication prescriptions among elderly nursing home residents.Arch Intern Med.2005;165:68–74. , , , , .
- Can an algorithm for appropriate prescribing predict adverse drug events?Am J Manag Care.2005;11:145–151. , , , , , .
- Healthcare outcomes associated with Beers' criteria: a systematic review.Ann Pharmacother.2007;41:438–448. , .
- Surveillance of antimicrobial use and antimicrobial resistance in United States hospitals: project ICARE phase 2.Clin Infect Dis.1999;29:245–252. , , , et al.
- Systematic overview of co‐proxamol to assess analgesic effects of addition of dextropropoxyphene to parcetamol.BMJ.1997;315:1565–1571. , .
- Effects of geriatric evaluation and management on adverse drug reactions and suboptimal prescribing in the frail elderly.Am J Med.2004;116:394–401. , , , et al.
- Antimicrobial stewardship programs in health care systems.Clin Microbiol Rev.18;4:638–656. , .
- National surveillance of emergency department visits for outpatient adverse drug events.JAMA.2006;296:1858–1866. , , , , , .
Tuberculosis: In and out of the airways
A 23‐year‐old Chinese woman presented with worsening exertional dyspnea. Her medical history was notable for pulmonary tuberculosis treated at the age of 16. Over the past 3 years, she reported progressive respiratory symptoms resulting in marked exercise intolerance. She denied any fevers, cough, or weight loss. On examination, she had right‐sided tracheal deviation but spoke comfortably. Her heart sounds were displaced and right‐sided breath sounds nearly absent. Chest x‐ray (Fig. 1) and subsequent CT revealed complete collapse of the right lung with associated hyperexpansion of the left lung and left‐to‐right mediastinal shift (Fig. 2, with an asterisk denoting the aortic arch; an arrow, the right main‐stem bronchus, which would soon terminate; and arrowheads, the collapsed right lung). No lung masses or effusions were noted; active TB had been ruled out with AFB sputums. Bronchoscopy revealed a fibrotic and stenotic right main‐stem bronchus (Fig. 3, with an asterisk denoting a patent left main‐stem bronchus and an arrow denoting a stenotic right main‐stem bronchus). Pulmonary manifestations of TB include parenchymal and endobronchial disease. Patients more likely to develop endobronchial disease include those with extensive pulmonary involvement, particularly cavitary lesions. Between 10% and 20% of patients with endobronchial disease will have normal CXRs, though CT scans may reveal endobronchial lesions or narrowing. Complications of endobronchial disease include obstruction, bronchiectasis, and tracheal or bronchial stenosis. Some airway obstructions may be associated with enlarging peribronchial nodes, which may erode into the airways as broncholiths. Steroids have been used to prevent long‐term complications, but their efficacy is still unclear. Repeated dilation, stenting, and resection all serve as management options for advanced endobronchial disease. In our patient, the extensive bronchial scarring and stenosis were most likely complications from past endobronchial infection. Unfortunately, attempts at balloon dilatation of her right main‐stem bronchus were unsuccessful, and she continues to have considerable exercise limitation. More prompt recognition of the disease may have allowed for an earlier and more successful intervention.
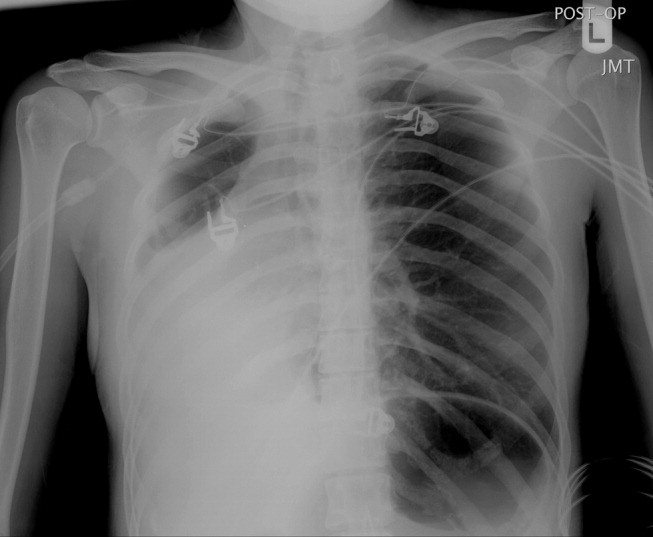
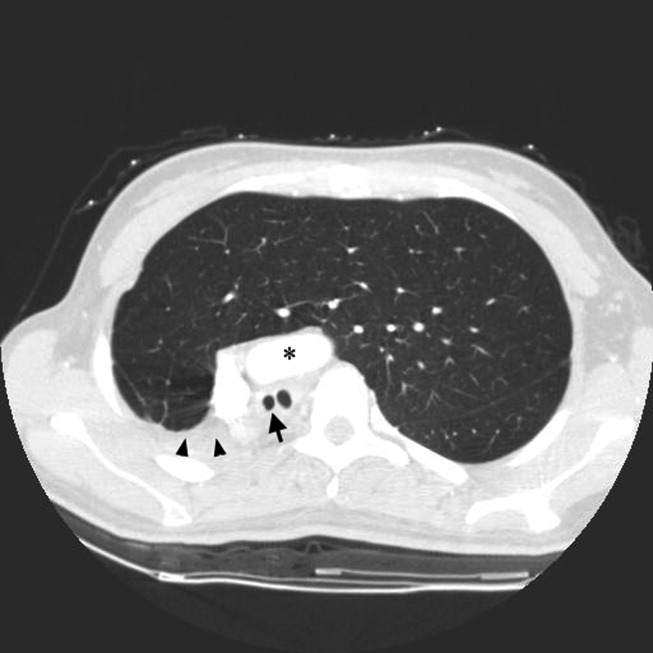
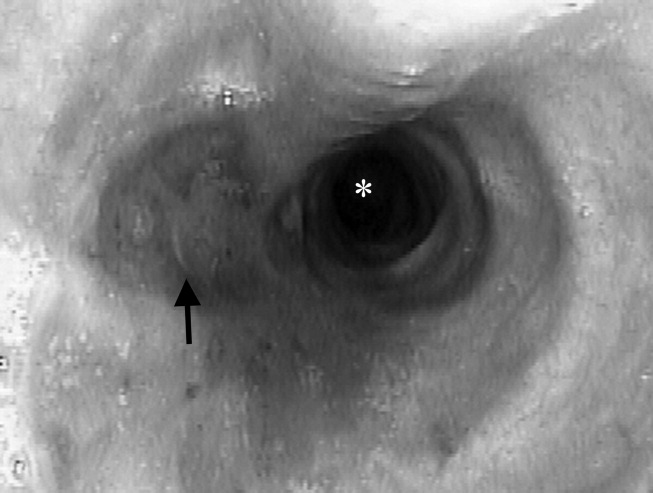
A 23‐year‐old Chinese woman presented with worsening exertional dyspnea. Her medical history was notable for pulmonary tuberculosis treated at the age of 16. Over the past 3 years, she reported progressive respiratory symptoms resulting in marked exercise intolerance. She denied any fevers, cough, or weight loss. On examination, she had right‐sided tracheal deviation but spoke comfortably. Her heart sounds were displaced and right‐sided breath sounds nearly absent. Chest x‐ray (Fig. 1) and subsequent CT revealed complete collapse of the right lung with associated hyperexpansion of the left lung and left‐to‐right mediastinal shift (Fig. 2, with an asterisk denoting the aortic arch; an arrow, the right main‐stem bronchus, which would soon terminate; and arrowheads, the collapsed right lung). No lung masses or effusions were noted; active TB had been ruled out with AFB sputums. Bronchoscopy revealed a fibrotic and stenotic right main‐stem bronchus (Fig. 3, with an asterisk denoting a patent left main‐stem bronchus and an arrow denoting a stenotic right main‐stem bronchus). Pulmonary manifestations of TB include parenchymal and endobronchial disease. Patients more likely to develop endobronchial disease include those with extensive pulmonary involvement, particularly cavitary lesions. Between 10% and 20% of patients with endobronchial disease will have normal CXRs, though CT scans may reveal endobronchial lesions or narrowing. Complications of endobronchial disease include obstruction, bronchiectasis, and tracheal or bronchial stenosis. Some airway obstructions may be associated with enlarging peribronchial nodes, which may erode into the airways as broncholiths. Steroids have been used to prevent long‐term complications, but their efficacy is still unclear. Repeated dilation, stenting, and resection all serve as management options for advanced endobronchial disease. In our patient, the extensive bronchial scarring and stenosis were most likely complications from past endobronchial infection. Unfortunately, attempts at balloon dilatation of her right main‐stem bronchus were unsuccessful, and she continues to have considerable exercise limitation. More prompt recognition of the disease may have allowed for an earlier and more successful intervention.
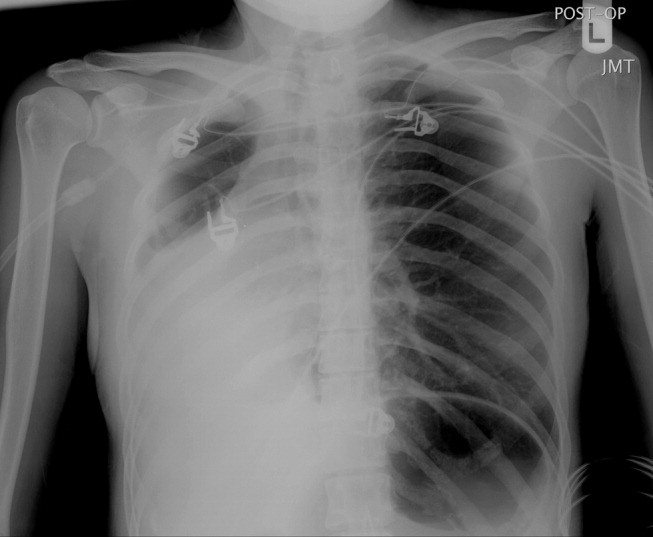
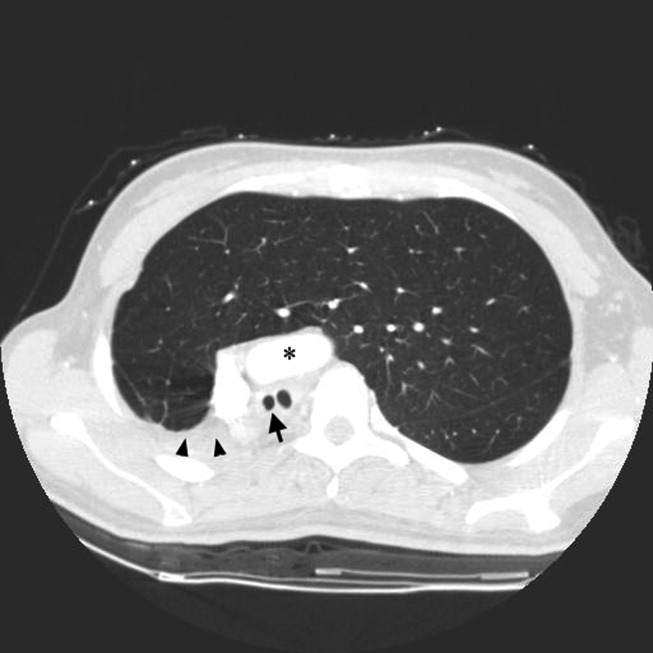
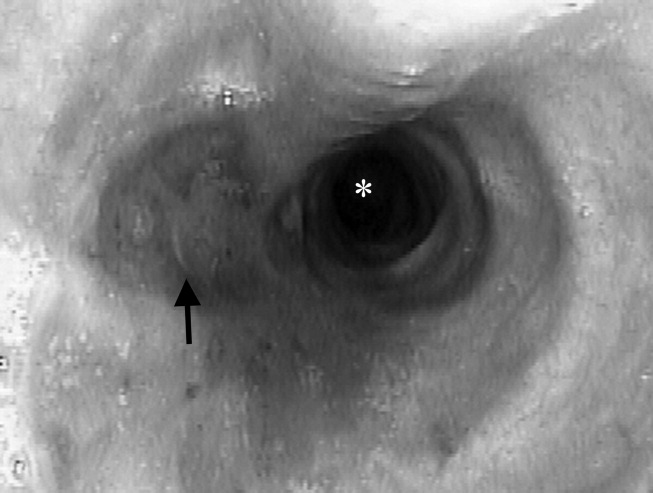
A 23‐year‐old Chinese woman presented with worsening exertional dyspnea. Her medical history was notable for pulmonary tuberculosis treated at the age of 16. Over the past 3 years, she reported progressive respiratory symptoms resulting in marked exercise intolerance. She denied any fevers, cough, or weight loss. On examination, she had right‐sided tracheal deviation but spoke comfortably. Her heart sounds were displaced and right‐sided breath sounds nearly absent. Chest x‐ray (Fig. 1) and subsequent CT revealed complete collapse of the right lung with associated hyperexpansion of the left lung and left‐to‐right mediastinal shift (Fig. 2, with an asterisk denoting the aortic arch; an arrow, the right main‐stem bronchus, which would soon terminate; and arrowheads, the collapsed right lung). No lung masses or effusions were noted; active TB had been ruled out with AFB sputums. Bronchoscopy revealed a fibrotic and stenotic right main‐stem bronchus (Fig. 3, with an asterisk denoting a patent left main‐stem bronchus and an arrow denoting a stenotic right main‐stem bronchus). Pulmonary manifestations of TB include parenchymal and endobronchial disease. Patients more likely to develop endobronchial disease include those with extensive pulmonary involvement, particularly cavitary lesions. Between 10% and 20% of patients with endobronchial disease will have normal CXRs, though CT scans may reveal endobronchial lesions or narrowing. Complications of endobronchial disease include obstruction, bronchiectasis, and tracheal or bronchial stenosis. Some airway obstructions may be associated with enlarging peribronchial nodes, which may erode into the airways as broncholiths. Steroids have been used to prevent long‐term complications, but their efficacy is still unclear. Repeated dilation, stenting, and resection all serve as management options for advanced endobronchial disease. In our patient, the extensive bronchial scarring and stenosis were most likely complications from past endobronchial infection. Unfortunately, attempts at balloon dilatation of her right main‐stem bronchus were unsuccessful, and she continues to have considerable exercise limitation. More prompt recognition of the disease may have allowed for an earlier and more successful intervention.
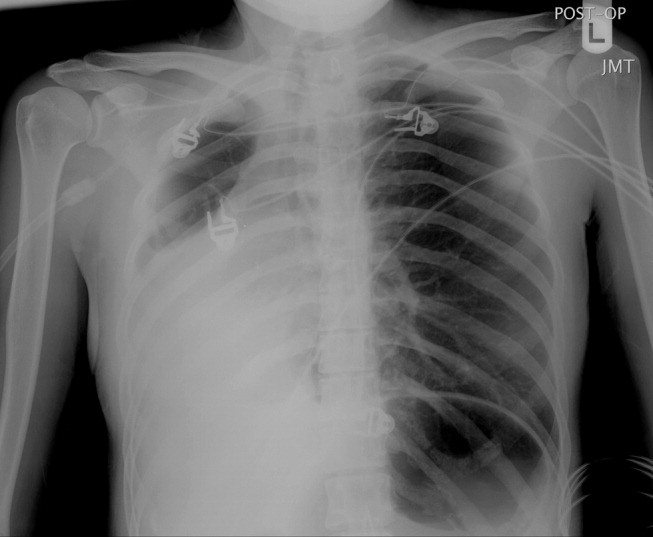
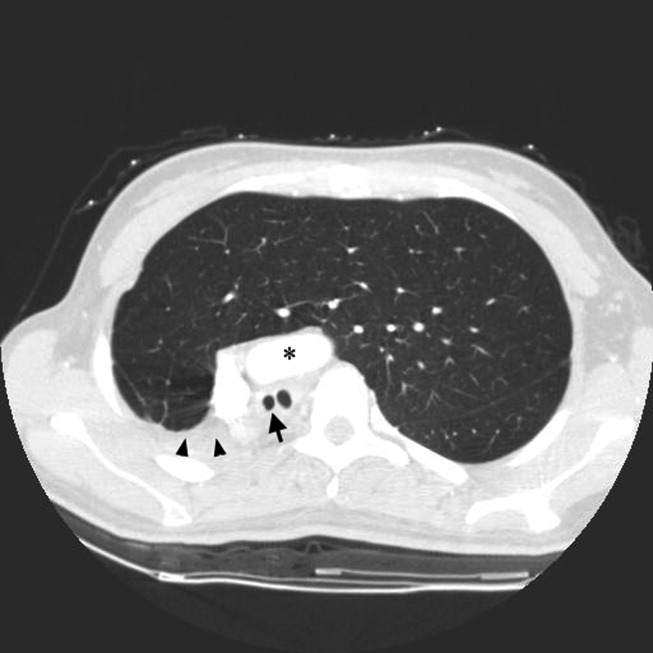
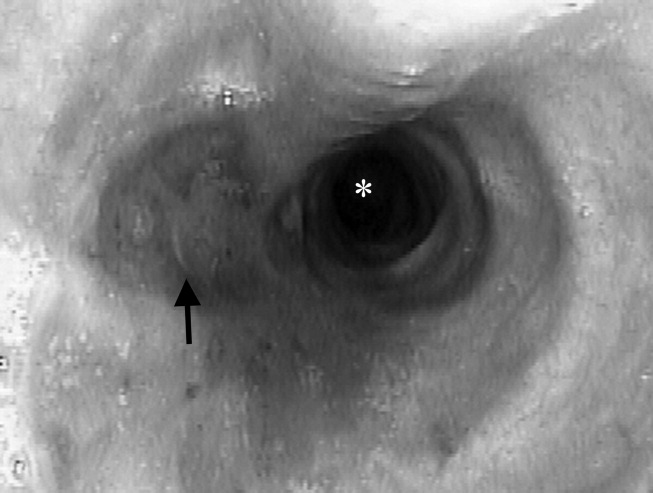